Introduction
Identifying alternative protein sources that could substitute fishmeal (FM) in aquaculture diets is a major challenge (Oliva-Teles, 2012). Because of its excellent lipid and protein profiles, as well as high biomass production, microalgae are potential substitutes for FM (Palmegiano et al., 2008). Among microalgae, Athrospira platensis has been widely used in animal and human nutrition because of its high protein content (60-70%), and can be used as a feedstuff in fish diets (Belay, 2002). Indeed, A. platensis has already been used as a FM replacement in aquaculture diets, improving growth performance of tilapia Oreochromis niloticus (Abdel-Tawwab and Ahmad, 2009), common carp (Cyprinus carpio L.) (Teimouri et al., 2015), and sturgeon (Huso huso) (Adel et al., 2016). Due to its bioactive components, A. platensis improves carcass quality (carotenoids) (Nandeesha et al., 1998), and provides immune stimulants (polysaccharides) (Abdel- Tawwab and Ahmad, 2009), antioxidant activity (β-carotene and C-phycocyanin) (Ravi et al., 2010), and polyunsaturated fatty acids (-linolenic acid - GLA) (Jafari et al., 2014). Besides improving growth parameters, it enhances the immune system -critical in aquaculture production- due to a wide variety of macro and micronutrients (Ringø et al., 2010). Dietary administration of A. platensis might benefit specific and non-specific cellular immune parameters in fish, as already shown in macrophage and natural killers cell (NK) activities in tilapia (Oreochromis niloticus) (Abdel-Tawwab and Ahmad, 2009), increasing mucus protease production in sturgeon (Adel et al., 2016), interleukin gene expression in C. carpio leucocytes (Watanuki et al., 2006), and granular hemocytes in white shrimp (Litopeneaus vannamei) (Macias-Sancho et al., 2014).
The mullet (Mugil liza) has desirable characteristics for aquaculture, including its iliophagus habits (Vieira, 1991), fast ontogenic development (Galvão et al., 1997), and easy adaptation to consuming novel dietary ingredients (Ramos et al., 2015; Zamora-Sillero et al., 2013). Therefore, we evaluated the effects of A. platensis as a FM substitute in practical diets on growth performance and non-specific immune cellular response of juvenile mullets
Materials and Methods
Fish source and experimental design
Once approved by the Ethics Committee (FURG- CEUA Pq036/2014), juvenile mullets (n=210) were captured using a 3 mm beach seine net at Cassino Beach/ Brazil (Latitude, -32.1833; Longitude, -52.1667) and taken to Laboratório de Nutrição de Organismos Aquáticos-FURG at the Universidade Federal do Rio Grande (FURG), Brazil. The fish were acclimated to the laboratory conditions for one month in a 500-L tank under controlled temperature (26 °C) and salinity (30 ppt). During the acclimation period, the mullets were hand-fed the control diet (D1) four times per day (at 9:00, 12:00, 14:00, and 16:00). To maintain water quality conditions, a full water renewal was carried out every day.
After the acclimatization period, an 80-day experiment was conducted in 15 50-L fiberglass tanks connected by a recirculation system, consisting in a biological filter, a UV light filter (18 w Philips®, São Paulo, Brazil) and a protein skimmer. During this period, water flow rate was 3 L/min, and a daily water exchange corresponding to 10% of the total tank volume was carried out. Each tank was stocked with 14 individuals (0.26 ± 0.01 g initial weight). Water quality parameters remained stable throughout the experimental period. Water parameters were measured daily in all tanks. Dissolved oxygen concentrations and temperature were measured using a multi-parameter electrode (YSI, 550A, Yellow Springs, Ohio, USA) and maintained at 6.1 ± 0.6 mg/L, 25.9 ± 0.6 °C, respectively. The pH was measured with a digital pH meter (Hanna Instruments, HI221) and presented a mean value of 7.7 ± 0.1. Salinity was kept constant at 29.2 ± 0.8 ppt and was measured with an optical refractometer (RTS 101, ATAGO, Tokyo, Japan). A photoperiod of 12:12 h (light: dark) was maintained. The ammonium and nitrite concentrations were determined according to the methods presented by Benderschneider and Robinson (1983), and Strickland and Parsons (1972), respectively. Total ammonia and nitrite levels were 0.17 ± 0.09 mg/L and 0.31 ± 0.34 mg/L, respectively. Alkalinity was maintained by the addition CaCO3 (to maintain 100 mg/L of CaCO3 in the water), thereby maintaining the biofilter. Alkalinity was measured according to APHA (2005).
