1. Introduction
Although, the organic peroxides are widely used as bleaching agents, antibacterial and polymeric initiators, the current interest on these substances lies in the application of these derivatives as explosive initiators [1]. Particularly, the compounds diacetone diperoxide (DADP) and triacetone triperoxide (TATP), are the most common acetone peroxides for the synthesis of homemade explosives (HME), and for this reason they are of interest for defense and national security applications. Their easy synthesis from the reaction of hydrogen peroxide with acetone catalyzed by acid, together with the high availability of the basic products make them attractive precursors for the preparation of homemade explosive materials [1-6].
Due to the great instability presented by these materials they are not normally used for military purpose, but rather for criminal purposes, therefore their identification and proper detection becomes of great importance [7]. Although there are many studies on the TATP molecule, the DADP molecule has a feature that makes it more attractive for the design of energetic materials; this fact is because the oxygen atoms in the molecule are more accessible than in the TATP, which enables them to be easily functionalized [8].
For many years, the infrared and Raman spectra have been used for the identification and structural elucidation of organic molecules due to its potential to provide vibrational information on what type of atoms are involved in a particular bond, these techniques prevail as one of the most commonly used for the characterization of DADP and others compounds of interest. In most of the studies described, the band assignment is done theoretically, or by isotopic labeling of hydrogen atoms with deuterium atoms. With this type of isotopic labeling is not achieved the strategic labeling of the carbon atoms involved in the different vibrational modes [3,9-12].
This research deals with the assessment of the theoretical assignment of the different bands in the vibrational spectrum of the DADP molecule. This verification was made through partial isotopic labeling of the different carbon atoms in the molecule, thus achieving, the corresponding displacement in the bands involved. Use Time New Roman font with size of 10 points for normal text paragraphs. The first line of each paragraph is indented 0.5 cm.
2. Experimental setup
2.1. Reagents
The reagents used in the synthesis of the DADP isotopomers were: acetone-13C, acetone-13C2, and neat acetone at 99.7%, hydrogen peroxide stabilized at 50%, ultra-pure water, m-sulphonic and toluene-sulphonic acids. Dichloromethane at 99.8% stabilized HPLC grade. All the reagents listed above were purchased in Fisher Scientific and used without further purification.
2.2. Synthesis of DADP
DADP and its isotopomers was prepare using a modified procedure of the reported by Espinosa-Fuentes et al, which consists in the oxidation of acetone with hydrogen peroxide catalyzed by m-sulphonic or toluene-sulphonic acids [6]. The acetone labeled on the carbonyl carbon and the methyl carbons atoms were used for the synthesis of the DADP-C2 and DADP-C4 isotopomers respectively. The white crystals of DADP were washed with deionized water and recrystallized in dichloromethane. Immediately shows a reaction scheme.
2.3. Instrumental analysis
The infrared spectra were recorded in an infrared spectrophotometer with Fourier transformed, Bruker Optics brand, model IFS 66v/S, equipped with a DTGS detector, in the spectral region of 400 to 4000 cm-1, 10 scans per spectrum and a resolution of 4 cm-1 were used. The Raman spectra were recorded using a Raman spectrophotometer, EZ-Raman, equipped with an excitation line of 785 nm, in the spectral region of 400 to 3200 cm-1, 10 accumulations per spectrum and a resolution of 1 cm-1.
3. Results and discussion
To verify the assignment of each band in the vibrational spectra of the DADP molecule, it was taken as a basis the theoretical distribution reported by Coronel et al [10]. For DADP, a nonlinear molecule, the 3N-6 modes correspond to 50 vibrations. Before checking the theoretical assignment of each vibrational band, we will explain the relationship between the atoms in the molecule (see Fig. 1) with the internal coordinates and the distribution of the potential energy of a particular band. For this, was chosen the vibrational mode 44 (ν44), which has the following distribution of potential energy 50S2+44S1, this means that, the internal coordinates S1 and S2 contribute to the vibration energy in a percentage of 50 and 44 respectively. According to supplementary Table 1 and the molecular numbering of Fig. 1, the atoms involved in this vibration are H(14), H(13), H(12) and C-methyl(11) for S1 and S2: H(18), H(16), H(17) and C-methyl(15). For this particular band, is possible to corroborate experimentally its assignment, by labeling the methyl atoms. The isotopic labeling of a carbon atom different to the previous ones should not displace the band corresponding to Mode 44 (Table 1-2 of the supplemental material); if this happens, then the theoretical contribution is wrong.
