INTRODUCTION
Throughout its existence, humans have faced hunger and diseases periods that have threatened with its extinction. Their survival has depended on the ability to store energy and use it in times of limited food availability as well as the ability to cope with infections and the damages caused by them.
This is how the metabolic and immune systems have ensured the basic requirements for survival and have evolved together, although independently. Several hormones, cytokines, transcription factors, and lipids participate in both systems and, in addition to using the same metabolic and immune mechanisms, they can regulate each other [1]. For instance, a normal inflammatory response needs active metabolic pathways to divert energy, which is the case of stored lipids that are mobilized in the fight against an infection during the acute phase response. The inflammatory response (obesity is considered a state of chronic inflammation) favors a catabolic state and suppresses anabolic pathways, such as the insulin signaling pathway [2].
Nowadays, excessive consumption of energy-rich foods has now become a serious public health problem in the world, which, along with wrong lifestyles, has led to the emergence of two diseases which are characteristic of the Western Hemisphere: obesity and type 2 diabetes. The biochemical consequences in an overweight organism are energy imbalance and metabolic stress that produce an inflammatory and oxidizing response [3].
Initially, the treatment of these two diseases is aimed at decreasing weight and glucose in the plasma by means of diet and aerobic exercise. However, lack of commitment to this treatment causes hypoglycemic agents and medications to be prescribed in order to help body weight regulation.
Hypoglycemic agents on the market, although effective in controlling plasma glucose, have undesirable and sometimes serious side effects for the individuals who take them; In addition, the medications used to maintain body weight are not safe enough for human health. Therefore, it is necessary to search for new pharmacological alternatives with fewer side effects that allow combatting the symptoms of these two diseases: inflammation and hyperglycemia by regulating the molecules involved [1].
Lippia alba Mill N.E. Brown (Family Verbenaceae) is an aromatic plant of the Center, South America, and the Caribbean. Traditionally, it has been used for the treatment of various diseases such as diabetes, hypertension, respiratory and digestive conditions [4-6]. From this plant, different essential oils are extracted. In the present study, L. alba chemotype carvone was used, which has been tested due to its antifungal, antibacterial, anxiolytic and antioxidant activity [7-10]. The latter property is very interesting, as several studies suggest that oxidative stress plays a central role in the pathogenesis of various metabolic diseases such as diabetes and obesity [11, 12].
The objective of this study was to evaluate the metabolic effect of Lippia alba Mill N.E. Brown chemotype carvone EO on molecules related to type 2 diabetes mellitus (T2DM) and obesity as TNF alfa and adiponectin cytokines using Wistar rats as test subjects.
METHODOLOGY
Animals
This study used Wistar rats ranging from 200 to 250 g, which came from Universidad Industrial de Santander's Health Faculty's Bioterium; The rats were kept in optimum housing conditions, light and dark cycles of12 hours, water and food ad libitum, temperature 22 ± 2°C and humidity 50-60%. In all procedures, the ethical considerations of Directive 2010/63 of the European Union were taken into account [13]. The Ethics Committee of Universidad Industrial de Santander - ECINCI approved this work.
Toxicity analysis
To test the acute oral toxicity of the oil, the methodology proposed by the OECD was followed [14]. Twenty-four Wistar rats (12 females and 12 males) were divided into four groups of females and four groups of males. They were given 0, 10, 200 and 500 µL / kg body weight of L. alba EO using 0.5% Carboxymethylcellulose (CMC) as carrier. Behavior changes and disease symptoms such as excitement, fatigue, lethargy, itching, hair loss, diarrhea, backache, tremor and death for 1 hour after 6, 12 and 24 hours and every day for 14 days were observed.
Blood samples collected after 14 observation days were used to determine, by commercial colorimetric kits, AST and ALT transaminases, markers of liver damage.
Induction of diabetes using streptozotocin
The induction of diabetes was performed in three groups of Wistar rats of five animals each, fasted for eight hours. Subsequently, a single intraperitoneal injection of streptozotocin (STZ) (Sigma, USA) of 50 mg/kg in fresh 0.1 M citrate buffer (pH 4.5) was applied [15]. In addition, a 20% glucose solution was given during the following 24 hours which allowed them to cope with the initial hypoglycemic effects of STZ [16].
Essential oil of Lippia alba Mill N.E. Brown
The essential oil (EO) was obtained by microwave-assisted hydro-distillation (MWHD) of L. alba (Carvone chemotype), grown at the Research Center for Agro-industrialization of Tropical Medicinal Aromatic Vegetable Species (CENIVAM), Bucaramanga, Colombia, with a yield of 0.6% (w/w). The identification of the plant and its deposit are in the National Herbarium with voucher N°. 512078. EO characterization (see table 1) was performed by gas chromatography coupled to mass spectrometry with the methodology published [8,9,17] at Universidad Industrial de Santander's -UIS Center of Chromatography and Mass Spectrometry -CromMass.
Experimental design with working groups
Male Wistar rats were divided into five groups (see table 2), each with five animals. The 0.5% CMC compound was used as carrier. The highest non-toxic dose of EC was chosen. Diabetes in rats was induced with STZ as specified in the methodology. All doses were given daily for 21 days and were administrated orally by gastric tube.
