Introduction
Effective preservation of tropical grass is a management strategy to ensure the availability of feed for animals throughout the year (Daniel et al., 2019). Tropical grass silage species are easy to manage, have high biomass production, and high nutritional value during the wet period (Tomaz et al., 2018). However, according to the same researchers, in this vegetative stage the grass has a high moisture content, which hinders its preservation in silage form and favors the growth of undesirable microorganisms (e. g., Clostridia), resulting in secondary fermentation and effluent production.
Among tropical grasses, elephant grass (Pennisetum purpureum Schum.) is one of the most widely used species in silage production because it is easy to handle and highly productive [approximately 80 tons of dry matter (DM)/ha/ year] (Rocha et al., 2017). However, similar to other tropical grass species, elephant grass has high moisture content (75-82%) at the ideal cutting stage, thereby strategies are needed to improve the fermentative profile of the silage (Santos et al., 2013). Absorbent additives may be used to improve the fermentation process and reduce effluent losses in tropical grass silages. These additives act as moisture absorbing agents, increase the DM content of the ensiled material, produce minimal leachate, and indirectly inhibit the action of spoilage microorganisms (Daniel et al., 2019). Thus, additives can improve silage quality of tropical grasses (Rêgo et al., 2010).
The Amazon biome holds a high diversity of oilseeds that are used in agribusiness to produce cosmetics, fuels, food, and other products (Funasaki et al., 2016). Among these, murumuru (Astrocaryum murumuru) stands out as a promising species for oil extraction, which generates a byproduct during this process (Mota et al., 2015). Usually, such byproduct has a high DM (>85%) and satisfactory chemical composition, making it suitable for inclusion in ruminant diets (Menezes et al., 2016).
The objective of this study was to determine the effects of including increasing concentrations of murumuru meal on the fermentative characteristics, microbiological activity, aerobic stability, and chemical composition of elephant grass silage.
Materials and methods
Elephant grass was grown in a grassland area, located at 1°27′07″S, 48°26′14″W, at an altitude of approximately 15 m. The climate of the region, according to the Köppen-Geiger classification, is Af type. The grass was harvested manually near ground level at 60 d of growth and then processed in a forage chopper to obtain 1.0 cm theoretical particle size (Nogueira, Itapira, São Paulo, Brazil). The material was ensiled in 15 L plastic buckets, with sand and non-woven fabric (TNT) on the bottom to quantify effluent production. An average of 15 ± 2 kg of forage or forage natural matter (NM) was ensiled in each silo, at 600 kg/m3 density.
Murumuru meal was sourced from a local company in Ananindeua, Pará (Brazil). The meal is the byproduct of mechanical extraction of oil from murumuru seeds. Before ensiling, elephant grass and murumuru meal samples were collected for chemical characterization (Table 1). Several murumuru meal concentrations [0.0, 7.0, 14.0, 21.0, and 28.0%, depending on the NM of the ensiled mass] included in elephant grass ensiling were evaluated.
After processing, elephant grass and murumuru meal were weighed, homogenized, and compacted in the silos. Subsequently, the silos were sealed and weighed. After 93 d, the silos were re-weighed to quantify dry matter recovery (DMR; Jobim et al., 2007). Then, the silos were opened and weighed. The visually deteriorated portion was weighted (WDM). Then, the sand and TNT were considered to determine the effluent losses (EL), and silage losses were estimated using equations proposed by Jobim et al. (2007), disregarding the deteriorated silage portion from the total weight of the silo after opening. An 800 g sample was taken from each silo, part of which was used for microbiological evaluation; the remaining sample was packed in plastic bags and stored at -20 °C for further chemical composition analysis and evaluation of fermentative characteristics.
The microbiological evaluation was performed using an aqueous extract composed of 25 g of silage and 225 mL of peptone water (1 g/L of water), which was manually homogenized for 3 min in closed sterile bags. The extract was surface-plated on potato dextrose-agar (Sigma- Aldrich, Ribeirão Preto, São Paulo, Brazil). Yeast and mold colonies were counted after incubation at 26 °C for 2 and 5 d, respectively. The pH of the aqueous extract was measured at time zero using a potentiometer (Tekna, São Bernardo do Campo, São Paulo, Brazil). Ammonia nitrogen (N-NH3) analysis was performed according to method No. 941.04 (AOAC, 1990).
For the organic acids (lactic, acetic, propionic, butyric, isobutyric, valeric, and isovaleric acids) evaluation, 25 g samples were weighed, solubilized in 300 mL of deionized water, and processed in a stomacher-type homogenizer (Cole-Parmer, Madison, Wisconsin, USA). Subsequently, 1 mL of the supernatant liquid was removed for gas chromatography. An Agilent 7890A gas chromatograph equipped with a flame ionization detector (7683B) and a fused silica capillary column (J & W 19091F-112; Agilent Technologies, Santa Clara, CA, USA) was used. After that, total organic acids were determined summing all evaluated acids. The lactic acid and acetic acid ratio (LA:AA) was established by dividing lactic acid by acetic acid contents.
