1. Introduction
The market for aromas currently plays an important role in the world economy. In the year 2016, it reached 26.5 billion, with an annual growth rate of approximately 4.4%[1]. In the year 2010 alone, in the USA, the demand for aroma compounds was 75 tons [2]. Aroma and flavour compounds are added to foods for the purpose of conferring, defining, enhancing or reconstituting flavour. They are considered food additives because they have no nutritional function; these compounds can be classified as natural, artificial or identical to natural.
Artificial or natural-like flavours are chemically synthesized, for example, vanillin is generally synthesized from catechol, which is initially methylated to generate guaiacol, which is subsequently condensed to generate the vanillin [3,4]. However, some of the compounds employed for the synthesis of flavourings such as benzene, toluene, and xylene come from petroleum and have been listed as toxic [5]. So, with the increased industrialization of food, demand for more practical and healthy products and depletion of the oil supply, many studies have been carried out to develop processes to produce natural aromas from renewable sources [5,6]. According to Lee and Wendisch [5], Rodriguez et al. [7], aroma compounds in plants, bacteria and fungi are generally derived from the shikimate route. Therefore, the knowledge about this route is considered the key to understanding the metabolism and biological production of the compounds mentioned.
To this end, Zhu et al. [8] produced 15 types of aromatic compounds, including phenylacetic acid, 4-hydroxy-benzoic acid and vanillic acid, using Bacillus ligniniphilus L1 and alkaline lignins as the carbon source. Bruckner et al. [9] obtained a concentration of 1.2 g/L of muconic acid using Saccharomyces cerevisiae. Bluemke and Schrader [10] produced ethyl acetate, propyl acetate, isobutyl acetate, isoamyl acetate, citronellol and geraniol using Ceratocystis moniliformis.
The encapsulation of aromas is a technology of great interest in different areas, especially in food, since preserving the aromas of the products themselves is one of the major concerns of manufacturers. Encapsulation describes different processes used to cover an active compound with a protective wall material. It can be used to treat flavours or convey some degree of protection concerning evaporation, reaction or even migration in food Madene et al. [11]. In general, a microencapsulation process, whereby the active agent is trapped by a coating of polymers with the release of these active agents occurring under specific conditions, is used to protect the material from the adverse conditions of the medium, promoting product stability and increased shelf life.
Encapsulation methods can be divided into two groups: (i) mechanical, such as spray drying, freezing/cooling, extrusion and (ii) chemical, such as coacervation, co-crystallization, ionic gelation, interfacial polymerization and molecular inclusion, ionic gelation, polymeric incompatibility and entrapment in liposomes [11,12]. However, one of the most widely used encapsulation methods is spray drying [11-14].
The spray drying method has been used for decades to encapsulate ingredients used in foods such as aroma compounds (oregano, citronella, cardamom oil etc.), lipids, linoleic acid, essential oils, anthocyanin pigments etc. [11,13,15]. The main advantages of this method are that it provides a high encapsulation efficiency of approximately 96%-100%, as well as being an inexpensive method when compared with other processes [16]. However, this requires studies of encapsulating agents as well as optimization of operating conditions [17].
The encapsulation process through spray drying is generally carried out in three stages: emulsion preparation, homogenization and atomization. The principle of the process is to atomize the material while it is in the liquid state, and when the small drops come into contact with the hot gas, the solvent evaporates, forming a thin layer of the coating material [17]. In this method, the compound to be encapsulated is covered by a matrix generally made of starch, modified starches, some gums, maltodextrins and carboxymethylcellulose, among others [18]. Because of its relatively low cost, starch is one of the most widely used encapsulating agents. The objective of this work was to microencapsulate aromas produced by the yeast Pichia fermentans in sugarcane molasses and apply them to two iced beverages.
2. Methodology
2.1 Microorganism and inoculum preparation
The aroma-producing yeast strain used in this study, P. fermentans ITD 00165, was provided by Microbial Biotechnology Lab's Culture Collection at Durango Institute of Technology (Mexico). For inoculum preparation, the cells were transferred with a wire loop to an Erlenmeyer flask containing 50 mL of YM broth (Himedia). The flask was incubated at 30 °C and 120rpm for 24 h.
