1. Introduction
Brazil is in a large mid-plate region considered one of the least seismically active continental areas in the world. Earthquakes with maximum magnitudes 5 and above occur with a return period of 4 years [1]. This means that recordings of strong ground motions in the region are scarce. As alternative to obtain earthquakes to be applied in the seismic design of structures or site response analyses in such intraplate regions, different methodologies can be applied [32].
This paper aims to obtain a series of accelerograms representative of the earthquake scenarios in a region located in Southeastern Brazil, specifically in the Campos Basin Continental Slope, offshore of the State of Rio de Janeiro. The dominant earthquake scenarios and mean uniform hazard spectra used in this work for the region of interest were developed by [5]. Three methodologies were employed to obtain spectrum-compatible accelerograms. The first approach consisted of the use of real accelerograms obtained from the Pacific Earthquake Engineering Research (PEER-NGA) database [58] and the application of spectral matching procedures. A second methodology of developing synthetic accelerograms generated from seismological source models [45,46]. And a third alternative, also based on synthetic accelerograms, using envelope shapes adjusted from real records of accelerograms in Brazil [3,57].
1.1 Seismic hazard in Southeastern Brazil
Based on the distribution of seismicity, the main active seismic areas in Brazil were delineated by [1] as follows: (1) Southern part of the Guyana shield and middle Amazon basin; (2) a North-South-trending zone along the Eastern border of the Amazon craton; (3) Northern part of the Borborena Province, in Northeastern Brazil around the Potiguar marginal basin; (4) the Porto dos Gauchos seismic zone; (5) a NE-SW zone in the Tocantins province possibly continuing towards the Pantanal Basin; (6) Southern Minas Gerais zone, in and around the southern tip of the São Francisco craton; and (7) the Southeast offshore zone with activity concentrated along the continental slope from the Pelotas Basin in the south to the Campos basin from 33°S to 20°S [2,3]. The Campos Basin represents an important pole in Brazilian economy and is one of the most prolific Brazilian oil-producing basins [4]. Given the importance of the Campos Basin, moderate seismicity in the oceanic portion of the Southeast region (magnitude 5 every 10-30 years and potential occurrences of magnitude up to 7) represent a hazard to the design of offshore infrastructure developments. The area of interest for the analyses presented in this work correspond to the continental margin of Southeastern Brazil. Specifically, the Campos Basin Continental Slope [6], offshore the state of Rio de Janeiro (Fig. 1).
Two main sources of stress are associated in the seismicity pattern in the Brazilian Atlantic coast. A regional component of compressive stresses and a local component due to density contrast between continental/oceanic crusts and lithospheric flexure due to sediment load in the continental shelf [2]. The overall structural style in Campos Basin is detached, with a detachment surface at the base of the Aptian salt [7]. Below the detachment, the main structural features are horsts and grabens limited by steep normal faults [8,9]. The stress regime is variable with depth. Extensional regimes at depths of less than 1,500 m, transtensional regimes at depths ranging from 1,500 to 3,500 m, and strike-slip, for depths greater than 3,500 m [10].
1.2 Earthquake catalog
Brazilian earthquake data from historical and relatively recent instrumental records have been compiled through the earthquake catalog provided by the Seismology Center of the Institute of Astronomy Geophysics and Atmospheric Sciences of the University of São Paulo (IAG/USP). Further details on the earthquake catalog are presented [11]. Fig. 2 presents the location of the epicentres and magnitudes of the historical earthquakes covering a period from November 1720 to December 2019. It can be observed from Fig. 2 a concentration of epicenters in the Campos Basin, roughly across the continental slope [5].
2. Selection of earthquake ground motion accelerograms
For seismic hazard assessment, the definition of the ground motions represents a significant role. The most complete way to represent the ground motion is through seismograms that describe the variation with time of the accelerations, velocity, and displacements. It is usually displayed the variation of the accelerations with time (accelerogram), the velocities and displacements are obtained by integration.