Five experimental diets were prepared by replacing 0 (D1), 30 (D2), 50 (D3), 70 (D4) and 100% (D5) of FM with A. platensis. Each diet was tested in triplicate tanks, which were randomly distributed. Fish were fed four times per day (9:00, 12:00, 14:00, and 16:00). At each feeding, fish were fed 10% of the total weight biomass per tank for the first 15 experimental days, and adjusted to 7% of the total biomass for the remaining days. Biomass was calculated daily assuming a feed conversion ratio of 2:1.
Diet formulation
A commercial A. platensis microalgae was used (Prilabsa®, Natal, Brazil). Composition of all feed ingredients was analyzed at Laboratório de Nutrição de Organismos Aquáticos-FURG according to the Association of the Official Analytical Chemists (A.O.A.C., 2000) methodology. Table 1 presents the proximal composition of A. platensis and the FM used in the study.
Table 1 Proximal composition of fishmeal and A. platensis in dry basis (g/kg).

a Calculated value (Merrill and Watt, 1973). NFE= Nitrogen Free Extract, calculated as 100 − (crude protein + lipids + ash + moisture + fiber).
Table 2 Diet formulation and final composition. Proximate analyses values expressed in g/kg.
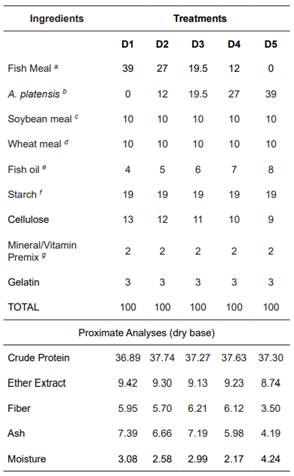
a Leal Santos, Rio Grande, RS, Brazil; b Prilabsa® , Brazil; c Sulino RS, Brazil; d Sulino , RS, Brazil; e Campestre®, São Paulo. Brazil; f Maizena, Brazil ®; g Premix M. Cassab, São Paulo, Brazil ((Vitamin A (500,000 lU/kg), Vit. D3 (250,000 lU/kg), Vit. E (5,000 mg/kg), Vit. K3 (500 mg/kg), Vit. B1 (1,000 mg/kg), Vit. B2 (1,000 mg/kg), Vit. B6 (1,000 mg/kg), Vit. B12 (2,000 mg/kg), Niacin (2,500 mg/ kg), Calcium pantothenate (4,000 mg/kg), Folic acid (500 mg/kg), Biotin (10 mg/kg), Vit C (10,000 mg/kg), Choline (100,000 mg/kg), Inositol (1,000 mg/kg). Trace elements: Selenium (30 mg/kg), Iron (5,000 mg/kg), Copper (1,000 mg/kg), Manganese (5,000 mg/kg), Zinc (9,000 mg/kg), Cobalt (50 mg/kg), Iodine (200 mg/kg)).
Diets were formulated to contain 35% CP (Carvalho et al., 2010) and 9% lipids. The pre-weighed ingredients were mechanically mixed (Marconi, MA200, São Paulo. Brazil), and then mixed with oil and water to produce a stiff dough. Then, mixtures were pelleted using a meat grinder (Metalúrgica 9000, PC-22, São Paulo, Brazil). Pellets were air dried at 60 °C for 24 h in an oven (Marconi, MA035). The size of the resulting pellets was gradually adjusted to fish growth. Diets were stored in plastic bags at -18 °C until use. Diet formulation and proximate composition is shown in Table 2.
Diet fatty acid identification
Fatty acid (FA) analyses were made at the Facultad de Ciencias, Universidad de la republica (Montevideo, Uruguay). Lipids were extracted according to Folch et al. (1957) and transesterified using 1 mL sulfuric acid (1%) in methanol (Christie, 1982). The antioxidant butylhydroxytoluene (BHT) (0.5 mL, 50 mg/L) was used to prevent FA oxidation. Samples were incubated at 49 ºC for 16 h in a nitrogen atmosphere. Next, hexane:ether (1:1 v/v) solution was used for FA extraction and KHCO3 (20g/L) was used to wash the hexane:ether solution. Finally, FA was dried for 24 h, and a dilution of chloroform 30 mg/mL was made and kept under a nitrogen atmosphere at -20 ºC until chromatography was performed.
The FA was quantified using gas chromatography (Hewlett Packard 5890, GMI, Minneapolis, MN, USA), with a capillary column of melted silica Supelco wax as stationary phase (30 m × 0.32 mm D.I., Supelco, Pennsylvania, USA). Nitrogen was used as carrier gas and split mode for the injection. Injector and detector temperatures were both 250 °C. Initially, temperature was 180 °C for 10 min, then increased at a rate of 2.5 °C/min up to 212 °C. Final temperature was maintained for 13 min. Chromatography Station for Windows (CSW Data Apex 1.7) was used for data processing of chromatograms. All FA were identified (Table 3) by comparing its retention time with cod fish oil standard (Supelco), according to Salhi and Bessonart (2013).