For evaluating aerobic stability after opening the silos, 500 g of silage was placed in 25 plastic buckets (9 L), which were covered with aluminum foil to prevent loss of silage moisture. The buckets and silages were kept in an air-conditioned room at 20 °C for 12 d, where ambient and silage temperatures were recorded every 30 min using data loggers (Escort, Buchanan, Virgínia, USA). Aerobic stability was defined as the number of hours the silage remained stable before reaching 2 °C above room temperature.
The samples were pre-dried in a forced-air circulation oven at 55 °C for 72 h and then ground in a mill fitted with a 1 mm sieve. The DM, ash, ether extract (EE), and crude protein (CP) content was determined according to the AOAC (1990) methodology (methods No. 934.01, No. 923.03, No. 920.39, and No. 978.04, respectively). The methods by Van Soest and Robertson (1985) were used to analyze neutral detergent fiber (NDF), acid detergent fiber (ADF), and acid detergent lignin (ADI) levels, which were determined by solubilization of cellulose with sulfuric acid. Non-fiber carbohydrates were quantified according to the method by Detmann and Valadares Filho (2010). For the determination of total soluble, the methodology described by Dubois et al. (1956) was used.
Statistical analysis
A completely randomized experimental design with five replicates per concentration was used. Before statistical analysis, the molds count data were log10-transformed. The fermentation, microbiological characteristics, aerobic stability, and chemical composition data of elephant grass silage was submitted to analysis of variance and polynomial and simple linear regression analysis to study the effects of murumuru meal concentrations. For this, we used the SAS® Statistical Analysis Program, Version 6 (Statistical Analysis System, 2001), with 5% as the significance level.
The mathematical model was as follows: Yij = µ + Ti + eij. Where: Yij = observation j, referring to each inclusion level of murumuru meal (i); µ = overall average; Tij = effect of the inclusion level of murumuru meal; and eij = error associated with each observation.
Results
Chemical composition of elephant grass fresh and murumuru meal are presented in Table 1.
DM = dry matter, FM = fresh matter, OM = organic matter, CP = crude protein, EE = ether extract, NDF = neutral detergent fiber, ADF = acid detergent fiber, HEM = hemicellulose, CEL = cellulose, LIG = lignin, NFC = non-fibrous carbohydrate, and SC = soluble carbohydrates.
There was a reduction (p<0.05) in the effluent production of elephant grass silage with the inclusion of murumuru meal (Table 2). With the inclusion of murumuru meal, a quadratic effect (p<0.05) on the dry matter recovery of silages (DMR%) was observed along with an increase (p<0.05) in the portion of deteriorated material in the silages that included the additive. An increase (p<0.05) in mold count was noted with the inclusion of murumuru meal to elephant grass silage. Yeast was not detected (p>0.05) in the silage, that is, the count was below 2.0 cfu g-1 of forage.
A quadratic effect (p<0.05) was observed in the pH value with the inclusion of murumuru meal in elephant grass silage (Table 3). Ammonia nitrogen (N-NH3) content of silages increased linearly (p<0.05) with the inclusion of murumuru meal. There was no effect (p>0.05) of the additive on the lactic acid concentration of the silages.
Table 2 Fermentative losses and microbial composition of elephant grass silage containing murumuru meal.

NM = Natural matter; DMR = Dry matter recovery; PDM = Portion of deteriorated material.
Table 3 Fermentative characteristics and aerobic stability of elephant grass silages containing murumuru meal.
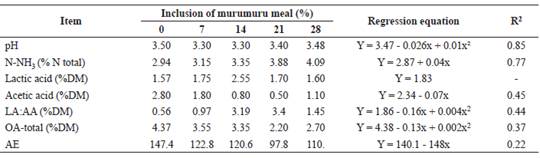
N-NH3/NT = ammonia nitrogen (% total nitrogen); DM = Dry matter; OA-total = Total organic acids; AE = aerobic stability; LA:AA = lactic acid/acetic acid.
However, there was a linear decrease (p<0.05) in the acetic acid content of silages with the inclusion of murumuru meal. A quadratic effect (p<0.05) was observed in the LA:AA ratio and the total organic acid concentration with the inclusion of murumuru meal in the elephant grass silage. Butyric, propionic, isobutyric, valeric, and isovaleric acids were not present in the silages. There was a linear reduction (p<0.05) in the aerobic stability of silages with the inclusion of murumuru meal.
The addition of murumuru meal in elephant grass silage linearly increased (p<0.05) the DM content of the silage (Table 4). However, it did not change (p>0.05) the OM or ash content of the silages. There was a reduction (p<0.05) in the CP content of silages with the inclusion of murumuru meal, while a quadratic effect (p<0.05) was noted on the EE levels.
A linear (p<0.05) reduction in the NDF content of elephant grass silages was observed with the inclusion of murumuru meal. Regarding the ADF, cellulose, and lignin levels, a quadratic effect (p<0.05) was observed with the inclusion of the additive. The hemicellulose content decreased linearly (p<0.05), and the NFC levels increased (p<0.05) with the inclusion of the additive to the silage.