2.2 Aroma production
Fermentation occurred in a 4.5 L bioreactor filled with 1.5 L of the medium as described by Rossi et al. [19]. Sugarcane molasses was used as the carbon source in fermentations. The culture medium was prepared with 50 g/L molasses, 1 g/L leucine and 5 g/L magnesium sulphate (MgSSO4-7H2O). Leucine and MgSO4 were added to the medium to improve isoamyl acetate production. After cooling, the bioreactor was inoculated with log 6 cells/ml of aroma-producing yeast and incubated under the following conditions: 30°C and 120 rpm. The profile of volatile compound production was monitored by gas chromatography (GC) over 28 h of fermentation. The fermented broth was concentrated by using a pervaporation module.
2.3 Pervaporation process
The process of recovery and purification of the aromas in the fermented product occurred by pervaporation. The pervaporation process used was previously described according to [19]. The module consisted of a thermostatic bath (Quimis), diaphragm pump (Bomax, Brazil), 4 L feed tank, permeation cell, support for the 1.5 L 'cold trap' (Corning Pyrex) containing liquid nitrogen, a vacuum pump (Boc Edwards, model RV12) and a digital vacuum pressure gage (Instrutherm VDR-920 model). A commercial polymeric organophilic membrane with a PDMS separation flm, PERVAP™ 4060 (Sulzer Chemtech AG, Allschwil, Switzerland) was placed in the permeation cell.
The membrane has a diameter of 8cm, and its effective area was 50 cm2. The permeation cell consisted of a metallic mesh as a solid support to provide tangential flow to the membrane surface. In the process, two different fractions of pervaporate were obtained. Fraction one consisted of the early pervaporate obtained in the first 6 hours. Fraction two consisted of the late pervaporate obtained from 7 hours to the end of the pervaporation process (13 hours).
2.4 SPME/headspace gas chromatography (HS-GC)
The volatiles produced (samples of fermentation broth and fraction recovered by pervaporation) were quantified by gas chromatography (GC) from the analysis of the headspace by solid-phase microextraction (SPME) as already performed by Rossi et al. [19]. The fibre used was carboxen/polydimethylsiloxane of thickness 75 μm (SPME CAR/PDMS), coupled to a manual holder (Supelco, Bellefonte, PA, USA). A 5-mL sample was placed in a 20-mL vial. The septum vials were kept at 30°C under stirring with a magnetic bar. The microfiber was exposed to the sample headspace for 30 min. Desorption was carried out by manual injection into a gas chromatograph equipped with a Shimadzu GC17A, DB-5 column (30mx0.32mm), and a flame ionization detector. The fibre was thermally desorbed by direct exposure to the injector port for 2 min. The injector and detector temperatures were maintained at 250°C. The oven temperature was initially programmed to 40 °C for 5 min and increased at a rate of 20 °C/min to a final temperature of 150 °C, which was maintained for 4 min. Nitrogen was used as the carrier gas, at a flow rate of 1.5mL/min. The split ratio was 1:5. The concentration of volatiles was calculated from a standard curve of isoamyl acetate. Compounds were identified by chromatography by comparing the retention time of the compounds present in the headspace with the retention times of pure standards (Sigma Aldrich) analysed under the same conditions.
2.5 Analysis of aroma compounds by GC-MS
In order to confirm their identification, aroma compounds present in the feed and in the permeate were also analysed in a GC Varian 450 coupled to a Varian 320-MS TQ mass detector with an HP-1MS column (30mx0.25mm) and 1um film (Agilent J & W). The run time was set to 21 min with manual injection of 400μL of headspace using a gastight syringe.
The initial oven temperature was set to 40 °C and raised by 10°C/min to 200 °C. The injector temperature was 250 °C, and the split ratio was 1:2. The mass spectrometer ionization chamber was operated at 250 °C and the transfer line at 280°C. The scan range was 10-300 m/z. Identification was accomplished by comparison with the NIST MS Library 98 (VARIAN) in addition to specific literature [20].
2.6 Microencapsulation
The encapsulating agents tested were dextrin (modified starch 'snowflake 6131' provided by Corn Products Brasil), maltodextrin Mor Rex 1920 (Corn Products Brasil) and pure gum Arabic (Synth, Brasil). The drying and microencapsulation of the aroma were carried out in a spray dryer Labplant model ST05. The drying conditions were air inlet temperature of 150 °C, air flow 57 m3/h and feed rate 390 mL/h. The atomizer nozzle used had a diameter of 0.5 mm.