One common challenge of many intraplate regions is the limited amount of significant earthquake records due to the infrequent occurrence of these events. When two of the largest Brazilian earthquakes occurred in 1955 (mb 6.2 on January 31 and mb 6.1 on March 1) no Brazilian stations were operating [11]. Early instrumental data in the Brazilian earthquake catalog were obtained from international agencies (USGS and ISC) and using stations from other countries. Only in the late 1960s with the installation and in the 1970s instrumental records begin in earnest [12]. Further details related to the history of the seismological stations in Brazil can be found in [13]. The most recent boost in the seismological research in Brazil started in 2011 with the operation of the Brazilian Seismographic Network [11]. Given the scarcity of recorded accelerograms, an alternative to obtaining strong motion data consist of scaling and matching ground motions from other regions with local seismic hazard response spectra [14] or the generation of spectrum compatible accelerograms [15-18].
The accelerogram generation methods can be divided into the following categories: (1) deterministic methods, implementing a superposition of harmonic waves to match a target response spectrum [19]; (2) wavelet-based techniques [20-23]; (3) stochastic methods that consider the accelerogram as a Gaussian process [24-26]. In this work, three methodologies will be employed to obtain spectrum compatible ground motions. For the analyses, the Mean Uniform Hazard Response Spectra for the site of interest at the continental slope of the Campos Basin for a return period of 975 years was used as target spectrum (Fig. 3).
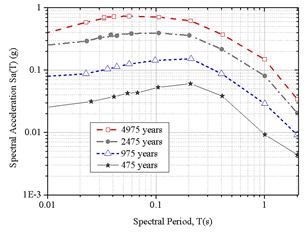
Source: Adapted from [5].
Figure 3 Uniform Hazard Response spectra for the site of interest at the continental slope of the Campos Basin for various return periods.
2.1 Method 1: seismic records from database
The first approach consisted of using recorded accelerograms from the Pacific Earthquake Engineering Research (PEER) Strong Motion Database. The PEER database consists of two databases: the NGA-East Ground Motion Database and the NGA-West Database [58]. The first constitutes the largest database of processed recorded ground motions in Stable Continental Regions (SRCs).
The second includes a very large set of ground motions recorded worldwide of shallow crustal earthquakes in active tectonic regimes. As Brazil is located within a stable continental intraplate region, it was considered at a first instance to use the NGA-East database. However, from the results of a preliminary search, no records satisfied the criteria discussed in the following section. Then, the NGA-West database was used. A similar approach was used for the selection of earthquake ground accelerograms in Hong Kong, also an intraplate area of low to moderate seismicity [14].
2.1.1 Search and selection criteria
The PEER web application allows the user to load a target spectrum and a series of criteria to select the earthquakes (magnitude, fault type, significant duration, distance to rupture plane, average shear wave velocity of top 30 meters’ site, and pulse characteristics). The ground motions can also be adjusted to reduce the Mean Squared Error (MSE) and increase the match between the target and recorded (scaled) spectra. A maximum limit for the scale factor of 2.0 was defined for the records that satisfied the search criteria. This value for the scale factor of 2.0 was proposed by [27].
From a disaggregation analysis, it is possible to obtain the contribution of different earthquake scenarios at the site of interest. The parameters considered are the magnitude intervals and distance ranges [28-30]. The moment magnitude and the source-to-site distance are two variables commonly used in the selection of accelerograms. The most relevant geophysical parameter for the selection of records is earthquake magnitude, as it strongly influences frequency content and duration of the motion [31]. Regarding the source-to-site distance, these effects have not been clearly established. Boomer and Acevedo [32] proposed that, in performing the selection of real records, the search window based on the magnitude should be as narrow as possible, and in terms of the distance, the range can be extended. [31] recommended the selection of records from events within 0.25 units of the target magnitude. [33] proposed select the time series, using records within 0.5 magnitude units of the design earthquake. For the current analyses, a search window in the earthquake’s magnitude of ±0.5 M w was adopted, a similar range was used by [14]. The primary reason was that using a narrower search window yielded less or no results when consulting the records from the PEER-NGA East database.