The theoretical energy distribution of the DADP spectrum presented several differences, regarding the experimental displacements of the isotopomers spectra (DADP-13C4 and DADP-13C2); among others, which agreed with the theoretical assignment. The bands at 3034, 3033, 2958, 3008, 1466, 1477, 1377, 1010, 943, 860, 687, 525, 476 cm-1 showed concordance with potential energy distribution (PED). These spectral bands correspond to the vibrational modes 20, 32, 2, 19, 45, 31, 3, 46, 45, 5, 48, 54, 37, 57, 58, 39 according to reported by Coronel et al [10]. These modes are related only with movements of the methyl group (C and H atoms) (Fig. 2-6).
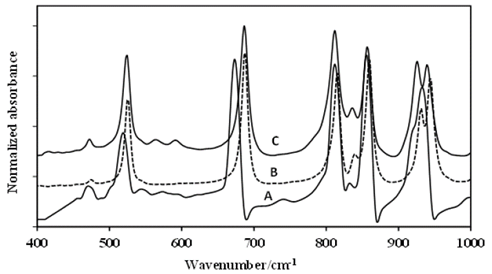
Source: The authors.
Figure 2 First portion of the FT-IR spectra of: A-DADP-C4, B-DADP and C-DADP-C2.
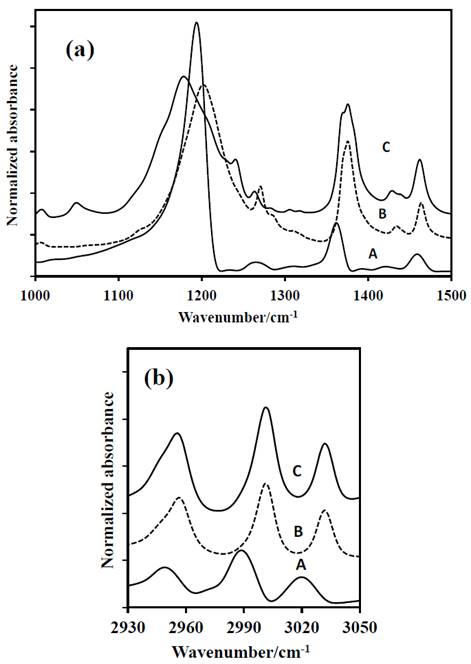
Source: The authors.
Figure 3 FT-IR spectra of: A-DADP-C4, B-DADP and C-DADP-C2. (a) at 1000-1500 cm-1 (b) at 2900-3050 cm-1 spectral range.
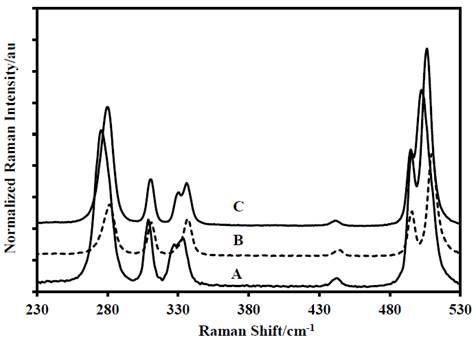
Source: The authors.
Figure 4 First portion of the Raman spectra of: A-DADP-C4, B-DADP and C-DADP-C2.
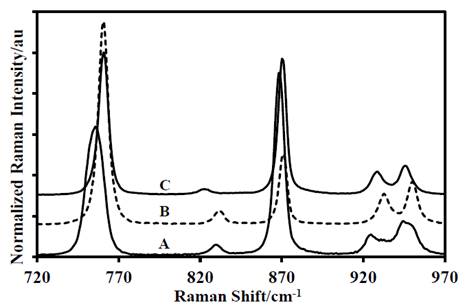
Source: The authors.
Figure 5 Second portion of the Raman spectra of: A-DADP-C4, B-DADP and C-DADP-C2.