The formulations initially proposed to obtain the powdered aroma can be found in Table 1. Formulations with ethyl acetate standard (>99.5%, Vetec) were tested In order to define the most suitable encapsulating agents. The formulations were prepared with the addition 15 mL of ethyl acetate in 100 mL of the emulsion prepared with addition of the encapsulating agent in water under magnetic stirring. Following identification of the best encapsulating agent, tests were performed with natural flavour (fermented and concentrated/permeated aroma) in an emulsion with the encapsulating agent according to Table 1. The samples were resuspended after drying (0.1 g of the dry powder in 5 mL of 1% NaCl solution) and analysed by GC. The natural aroma produced by fermentation and concentrated by pervaporation was microencapsulated after selection of the best encapsulating agent, according to the encapsulation efficiency and characteristics of the microcapsules (size and formation of grooves).
Table 1 Formulations of aroma (ethyl acetate) and natural aroma compounds with different encapsulating agents

* Natural concentrated aroma after pervaporation.
Source: The authors.
The encapsulation efficiency (EE), the concentration of aroma present in the microcapsules compared with the concentration of those initially used for drying, was calculated (Eq. 1) according to Rocha et al. [21]as follows:
2.7 Scanning electron microscopy
The encapsulated aromas were analysed by scanning electron microscopy to verify the size and surface of the microcapsules in order to relate these results to the volatile retention capacity and to evaluate the efficiency of the spray drying process. The microencapsulated aromas were fixed on a stub support with double carbon faceted coated with gold under an argon atmosphere in an SCD 30 Balzers metallizer. Afterwards, they were analysed by using a Tescan Vega 3 LMU micro-scanning microscope. The microcapsules were checked for uniformity of size and the formation of grooves on their surfaces.
2.8 Application of the natural aroma in beverages
The natural aroma obtained was applied to sugar-based soda and in a cocktail ('Ice' type mixed sparkling drink), both developed by Duas Rodas Industrial Ltda (Jaragua do Sul, SC). In both products the dosage was 1.0%.
2.9 Sensorial profile
These samples were kept frozen until application of the product in the drinks. Sensory testing was carried out by using a panel of seven trained evaluators. The sensory profiles evaluated were appearance, odour and taste. Analyses began by looking at the appearance of the products, then noticing the odour and finally the taste. Each rater received approximately 25 mL of each sample at 25 °C ± 2 °C in 50 mL clear plastic cups identified with a combination of three random numbers. The sensorial profile was established by discussion and consensus of the group of trained evaluators.
3. Results and discussion
3.1 Volatile profile of the natural aroma
The volatile compound found in the highest concentration in natural aroma by HS-SPME/GC analysis was isoamyl acetate (banana fruity odour) in both samples 1 and 2 (20.02 ppm and 11.89 ppm), comprising 66.66% and 49.54% of the total composition, respectively.
Sample 1 contained significant concentrations of propyl acetate (ethereal fruity odour of banana, 1.475 ppm) and isoamyl alcohol (1.315 ppm), while aroma sample 2 presented 2.791 ppm isoamyl alcohol (Table 2). The compound 2-heptanone, which has a sweet, fruity coconut odour, was found in both samples and showed higher concentrations in aroma sample 2 (1.33 ppm).
Table 2 Volatile profile of the natural aroma analysed by HS-SPME-GC
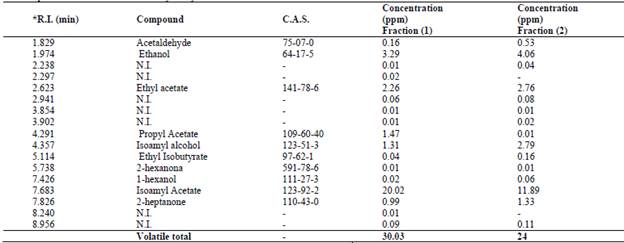
R.I: retention index; NI: Not identified.
Source: The authors
This result is important, given that isoamyl acetate and 2-heptanone are products with high added value and demand in the food, pharmaceutical and perfume industries [22,23]. Isoamyl acetate and 2-heptanone have been widely used in food processing, mainly in the manufacture of jams, fruit punches, honey, caramels, artificial coffee, etc. [24].Other works carried out on P. fermentans also reported on the production of the aroma compounds isoamyl acetate and 2-heptanone [25-27].