Amino acid evaluation in diets
The amino acids (AA) contained in the diets were calculated according to the AA profile of the principal protein sources (FM and Spirulina) analyzed by Evonik Industries AG (São Paulo, Brazil) by the AMINONIR® (Nitrogen infrared, NIR) technique and data gathered from the NRC (2011) (for soybean meal, wheat meal and gelatin), then calculated according to the inclusion of each ingredient (Table 4).
Evaluation of growth parameters and sampling
At the end of the 80-day experiment, each organism was anesthetized (benzocaine 50 ppm) and individual weights were obtained to determine growth parameters. The performance parameters were:
Weight gain (WG) = individual final weight (g) - individual initial weight (g)/individual initial weight (g);
Feed conversion ratio (FCR) = average individual dry feed intake/average individual weight gain;
Specific growth rate (SGR) = 100% × [ln (final weight) - ln (initial weight)]/days of feeding;
Protein efficiency ratio (PER) = average individual weight gain (g)/average individual protein intake (g);
Survival = (final number of fish/initial number of fish) * 100;
Afterwards, blood samples were collected from the caudal vein of six fish per treatment and a drop of blood was smeared onto a clean glass slide and dried. Next, all fish were euthanized with a benzocaine overdose (300 ppm). Nine samples of liver and spleen from each treatment were collected and fixed in 20% formalin solution for subsequent analyses. The carcasses of 12 fish per treatment were frozen for body composition analyses. All proximal analyses were conducted according to the AOAC (2000) methodology.
White blood cell count
Smears of blood were fixed in methanol and stained with Wright-Giemsa stain for determination of the differential White Blood Cell (WBC) count. At least 100 WBCs for each smear were counted for differential WBC determinations under an optical microscope. Six smears for each tank were counted.
Immunohistochemistry (IHC)
For IHC, analyses were conducted at Laboratório de Imunologia e Patologia de Organismos Aquáticos (FURG). Five spleens per each tank were fixed in 20% buffered formalin, embedded in paraplast and stained using the ABC peroxidase method (Vectastain Elite ABC Kit, California, USA), as described by Hsu et al. (1981). The sections were incubated with monoclonal anti-CD3 antibodies (Sigma®, USA), as previously tested by Romano et al. (2004) and Führ et al. (2016). Subsequently, the sections were washed (0.1% diaminobenzidine solution) and dehydrated; six slides per tank were examined under optical microscope.
The CD3 receptor expression was evaluated by quantitative analysis of phenotypic percentage per square millimeter (mm2) of tissue. The expression of these receptors in the spleen was quantified using Bioscan OPTIMAS 6.1 software according to the method proposed by Weibel (1981) and Romano et al. (1996).
Apoptosis evaluation
Five livers per tank were fixed in 20% buffered formalin and embedded in paraplast; the evaluation was conducted using the TUNEL method for ApopTag® Plus Peroxidase In Situ Apoptosis Detection Kit (Millipore), according to Charriaut-Marlangue and Ben-Ari (1995). Apoptotic cell expression was evaluated using quantitative analysis of the expression percentage cells per square millimeter (mm2) of tissue. The apoptotic cells in the liver were also quantified using Bioscan OPTIMAS 6.1 software.
Statistics
To test possible differences among treatments, we used one-way Analysis of variance (ANOVA). Normality and heterogeneity were tested according to Shapiro-Wilk and Levene tests, respectively. Percentage data were transformed into arcsine values before statistical significance tests. For each case, when significance between treatments was detected, a posterior means comparison was performed by Tukey’s test. All tests were conducted at the 5% significance level.
Results
A summary of growth parameters is given in Table 5. Except for FCR, all parameters increased for up to 50% FM substitution (D3 treatment), followed by gradual decrease until D5 treatment. When fed D5, almost all growth parameters were different from the other diets. Final weight (FW) and weight gain (WG) showed no significant difference between D1 and D5 (Table 5). Mullet survival ranged from 100 to 47.62%, and was different for full FM substitution compared to all other treatments.
For proximal analyses of the carcass, we detected no statistically significant differences among treatments (Table 6).
Table 5 Growth performance of mullets fed different Arthrospira platensis levels in substitution of fish meal.