During the 21 days of treatment, the body weight of each animal was recorded. After day 21, the animals were fasted for 12 hours and sacrificed by decapitation; their blood was collected, and the serum was separated by centrifugation to perform the determinations using commercial cholesterol, triglyceride, AST, ALT and ALP colorimetric kits.
Essential oil effect on the intestinal glucose absorption glucose and concentration of triglycerides and serum glucose concentration
First, the absorption and metabolism of plasma glucose against an oral glucose load were evaluated using an AccuTrend® plus kit and glucose test strips from Roche Laboratories. To perform the glucose curve, before starting EO treatment, the first blood sample (basal value) was taken on fast without supplying the glucose load to the animal, the second sample was taken thirty minutes after having given the glucose load, and the third and fourth samples 60 and 120 min, respectively, after having given the glucose load to the animals. The glucose load applied was 2 g of glucose per kg of animal weight.
Second, evaluation of serum glucose and triglyceride concentrations of fasting animals after 21 days of treatment was performed using commercial colorimetric kits.
Evaluation of adipocytokines
The serum concentration of adiponectin and TNF-α of the animals under study after 21 days of oral treatment with the essential oil was determined by the ELISA technique following the protocol suggested in kits ab108784 and ab46070 abcam® Laboratories.
Statistical analysis
A one-way analysis of variance (ANOVA) was performed using the Tukey HSD test for normalized data and the non-parametric Kruskal-Wallis test, followed by Tukey HSD for non-normalized data ref. A value of p <0.05 was considered statistically significant. Statistical packages SIGMASTAT® and STATISTICA 8.0® were used.
RESULTS
Toxicity analysis
Toxicity studies revealed that in the control group the doses evaluated (10, 200 and 500 mg/kg) were safe. Animals of either sex showed no signs of toxicity or change in their behavior, which was corroborated by liver function evaluation. The AST and ALT hepatotoxicity markers did not show any variations with regarding the control group at the doses evaluated for the L. alba EO.
Glucose absorption and metabolism, and diabetes induction with STZ
The oral glucose tolerance test performed on all work groups prior to initiating EO treatment did not show alterations in glucose uptake, figure 1, including the groups that were induced diabetes with STZ. At minute 30, all groups evaluated were able to absorb glucose (serum increases in glucose concentration) and were also able to regulate it at minutes 60 and 120 (serum decreases in glucose concentration). This decrease is caused by the presence of insulin. Therefore, it is evident that even the groups of animals that were induced by diabetes with STZ showed insulin remnants which resembles type 2 diabetes mellitus. This indicates that despite being treated with STZ, this drug's concentration is important in determining the model of diabetes to be developed [18-20].
Metabolic effects caused by the ingestion of EO from Lippia alba
Effect on body weight.
The weight gained by the animals in the different groups showed significant differences since the animals submitted to the EO treatment gained less weight than the animals in the groups that did not receive it (figure 2).
Cumulative hepatotoxicity analysis
In figure 3, the results of analyses performed to determinate the levels of AST liver enzymes and ALT are presented.
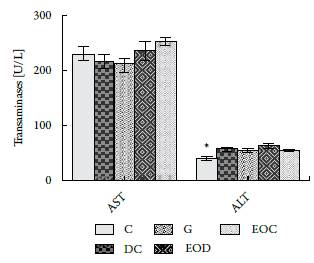
Figure 3 Activity of liver enzymes AST and ALT in serum after 21 days of treatment with EO. Groups: (C) control, (DC) Diabetic, (G) Diabetic Glibenclamide 10 mg / kg, (EOD) diabetic EO and (EOC) control EO. Data are presented as means ± SEM. P <0.05 with respect to EOC (*).
The evaluated aminotransferases do not show that EO has long term toxic consequences, only significant differences were found between groups C and EOC of the ALT enzyme. However, their difference does not reach between 3-15 times the value of the normal control, which could indicate the presence of hepatic injury. There are other factors that may alter this enzyme such as body mass index, food intake, exercise, among others [21]. These factors are congruent with the results found in this study with respect to body weight.
Serum glucose and triglyceride concentration.
In figure 4, a significant decrease was observed in the EOC group of glucose and triglycerides regarding group C. The decrease in glucose and triglycerides corresponds to 33 and 35%, respectively; Values that are lower than those presented by the group of animals treated with Glibenclamida® (group G). It is noteworthy that the animals that were induced by diabetes and that were treated with the EO, EOD group, did not show significant changes with by comparison to the control diabetic group, DC, in terms of triglycerides and glucose.
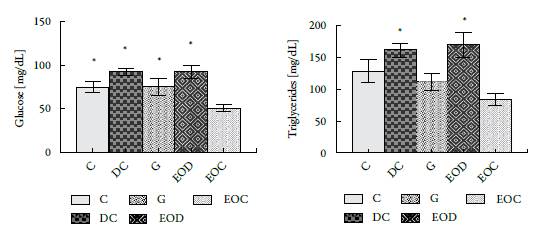
Figure 4 Effect of administration of L. alba EO at the end of 21 days of treatment in biochemical analyzes: (A) Glucose and (B) Triglycerides. Groups: (C) control, (DC) Diabetic, (DG) Diabetic Glibenclamide 10 mg/kg, (EOD) diabetic AE and (EOC) AE control. All results presented are expressed in [mg/dL]. * P <0.05 with respect to the EOC group.