On the other hand, other researchers have used enzymes such as lipases for the production of natural isoamyl acetate; however, high-purity reagents are required (ethyl acetate >99 %, isoamyl alcohol >99 %, acetic anhydride >99 %) [1,24]. The fact that isoamyl acetate can be obtained in a natural way and at low cost from a renewable substrate is an advantage for its commercialization because, at present, the trend is toward the consumption of naturally synthesised products [1,24]. According to Zare et al. [24], Yuso et al. [28], isoamyl acetate is traditionally produced by chemical synthesis whereby additional steps are required to separate the solvents from the catalysts employed. Furthermore, chemical syntheses in general have a negative impact on the environment, with low yields and high production costs [2].
Isoamyl acetate and isoamyl alcohol were identified in both fractions at significant concentrations. The latter compound has also been reported by Kong et al. [29], who used Pichia fermentans and Saccharomyces cerevisiae to change the aroma of wine. Table 2 shows the presence of compounds such as acetaldehyde, 2-heptanone, propyl acetate etc. and others that were not identified. This indicates that in the fermentative process, different metabolic routes predominate. Some aroma compounds were detected and identified by GC/MS and did not appear in the CG/FID analysis.
On the other hand, the encapsulated aroma contained fewer components than the concentrated form, which may have been due to the degradation of components such as acetaldehyde, ethanol and 1-propanol, among others (Table 3) by the process of spray drying. According to Favarotrindade eta al. [16], the efficiency of the process of encapsulation by spray drying exceeds 90%, that is, some compounds undergo degradation; however, it is still one of the most efficient encapsulation methods. Aroma compounds concentrated by pervaporation have a higher content of isoamyl alcohol than isoamyl acetate, according to quantification by GC/FID.
3.2 Sensorial Profile of the natural aroma
Sensorial analysis characterized the sugar-based soda containing the natural aroma (fraction 1) as an intense, yellow, turbid liquid. It was described as having an odour with fermented and cooked fruit notes, referring to the banana. It also had soft, fleshy and woody notes reminiscent of sugarcane juice. The taste was sweet, with alcoholic and fermented fleshy notes of fruit. It presented a mild residual taste that was both bitter and phenolic.
The flavoured sugar-based soda (fraction 2) also appeared as a bright yellow turbid liquid. It had an odour with soft, alcoholic notes of fermented and cooked fruit, fused with sweet notes. The taste was alcoholic, sweet and fruity, with fermented features resembling those of cider.
The cocktail/mixed sparkling flavoured drink (fraction 1) was a yellow, turbid liquid with an alcoholic odour and fermented, fruity and oxidized features. It had an acholic, acidic and mildly bitter taste. It presented soft fermented and citrus notes, resembling albedo. The cocktail/mixed sparkling flavoured drink (fraction 2) was described as a yellow, turbid liquid with an intense odour. It had fermented notes with citric features. The flavour was alcoholic, with an intense, bitter and mildly acidic taste. It had a mild, oxidized residual taste. This can be explained by the fact that the aroma compounds were not pure but present in a mixture (Table 2). Thus it was observed in all organoleptic analyses that the fruity notes of banana odour were always more intense, since isoamyl acetate, responsible for banana odour [24], was present in a higher concentration. In addition, the fermented product contained significant amounts of acetaldehyde and 2heptanone, which also give off a fruity odour [30], and ethyl acetate. According to [31,32], the main constituents of banana flavouring are ethyl acetate, ethanol, propyl acetate, isobutyl acetate, ethyl butyrate, isobutyl butyrate, 3-methyl butyl acetate (isoamyl acetate or isoamyl acetate), 2-pentanol, 3- methyl-1-butanol (isoamyl alcohol), 2-methylpropyl butanoate etc. On the other hand, it was observed that the panellists also noticed some alcoholic notes. This could be attributed to the presence of compounds such as ethanol and 1-hexanol.
The aromas presented variations in the sensorial profile according to the application. In soda, fruit notes were described, which referred to banana and fusible notes characteristic of sugarcane liquor. In the cocktail application, citrus features were detected, which were reminiscent of albedo and cider. The sensorial profile detected the presence of fermented notes and residual flavour, suggesting the need to improve these aspects for application in cocktails or mixed sparkling drinks. Aroma (fraction 2) was the product that presented the highest acceptability when applied to beverages by a trained aroma panellist. In order to preserve the original characteristics of the aroma and avoid oxidation, the product was dried and microencapsulated in a spray dryer. The efficiency of microencapsulation of the compounds (ethyl acetate and natural flavour) was determined according to the concentration of the volatile components measured by SPME-CG. The concentrations of powdered compounds (ethyl acetate and natural flavour) in the different formulations were compared in the spray drier feed. The results can be seen in Fig. 1. According to the highest final concentration in the resuspended powder, the best encapsulating agents were dextrin (modified starch, 73.49 ppm) and the mixture of dextrin and maltodextrin (72.58 ppm), which were formulations 1 and 4, respectively.