For the area of interest at the offshore Campos Basin, the most significant contribution comes from magnitudes 4.5 to 5.1 M w at distances between 40 and 100 km. Regarding large earthquakes (magnitudes up to 5.7 M w ) it was defined a contribution at distances up to 200 km [5]. There are few events accurately located in the Brazilian earthquake catalog, and the source-to-site distance estimated was at less than about 15 km. In addition, [2] reported an average distance of 10 km for oceanic source zones. Subsequently, the minimum distance used for the search criteria was reduced from 40 to 10 km. Given the stress regime in the Campos Basin, the failure mechanism considered reverse and normal faults, however, to include larger amplitude motions reverse and reverse/oblique faults were accounted in the search criteria. The target site rock condition of the uppermost 30 m of the earth crust (v s,30 ) was defined for v s,30 values higher than 800 m/s. With those criteria, three earthquake scenarios were defined, and the search parameters are shown in Table 1.
The search results are shown in Table 2, 3, a total of twelve records were found. The response spectra of the scaled ground motions are presented in Fig. 4 including the geometric mean of the results. The process of searching and scaling the accelerograms returned a series of results that on average are compatible with the target response spectrum.
Table 3 Accelerograms selected from PEER NGA-West 2 Ground Motion Database (part 2)
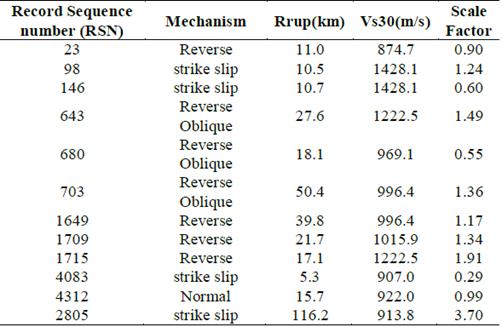
Source: the authors
From Fig. 4, it can be observed that the scaled records either exceed or are equal to the design spectra only over a certain period range. In addition, the second part of the application of the methodology consists of modifying the time series and makes them compatible with the target response spectra at all spectral periods is the spectral matching. The use of spectrum compatible time series for the seismic design of structures has been discussed by [33]. On one hand, spectrum compatible time series represent more than one earthquake and may be considered unrealistic when compared to typical earthquake response spectra. On the other hand, spectrum compatible time series has the advantage to reduce the number of time series necessary for an Engineering analysis [34], and the variability in spectral amplitude is considerably shortened.
Various methodologies have been proposed to obtain spectrum compatible time series [56,35,37-39]. Three basic approaches for spectral matching have been adopted in the different methods [33]: frequency-domain method, frequency-domain method with Random Vibration Theory (RVT), and time-domain method. The third approach is generally more complicated, however, produces a good convergence [40,41]. [42] implemented in RSPMatch program the Lilahanand and Tseng algorithm, a method based on the spectral matching in the time-domain. The adjustment wavelets used, however, produced drifts in the displacement and velocity time series and baseline corrections were required. The method was revised by [43] using new wavelets, to eliminate the drift in the adjustment wavelets to produce time series that do not require baseline correction.
The methodology applied in the current work and implemented in the software SeismoMatch [22] used the wavelets algorithm proposed by [33]. It has the advantage of matching the pseudo-acceleration response spectra of the time series with the target spectra, it does not introduce additional energy into the ground motion, preserves the characteristics of the original ground motion and eliminates the drift in the velocity and displacement time series.
As an example of the matching process in terms of the target response spectra, Fourier spectra, and Arias intensity, two earthquakes from Table 2 were selected. Arias Intensity (energy content) was included in the comparison of the matched records, considering its importance in seismic hazard analysis [44]. Fig. 5 shows the acceleration time-histories, Fourier spectra and Arias Intensities of the recorded and matched time series of earthquakes RSN 23 and RSN 4803. The Fourier amplitude maintains the characteristics of the initial time series. In addition, a good agreement was observed between the normalized Arias intensities of the initial (measured) and the modified (spectrally matched) time series. The same procedure was employed for all the records shown in Tables 2, 3 and the spectral matching results are presented in Fig. 6.