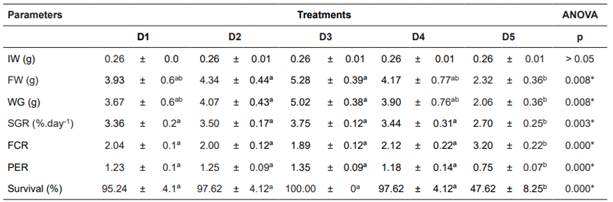
Values are expressed as means ± SD of three replicate groups. Different letters within (a, b) each row show significant differences in Tukey test at p≤0.05. IW= initial weight; FW = final weight; WG = weight gain; SGR = specific growth ratio; FCR = feed conversion ratio; PER = protein efficiency ratio.
Table 6 Whole-body proximate composition of mullets (wet weight basis, g/kg) fed different Arthrospira platensis levels in substitution of fish meal.
Values are expressed as means ± SD of three replicate groups. Different letters within each row show significant differences in Tukey test at p≤0.05.
Table 7 White blood cell count, lymphocytes (%), monocyte (%) and granulocytes (%), CD3 and apoptosis reactive cells (mm2) of mullets fed different Arthrospira platensis levels in substitution of fish meal.
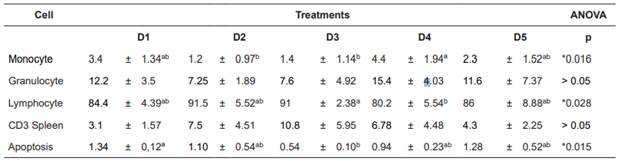
Values are expressed as means ± SD of three replicate groups. Different letters within (a, b) each row show significant difference.
The proportions of WBCs varied among treatments (Table 7). Proportion of monocytes was different between D2 and D3 treatments compared to D4 treatment. Proportion of lymphocytes was different between D3 and D4 treatments. However, no statistically significant differences among treatments for the granulocyte or T-Cell CD3 receptor expression were detected. Apoptotic expression was different between treatments without A. platensis (1.34 ± 0.12 mm2), and D2 treatment (0.54 ± 0.10 mm2) (p=0.015).
Discussion
Spirulina has been widely recommended by the FAO as a food supplement in humans and as a high-quality feed ingredient in animal nutrition (Habib et al., 2008). The use of these microalgae as a FM substitute has been tested in many fish species and at various levels of FM substitution. El-sayed (1994) found that 50% of the FM substitution results in the best growth for silver seabream (Rhabdos argussarba). Palmegiano et al. (2005) show that 50% of Spirulina inclusion results in the best growth, FCR, and PER performance for sturgeon (Acipenser baeri). Rincón et al. (2012) observed that 30% of FM substitution for Spirulina results in the best growth and FCR for Oreochromis sp. Velasquez et al. (2016) found that the best FCR and PER could be achieved at 30% of Spirulina substitution in tilapia (Oreochromis niloticus). In the present study, we demonstrated that up to 50% of FM might be substituted with A. platensis in diets for juvenile mullets, showing that this level of inclusion produced the best weight gain, FCR, and PER results. However, total substitution of FM (D5) resulted in reduced growth performance and survival (47.62%).
The poor growth and survival rates observed at full FM substitution (D5) might be related to an imbalance in FA and AA. Since AA requirements are species-specific, the quality of dietary AA may affect growth, survival, or both (Li et al., 2009). For this reason, FA and AA compositions were evaluated for all diets. Lipid analyses demonstrated no variation in the quantity of essential fatty acids (EFA) among the tested diets. Neither the content of total n-3 HUFA nor the content of DHA or DHA/EPA ratio presented differences between diets that might have accounted for the survival and growth results. However, the AA calculation table showed a decrease in histidine (-18.44%) and lysine (-21.01%), when comparing the full FM diet (D1) and the 100% A. platensis diet (D5). Each of these AA plays an important role in fish growth and survival (Hauler and Carter, 2001).
Borlongan and Coloso (1993) observed that a histidine deficiency could result in elevated mortality and small differences in Milkfish (Chanos chanos) growth. Waagbø et al. (2010) found a promotion in salmon (Salmo salar) growth for diets supplemented with histidine, while lysine deficiency had no effect on survival but could strongly affect growth. The effects of lysine deficiency are observed in many species, including red sea bream (Pagrus major) (Forster et al., 1998), striped bass (Morone saxatilis) (Small and Soares, 2000), and black sea bream (Sparus macrocephalus) (Zhou et al., 2010). As such, it is clear that deficiency of these AAs in A. platensis might affect mullet survival and growth.