From the results obtained in tests using ethyl acetate, the encapsulating agents dextrin and maltodextrin were the most efficient at retaining aroma compounds. These agents were used to encapsulate the natural aroma obtained by fermentation. In microencapsulation, the aroma produced by fermentation without any downstream process was called 'fermented', and the aroma recovered and concentrated by pervaporation was called 'permeated'.
The concentration and powder yield of isoamyl acetate were 9.86 ppm and 18.56%, respectively, for formulation 5 (dextrin) and 7.90 ppm and 26%, respectively, for formulation 6 (dextrin and maltodextrin). In relation to the concentration of isoamyl acetate in the powder, the best encapsulating agent for the permeate (concentrated natural flavour) was dextrin. The formulation fermented with dextrin presented the lowest concentration of isoamyl acetate, 3.2 ppm (Fig. 1). The best encapsulating agents according to their efficiency (%) were dextrin (52.46%), maltodextrin with dextrin (42.46%), gum Arabic (25.92%) and maltodextrin (19.68%) (Table 4). Among the samples with concentrated natural flavour, the highest efficiency was for dextrin, with 68.95%, followed by the mixture of maltodextrin and dextrin encapsulating agents, whose efficiency was 39.33%.
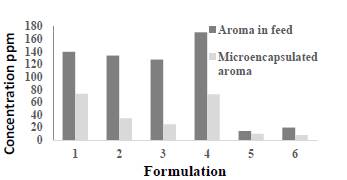
Source: The authors.
Figure 1 Concentrations of aroma compounds (ethyl acetate and isoamyl acetate) according to the formulations studied for drying. 1) ethyl acetate/dextrin, 2) ethyl acetate/gum Arabic, 3) ethyl acetate/maltodextrin, 4) ethyl acetate/dextrin and maltodextrin, 5) natural flavour/dextrin and 6) natural flavour/dextrin and maltodextrin.
Previous work has shown that gum Arabic, dextrins, maltodextrins etc. or their mixtures are good encapsulating agents [33,34]. Aburto et al. [35] identified modified starch (dextrin), with volatile retention greater than 93%, gum Arabic (85%) and maltodextrin (80%) as the best encapsulating agents for orange essential oil. Balasubramani et al. [36] reported a maximum encapsulation efficiency of 82% for a garlic oleoresin using 60% maltodextrin. In addition, Ascheri et al. [37] microencapsulated orange essential oil and obtained the best result with 36% maltodextrin and 10% modified starch (capsule), in which the retention efficiencies of aromatic compounds were 83%, 84% and 99% for α-pinene, β-pinene and limonene, respectively.
In this research, the mixture of 15% modified starch and 15% maltodextrin resulted in an encapsulation efficiency of 33.9%. For the natural aroma (permeated samples), the best encapsulating agent was snowflake dextrin modified starch, with a retention rate of 68.95% for isoamyl acetate.
According to the results shown in Table 4, maltodextrin was the encapsulating agent with the lowest encapsulation efficiency (19.68%). This probably occurred because the concentration tested (30%) was 2 times lower than the one used by Balasubramani et al. [36], and the air inlet temperature (150 °C) was also lower than the temperature used this work (200 °C).
In the abovementioned studies, results varied regarding the encapsulation efficiency of the agents tested. This was most likely due to other parameters that influence on this method of drying. Such parameters are the different characteristics and quantities of the aroma compounds added to emulsion, ratio of the nucleus to the wall material and inlet and outlet air temperature in the spray dryer during drying Janiszewska et al. [38].
3.3 Scanning electron microscopy
Microcapsules of natural aroma usually have particles with spherical, smooth and flat characteristics. These are desirable characteristics because they allow greater flavour retention (less surface area and relative proportion/volume ratio), higher mass densities (better powder packaging) and better flow to be reached. As seen in Fig. 2, it is generally observed that the microcapsules have irregular surfaces with folds. According to Ascheri et al. [37], this probably happens due to the internal development of vacuoles, tooth depressions and external cracks. Furthermore, surface imperfections occur when there is a slow formation of crust on the surface of the particles as the pulverized droplets dry. The presence of 'teeth' has an adverse effect on the flow properties of the product.