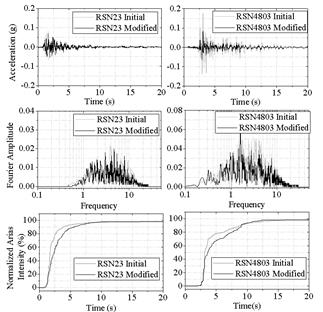
Source: the authors
Figure 5 Example of matched accelerograms: acceleration time-histories, Fourier amplitude and normalized Arias intensity.
From this methodology, it can be stated that nowadays it is relatively straightforward to have access to extensive databases of real strong-motion records. And the criteria for selecting the records are available in the literature [32] to obtain results representative of the seismicity of the area of interest. The spectrum compatible accelerograms obtained presented a satisfactory agreement also in terms of the frequency content and energy when compared to the original records.
2.2 Method 2: synthetic accelerograms
The second alternative consisted of the generation of artificial accelerograms based on the adaptation of a random process to a target spectrum. One of the most complete methodologies for obtaining ground motions is the specific barrier model developed by [45,46]. The model has been implemented and calibrated with extended databases of response spectral amplitudes from earthquakes of intraplate areas, interplate regions and regions of tectonic extension [47]. The advantage of this model is the use of relatively few parameters to generate records: (1) tectonic regime, (2) magnitude, (3) distance, and (4) soil/rock type. The method has been implemented in the software SeismoArtif [48], in which the generation of the synthetic accelerogram starts from a Gaussian white noise that is multiplied by the [49] envelope shape and then adapted to a target spectrum using the Fourier Transform Method [50].
Fig. 7 presents a comparison between the Peak Ground Accelerations (PGA) obtained from the synthetic accelerograms versus distance and the curve obtained from the Ground Motion Prediction Equation (GMPE) developed by [51] and modified by [52], for the stable continental region in Central and Eastern North America (curve for a scaling factor equal to 1.00 in Fig. 7). Considering that Brazil is in an intraplate setting of low attenuation, [5] adopted [51] and [52] GMPE for the probabilistic hazard assessment in the continental margin of Southeastern Brazil. Additional curves were generated using Scaling Factors (SF) to cover the range of possible seismic attenuation in Brazil (SF = 0.4, 0.75, 1.00, 1.33). From Fig. 7, it can be observed a good agreement in terms of the PGA values obtained from the synthetic accelerograms and the curves obtained from GMPE.
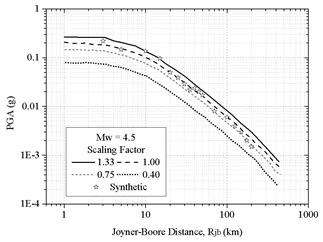
Source: the authors.
Figure 7 Peak Ground Accelerations with distance: comparison between GMPE and synthetic accelerograms for a 4.5 M w earthquake.
A series of artificial accelerograms were obtained using the criteria of the disaggregation analysis for earthquake scenarios as presented in Tables 1 and 4 presents in detail the criteria adopted for the generation of the synthetic accelerograms. Figs. 8 and 9 show examples of synthetic acceleration time-histories for two earthquakes of magnitudes 4.5 and 5.5 M w , for a rupture distance of 10 km.
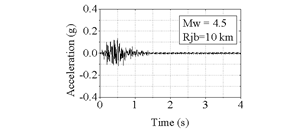
Source: the authors.
Figure 8 Example of a synthetic accelerogram using the specific barrier model (Mw=4.5).
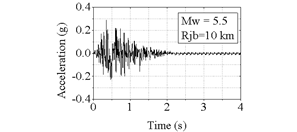
Source: the authors.
Figure 9 Example of a synthetic accelerograms using the specific barrier model (Mw=5.5).
Once obtained the synthetic accelerograms, the following stage consisted of performing a spectral matching based on the target spectra. The results are presented in Fig. 10. The algorithm was able to converge for all the synthetic accelerograms with slight variations for periods larger than one second.