Table 4 Chemical composition of elephant grass silage with the addition of murumuru meal.
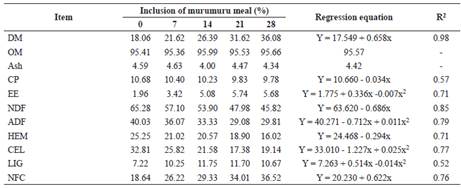
DM = dry matter, OM = organic matter, CP = crude protein, EE = ether extract, NDF = neutral detergent insoluble fiber, ADF = acid detergent fiber, HEM = hemicellulose, CEL = cellulose, LIG = lignin, NFC = non-fibrous carbohydrate.
Discussion
Absorbent additives can increase DM content of the silage (>85%), allowing them to act as moisture scavengers and increase the DM content of the ensiled material, reducing EL in high humidity silages (Razak et al., 2012). The murumuru meal used in this study had DM content above the minimum values suggested for a moisture sequestering additive. This characteristic allowed it to decrease effluent production in the grass silage (Table 2). This reduction has a direct effect on reducing the potential environmental impact of the effluent. Silage effluent is a pollutant that can cause eutrophication of water bodies (Gebrehanna et al., 2014).
The increase in PDM with the inclusion of murumuru meal was probably a reflection of the rise on NFC content on these silages. It is possible that the chemical composition of murumuru meal in elephant grass ensiling provided a greater input for the proliferation of undesirable microorganisms, which deteriorated the ensiled mass (Monteiro et al., 2011).
This was confirmed by the high mold count due to the inclusion of the additive. The fact that no yeast was observed in the silages does not necessarily mean that there was no presence of this group of microorganisms. Yeast may have been present in the culture medium used; however, the latter may not have been ideal for cultivating such microorganisms. The presence of mold in the silages may be associated with the deleterious effects of oxygen, especially in the upper portion of the silo due to the difficulty of compacting this region during ensiling (Borreani et al., 2018), reflecting the high PDM levels. This result contributed to the low DMR levels in murumuru meal silages.
Although there was an increase in the N-NH3 content with murumuru meal inclusion, the highest content observed was still below the range considered desirable (<12%) for quality silage (Lopes and Evangelista, 2010). However, our results showed that the proteolysis that occurred during fermentation was not enough to alter CP levels, which varied below the 1.5% level among silages. That indicates no excessive protein degradation at the levels studied.
Lactic acid content observed in the silages indicate that fermentation occurred adequately as it was mainly responsible for the reduction in pH during ensiling and for maintaining acidification of the mass until the silo was opened. This response was evidenced by the pH values observed at this time, as well as by their similarity irrespective of murumuru meal inclusion. Reduction in acetic acid concentration with the addition of murumuru meal was probably a limiting factor in inhibiting mold growth in elephant grass as this acid has antifungal properties. The LA:AA ratio and total organic acid concentration reflected the aforementioned acid concentrations.
Reduction of aerobic stability of the silages with inclusion of murumuru meal occurred due to increase in NFC with meal addition (Table 4). The NFC are substrates used by spoilage microorganisms in the silage. The high substrate input evidenced by NFC content, high mold count, and low acetic acid concentration results in less stable silages when the additives are included (McDonald et al., 1991).
Increase in DM content of the silage with inclusion of murumuru meal was caused by high DM content of the additive, thus, demonstrating its potential as an absorbent additive for grass silage. The similarity in OM and ash concentrations among silages was probably caused by the similarity of these constituents in the meal and elephant grass.
Reduction in NDF, ADF, HEM, and CEL content with inclusion of murumuru meal in grass silage was probably caused by the lower content of these components in murumuru meal than in elephant grass. By adding murumuru meal to elephant grass silage, the concentrations of these components decreased proportionally. Despite reduction of NDF, ADF, HCEL, and CEL parameters in elephant grass silage with the inclusion of murumuru meal, lignin content increased. Lignin is a component that can interfere with feed digestibility. Its increase was anticipated as murumuru meal has higher lignin content (13.7%) than that of elephant grass (3.7%). High lignin levels may compromise the use of food by ruminal microorganisms and affect DM digestibility as they make part of unavailable cell wall components, thus resulting in fiber degradation (Wilson and Hatfield, 1997).
Increase in the NFC content of the silages with inclusion of murumuru meal was probably caused by high NFC content in the meal. Non-fibrous carbohydrates are considered mostly soluble and highly digestible, thereby increasing the nutritional value of the feed. In the case of silages, this increase in nutritional value depends on the microorganisms (homofermentative or heterofermentative), and products such as water, heat, carbon dioxide, lactic acid, alcohol, and volatile fatty acid generated (Muck, 2010).
In conclusion, including up to 28% murumuru meal in elephant grass silage improves the chemical composition of the silage, but it makes it more susceptible to deterioration when exposed to oxygen. Based on these results, we recommend to offer elephant grass-murumuru meal silage to the animals shortly after it is removed from the silo to avoid significant losses from aerobic deterioration.