Effect on adipocytokines.
The essential oil's effect on evaluated adipocytokines, adiponectin and TNF-a, shows that adiponectin from the groups of animals that consumed EO (EOD and EOC) did not differ significantly from the control group (C), figure 5A.
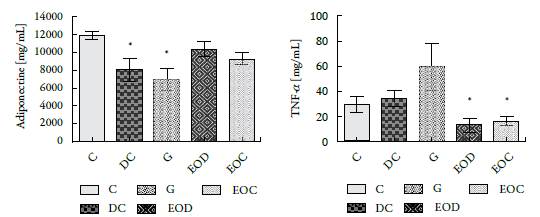
Figure 5 Concentration of (A) Adiponectin and (B) TNF-α, present in the serum of the different groups of animals evaluated after 21 days of treatment. Groups: (C) control, (DC) Diabetic, (G) Diabetic glibenclamide 10 mg/kg, (EOD) diabetic AE and (EOC) AE control. Values are presented as means ± SEM. * P <0.05.
As for TNF-α, the groups of animals treated with the essential oil (EOD and EOC) showed a decrease in the plasma concentration of this cytokine compared to that presented by its controls, figure 5B. Interestingly, the group of diabetic animals treated with glibenclamide (G), commercial hypoglycemic, showed a significant increase in TNF-α concentration compared to their control, the DC group and the animals treated with the essential oil.
DISCUSSION
The essential oil of L. alba Mill N.E. Brown, carvone chemotype, used in the present study, is non-toxic at the concentration used (500 µL/kg). There were no deaths, behavioral changes, or hepatotoxicity (did not occur with either single dose or repeated doses of EO). Therefore, its LD50 is much higher than 500 µL/kg b.w. This is supported with Olivero-Verbel et al. [17], who evaluated the toxicity of the essential oil of L. alba Mill N.E. Brown, citral chemotype, administered intraperitoneally. The authors found that the toxic effects of this oil were shown with doses equal to or greater than 1000 mg/ kg (b.w.).
About figure 1, it can be deduced that STZ does not alter the absorption of glucose at the intestinal level, this is supported by the increase of glucose 30 minutes after the glucose load supply to the animals. On the other hand, it should be emphasized that the dose of this drug in the study does not completely destroy the Langerhans (3 cells responsible for insulin production (the slope observed in figure 1 between minutes 30-120). Which brings the model used closer to what happens in T2DM.
An interesting effect shown by this EO is the regulatory effect of body weight (see figure 2). Asnaashari et al. [22]) report that the essential oil of Citrus aurantifolia, whose main component is D-limonene (28.27%), also prevents body weight gain, due to a decrease in appetite and energy. Therefore, Najafian [23] argues that body weight gain can be reduced by administering essential plant extracts or oils because they contain molecules that act as inhibitors of a-amylases. In fact, Najafian and Ebrahim-Habibi [24], who worked with citral, a compound found in several essential oils like Back-housia citriodora, L. alba (citral chemotype), among others, found that it promotes weight loss, decreases levels of glucose and has moderate activity in the inhibition of a-amylases. This could explain the reduction in serum glucose levels as well as body weight gain. In contrast with the results reported by Najafian, the present research found a normal glucose absorption, then the a-amylase inhibitions is not the pathway that explains the weight loss. The identification of the weight loss metabolic pathway reason is beyond of the research scope.
Animals in the EOC group decreased glucose and triglyceride levels by 33 and 35%, respectively, compared to the control group. It is noteworthy that in the animals that were induced diabetes with STZ, there are no significant changes in glucose and tri-glyceride concentrations compared to their controls which could indicate that the oil cannot reverse the metabolic effects caused by the STZ (see figure 4).
As for adipocytokines, it was found that EO caused a significant decrease in TNF-alpha between the groups treated with EO (EOC and EOD) congruent with the anti-inflammatory properties of EO. On the other hand, the results show that there are no significant changes in the concentration of adiponectin in the different treated groups (see figure 5A). The observed changes for this molecule could be since the groups treated with the oil were the ones that gained the least weight (adiponectin increases have been reported when there is weight loss [25-27] or could be due to genetic activation caused by Oil. New experiments should be planned to solve this dilemma. Increases in adiponectin and decreased TNFα are related in the literature with decreased resistance to insulin action [11, 27-29].
The increase in TNFα caused by the commercial hypoglycemic agent glibenclamide, which, together with the body weight achieved by these animals, would indicate that in subsequent studies this drug should not be used as an internal control (see figure 2 and figure 5 B).
CONCLUSIONS
In conclusion, the decrease in TNF-α and body weight presented by the animals treated with L. alba, carvone chemotype EO indicate that its components represent a therapeutic alternative in the treatment of disease as obesity and T2DM complementary to hypocaloric diet and aerobic exercise.