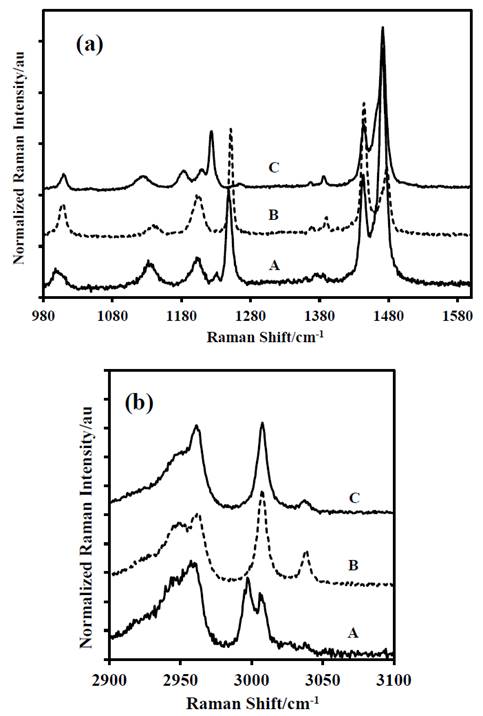
Source: The authors.
Figure 6 Last portion of the Raman spectra of: A-DADP-C4, B-DADP and C-DADP-C2 (a) at 2900-3100 cm-1 spectral range. (b) at 1000.1600 cm-1 spectral range.
In contrast with the bands above, some differences were observed in the others assignments. For example, the band at 1439 cm-1, despite the fact that its theoretical contribution is only for methyl groups, when other atoms were isotopically labeled (specifically annular peroxyl carbons), the band showed a displacement of 7 cm-1 by change in its reduced mass. The above analysis demonstrates that the theoretical assignment is wrong and the peroxyl carbon atoms also contribute to vibrational mode.
The band at 1273 cm-1 (ν52), despite that its theoretical contribution includes methyl group and carbon atoms of the DADP ring, it only presented displacement, when carbon atoms of the ring were labeled isotopically. This result suggests that the methyl groups do not contribute to the mode ν52. The bands at 1205 cm-1, 1141 cm-1 and 951 cm-1 (ν35, ν23 and ν11) showed concordance in the response by mass variation of their correspondent groups. But the largest displacement was observed when the peroxyl carbon atoms of the ring were labeled isotopically, despite the methyl groups have greater contribution to the band.
The following difference was found in the assignment of the band at 931 cm-1 (ν55), which is entirely due to the methyl groups motions, however this mode presented displacement by mass change of the non-methylic atoms. The opposite happened with the bands at 841 cm-1, 833 cm-1, 761 cm-1, 687 cm-1, 525 cm-1, 496 cm-1, 337 cm-1, 312 cm-1 and 280 cm-1 (ν56, ν25, ν14, ν57, ν58, ν26, ν17, ν28 and ν18), which presented displacement only when methyl carbon atoms (DADP-C4) were labeled.
Finally, according to the theoretical PED, the band at 873 cm-1 is due to vibration of the O-O bond, therefore this band should not present any mass-shift in the spectra of the DADP isotopomers, however, the spectrum of both isotopomers showed vibrational displacement, specifically with the methyl and peroxyl carbon atoms. The band at 860 cm-1 also showed dependence with the methyl group, despite that the theoretical distribution does not consider it.
Over the years have been shown that the theoretical methods are a useful tool for understanding certain complex problems. However, in this report was observed that, the theoretical method is fairly efficient for movements of pure groups. For vibrational movements involving various functional groups, the method does not work well. For example, fairly predicts mappings between methyl atoms, nor it predicts vibrations between methyl atoms and peroxyl carbon atoms in the molecule studied.
4. Conclusions
In general, the theoretical method for the assignment of vibrational bands is fairly efficient for pure group’s movements. For vibrational movements involving various functional groups, the method works with some difficulty. For example, barely predicts combined assignment between methyl atoms, but does not predict vibration between methyl atoms and peroxyl carbon atoms in the molecule studied. For peroxyl displacements, which are not totally pure, the theoretical PED was unable to predict its contribution. Finally, it is important that the vibrational assignment is based on strategic isotopic marking and not in theoretical assignments, for the unequivocal acceptance in applied research.