2.3 Method 3: synthetic accelerograms using shape functions
A third approach to obtain synthetic accelerograms consisted of modifying a starting random process using an envelope shape [53-55,49] and a Power Spectral Density Function (PDSF). The method was proposed by [56], and it starts with the generation of steady-state motions (sinusoidal waves) with phase angles in the interval (0, 2π) and amplitudes given by the PSDF (eq. 1).
Where q(t) is an envelope shape (or intensity function) that allows to resemble a real accelerogram; 𝐴𝑖, 𝜔𝑖 and 𝛷𝑖 are respectively the amplitude, frequency, and the phase angle if the i th sinusoidal wave. The generated ground motion is adapted to the target response spectrum using the Fourier Transform Method.
For the current analysis, it was adjusted an exponential envelope shape [54] defined by three parameters: duration, constants that modify the shape of the envelope (α and β) and a parameter (A) that is function of the and coefficients. Fig. 11 shows a representation of the envelope shape and the parameters involved in the calculation.
Given the lack of earthquake records in the continental margin of Southeastern Brazil by Ocean Bottom Seismometers (OBS’s), two earthquakes of particular interest were selected to obtain measured envelope shapes. The first earthquake selected occurred on May 19th, 2012, in the town of Montes Claros, Brazil, a region in which seismicity is not a recent occurrence [57]. The second earthquake occurred on April 23rd, 2008, 125 km South of the city of São Vicente, on the coast of São Paulo State. Both earthquakes were recorded by many stations of the Brazilian seismic network and well-detailed information about the mentioned seismic events was presented by [3] and [57]. Tables 5 and 6 show information about the selected events and the location of the stations that registered the accelerograms used for the analysis.
Figs. 12 and 13 present the acceleration time-histories and the associated envelope shapes of the recorded motions. It can be observed that low accelerations were recorded from the São Vicente earthquake, this is due to the distance between the station and the epicenter (around 313.8 km). The Montes Claros earthquake, on the other hand, was recorded by a station located at 1.44 km of the epicenter, then, relatively higher accelerations can be observed.
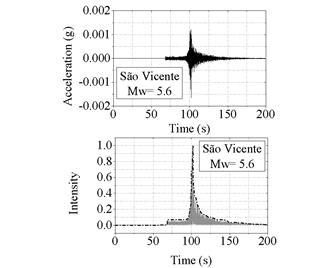
Source: the authors.
Figure 12 Recorded earthquakes and envelope shapes: São Vicente (2008) earthquake.
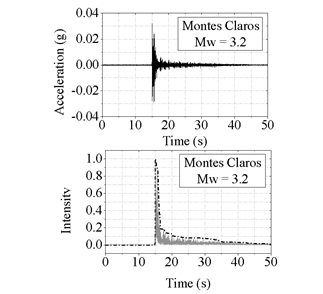
Source: the authors.
Figure 13 Recorded earthquakes and envelope shapes: Montes Claros (2012) earthquake.
Using the envelope shapes of the two recorded accelerograms, the coefficients α and β were defined to match an approximate envelope shape for the generation of the synthetic accelerogram (Fig. 14). The synthetic accelerogram and the response spectra are shown in Fig. 15.
3. Conclusions
This study presented three approaches to acquire spectrum-compatible accelerograms aimed to be applied for site response analyses or seismic design of structures in an area located in the Southeast region Brazil, specifically in the offshore Campos Basin. The first method presented, based on real accelerograms enables the obtention of accelerograms that are spectrum and energy-compatible accelerograms when compared with the historical records obtained from the PEER-NGA database. The second approach, based on seismological characteristics of the zone of interest, produced a good agreement in terms of Peak Ground Accelerations with distance obtained from the GMPE adopted for a stable continental region in Central and Eastern North America and adapted for the continental margin of Southeastern Brazil. The third alternative presented used information from real records in Southeastern Brazil, and well documented in the literature [3,57], to generate a synthetic accelerogram utilizing an adjusted shape function to capture the characteristics of real ground motions.
Further analyses can be performed in the future with more data from earthquakes that occurred in Brazil: to refine the parameters for the generation of synthetic accelerograms or to adjust real records compatible also with the energy and frequency content.