Immune system enhancement through the diet has been widely used to improve health and growth of cultured fish (Pohlenz and Gatlin, 2014). Spirulina has been tested for its immune-stimulant properties in fish feed at low inclusion levels (up to 10% inclusion). It has several effects on the immune system of fish species, by enhancing phagocyte, serum and complement activity in carp (Cyprinus carpio) and trout (Onchorincus mykiss) (Amar et al., 2004; Watanuki et al., 2006), increasing resistance against pathogens in shrimp (Litopenaeus vannamei) and tilapia (Oreochromis niloticus) (Tayag et al., 2010; Ragap et al., 2012), improving hematocrit and lysozyme activity in tilapia (Oreochromis niloticus) (Ibrahem et al., 2013), and activating leucocyte functions (Adel et al., 2016), among other effects. However, to the best of our knowledge, there are no reports in the literature regarding the influence of full substitution of FM for Spirulina in fish diets with respect to immune stimulation.
According to Simsek et al. (2007), WBC production in rats is increased by dietary addition of Spirulina, with changes in the proportions of these cells. In the present report, significant differences were found in monocyte and lymphocyte counts between D2 and D3 treatments. In particular, the D3 treatment resulted in the lowest monocyte proportion (1.4 ± 1.14%) and elevated lymphocytes (91 ± 2.38%). This same trend was observed by Abdel-Tawwab and Ahmad (2009), where the lowest monocyte and the highest lymphocyte production matched the diet that showed the best growth for tilapias fed Spirulina inclusion (5%). Watanuki et al. (2006) observed an increase in macrophage activity in diets supplemented with Spirulina in carp (Cyprinus carpio). This effect was also obtained by supplementing β-carotene in trout (Oncorhinchus mykis), which increased the protection of macrophage receptors against oxidative stress (Amar et al., 2000); Spirulina is known to be a rich source of carotenoids, such as β-carotene (Leema et al., 2010). These findings suggest a more efficient response to stress (monocyte activity) when β-carotene is present, which might account for the decrease in the production of this cell type observed here.
The spleen is a secondary immune organ consisting of lymphoid tissue, in which maturation of T-Cells occurs (Manning, 1994). The T-Cell co- receptor expression has been linked to the speed of development of lymphoid tissue (Miceli and Parnes, 1993). The CD3 complex technique has been recently used in fishes to detect the CD3 co-receptors on the T-Cells (Øvergard et al., 2009). The CD3 co-receptor analyses of spleen cells did not show any significant difference between treatments. Although fish from the 50% treatment showed the highest quantity of CD3 co-receptors (10.8 ± 5.95 mm2), and those of the control treatment had the lowest reaction (3.1 ± 1.57 mm2), this did not reach statistical significance. In some monogastric animals, β-carotene can be metabolized into various vitamin A metabolites, including Retinoic acid (RA) (Wang, 1994). RA can enhance T-Cell proliferation and stimulate a more accurate immune response (Ertesvag et al., 2002, Ross, 2012). In this context, Spirulina has been shown to stimulate proliferation of T-Cells on lymphoid tissue in rats (Simsek et al., 2007), increase lymphocyte proliferation in the spleen of parrotfish (Oplegnathus punctatus) (Tachibana et al., 1997), and trout (Amar et al., 2000). Our results suggest that Spirulina might have stimulated the development of lymphoid tissue, which results in a major detection of CD3 co-receptors. Moreover, Führ et al. (2016) suggested that a greater quantity of expressed CD3 co-receptors might represent the most immune stimulated physiological situation in fish.
Cell deletion is an important mechanism for health and disease maintenance (Elmore, 2007). Apoptosis might occur as a controlled alternative to infected cells (Valentim-Neto et al., 2014), but also via the incapacity of cells to resist ambient stressors (Tabas and Ron, 2011). Ibrahem et al. (2013) found that Spirulina might promote expression of the P53 protein, which plays an important role in cell maintenance and repair in tilapia. Here we detected significant differences in apoptotic expression between the treatment without A. platensis (D1) and the treatment with 50% substitution (D3). In this case, the decrease in the apoptotic process in organisms not exposed to pathogen agents is probably due to a more efficient cell response to oxidant damage and repair. The same response was observed by Chu et al. (2010) with Spirulina extract in fibroblast cells, and Macias-Sancho et al. (2014), wherein less apoptotic cells were observed in white shrimp feed with Spirulina.
In conclusion, A. platensis might be suitable as a FM substitute (up to 50%) in a practical fish diet for mullet. Also, partial substitution of FM for A. platensis affected the proportion of WBCs, improving non-specific cellular immune response of mullets by increasing the production of T-Cells and decreasing cell apoptosis.