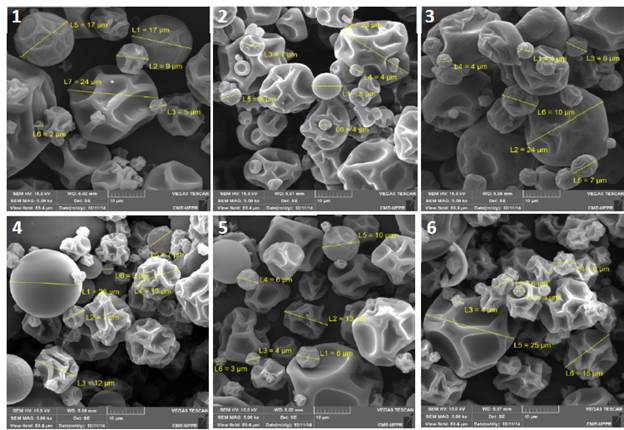
Source: The authors.
Figure 2 Natural-flavoured ethyl acetate microcapsules obtained by fermentation using different encapsulating agents. 1) ethyl acetate/dextrin, 2) ethyl acetate/gum Arabic, 3) ethyl acetate/maltodextrin, 4) ethyl acetate/dextrin and maltodextrin, 5) natural flavour/dextrin, 6) natural flavour/dextrin and maltodextrin.
On the other hand, flat spheres were also observed in Figs. 2-2, 2-4 and 2-5. According to Osorio et al. [39], flat spheres are desirable for the encapsulated ingredients stability and controlled release. Balasubramani et al. [36] obtained good retention of active compounds of garlic (81.9%).
Table 4 Characteristics of ethyl acetate spray drier emulsion, microcapsules and natural aroma
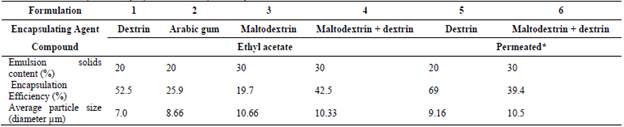
* Permeated: pervaporation concentrated natural liquid aroma
Source: The authors.
The compounds were encapsulated with maltodextrin, and microcapsules presented spherical structures with smooth surfaces and no grooves when analysed by scanning electron microscopy. The only samples which were composed by a few spherical and smooth particles were formulation 2(ethyl acetate/ gum Arabic), 4 (a sample of ethyl acetate with 15% dextrin and 15% maltodextrin) and 5 (natural aroma /dextrin). In these formulations, there was retention of volatile compounds of 25.92%, 42.46% and 69%, respectively. According to the figures below, all samples had particles ranging from 2 to 25 μm and grooves on the surface.
3.4 Microencapsulated flavour stability
After 3 months of storage, the samples were resuspended and analysed in GC by HS-SPME. All the samples were stable (Table 5) with regard to the concentration of microencapsulated isoamyl acetate. There was a decrease of 13.18% in the microencapsulated aroma with dextrin, 6.07% in the mixture of dextrin and maltodextrin and 13.75% for the fermented microencapsulated aroma with dextrin.
Table 5 Stability test regarding the concentration of encapsulated isoamyl acetate
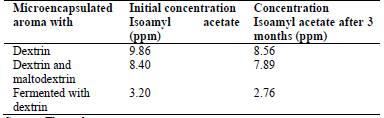
Source: The authors.
According to Phamhoang et al. [40], aroma compounds are very unstable molecules. When these volatile compounds are obtained by a powder microencapsulation technique, they become more stable and functional due to their controlled release. Water activity and storage temperature are the most important factors in the control of microcapsule stability. In this research, the stability study showed that in 3 months the volatile compounds present in the initial powder remained stable.
4. Conclusion
Spray drying is a promising drying technique for obtaining microcapsules of flavour compounds naturally obtained by submerged fermentation. Among the tested agents, the highest encapsulation efficiency was obtained using dextrin, while the lowest efficiency was with maltodextrin. The aromas produced were compatible with the characteristic aromas of banana and other fruit notes. Also, the sensory test showed high acceptability by the evaluators. However, improvement in some respects was suggested, as they identified some fermented notes and other residual aromas.