Introduction
Coffee farming generates a large amount of waste material, including hulls resulting from the dry processing required to obtain coffee beans. This waste has potential to be included in ruminant diets, as shown in the studies reported by Rocha et al. (2006), and Souza et al. (2006). It is noteworthy that this material is readily available at the processing sites, unlike many agricultural waste materials, which remain in the field, thus increasing collection and transport costs (Figueiredo et al., 2008).
Anti-nutritional factors such as caffeine, polyphenols, and tannins present in coffee hulls may negatively affect the intake of this feedstuff (Vargas et al., 1982). Another limitation for including high levels of this waste in diets is the excessive concentration of cell wall components, mostly cellulose, hemicellulose, and lignin. Valadares Filho et al. (2010) reported 65.53 and 50.11%, neutral (NDF) and acid (ADF) detergent fiber, respectively, on a dry matter (DM) basis.
Several alkalis are used in fibrous roughages to promote partial solubilization of the cell wall components and, consequently, increase nutrient utilization. In this regard, the use of calcium oxide (CaO) stands out as the safest low-cost alkaline treatment compared with other alkalis. Research has shown the effectiveness of CaO at the level of 50 g kg‒1 DM for the treatment of corn and wheat straw in anaerobic environment (Sewell et al., 2009; Russell et al., 2011). The reaction of CaO with water is exothermal and the heat is retained inside a closed container, which might influence the substrate, improving its nutritional quality.
Despite the available volume of coffee hulls and previous research on its use in fresh form (Rocha et al., 2006; Souza et al., 2006), further research is needed to treat coffee hulls, with CaO. Therefore, the present study aimed to evaluate the chemical composition and rumen degradation kinetics of DM and NDF of coffee hulls treated with CaO under aerobic and anaerobic conditions.
Materials and methods
Ethical considerations
The experimental procedures were undertaken according to the norms of the Ethics Committee in Livestock Research of the Universidade Federal de Viçosa (UFV-CEUAP- UFV, approval no. 58/2014).
Location and treatment of hulls
The experiment was carried out in Campo Agropecuário and in Laboratório de Forragicultura of Departamento de Zootecnia at Universidade Federal de Viçosa (UFV), Viçosa campus.
Treatments were arranged in a 2 × 2 factorial design consisting of two CaO levels (0 and 5%, on a dry matter basis) and two environment conditions (aerobiosis and anaerobiosis), in a completely randomized design with four replicates, totaling sixteen experimental units.
Coffee hulls were obtained after the grain was dry-processed. The material was homogenized, and a sample was collected to estimate DM prior to application of treatments using the Karl Fischer method (Bruttel and Schlink, 2006) in a 870 KF Tritino Plus KF titrator (Metrohm, São Paulo, SP, Brazil).
After assessing for DM content, the moisture of coffee hulls was adjusted to 50% by adding water (Shreck, 2013). For the 0% CaO treatment, 3 kg of coffee hulls were added to 0.50 × 0.70 m transparent polyethylene bags (Cepel, São Paulo, SP, Brazil). For the 5% CaO treatment, powdered CaO was added to 3 kg of coffee hulls and the mixture was homogenized. To obtain the aerobic environment, bags were kept opened during the entire experimental period, whereas anaerobiosis was obtained with an Eco vacum 1040 vacuum sealer (Orved, Musil di Piave, VE, Italy). The experimental units were stored in a protected location at room temperature for seven days (Shreck, 2013).
Microbiological and chemical analysis
Before the moisture adjustment, a sample of coffee hulls was collected to analyze for chemical composition (following Detmann et al.,2012) and for microbial counts, i.e., molds and yeasts (Table 1).
Table 1 Chemical composition and mold-yeast count of fresh coffee hulls.
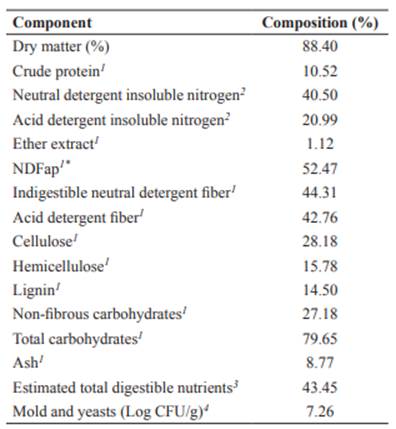
1Percentage of dry matter. 2 Percentage of total nitrogen. 3Estimated according to the NRC (2001). 4 CFU, colony-forming unit. *neutral detergent fiber corrected for ash and protein.
After seven days, a 25 g sample of the material was taken to quantify molds and yeasts populations, as described by Silva et al. (1997). The culture of microorganisms was carried out in sterile Petri plate in Potato Dextrose Agar medium (Kasvi, São José dos Pinhais, PR, Brazil) added with 1.5% tartaric acid 10%, using the Pour-plate technique. Plates showing between 30 and 300 colony-forming units (CFU) were considered suitable for counting, and the results were converted to log base (Log10 CFU). The second sample was weighed and dried in a forced-air oven (Fabbe-Primar, São Paulo, SP, Brazil) at 55 ºC for 72 h. Subsequently, the hulls were ground in a knife mill (Tecnal, Piracicaba, SP, Brazil) through 1- and 2-mm sieve. The concentration of DM, crude protein (CP), neutral detergent fiber corrected for ash and protein (NDFap), cellulose (CEL), hemicellulose (HEM), and lignin (LIG) was analyzed according to Detmann et al. (2012).
Experimental procedures, dry matter and neutral detergent fiber degradability
For the in situ degradability trial, samples of approximately 5.0 g (2 mm particles) were weighed and stored in previously weighed nylon bags (17 × 10 cm) with a pore size of 40 µm. Four bags from each treatment, per incubation time, were incubated in two rumen-fistulated male cattle (450 kg body weight) at the following incubation times: 3, 6, 9, 12, 24, 48, 72, 96, and 120 h. Bags were placed in reverse order, i.e., from the longest to the shortest incubation time, and removed simultaneously to be washed with the bags corresponding to time zero, which were not incubated. During the experimental period the animals received a diet containing 60% elephant grass (Pennisetum purpureum), 20% coffee hulls, and 20% concentrate, on a DM basis. After removing from the rumen, the bags were washed in running water, dried for 72 h in a ventilated oven at 55 ºC, and then weighed again. Subsequently, the bags were opened, and the residue was analyzed for the DM and NDF concentration, as described by Detmann et al. (2012).
The following equation, proposed by Ørskov and McDonald (1979), was used for the estimation of DM degradation kinetic parameters: Dt = a + b × (1 ‒ e‒ct), in which Dt is the fraction degraded at time t (%); a is the water-soluble fraction (%); b is the potentially degradable insoluble fraction (%); c is the degradation rate of fraction b (h‒1); and t is the incubation time (h‒1). Effective degradability (ED) was calculated through as: ED = a + (b × c)/(c + k), in which k is the rumen passage rate of the particles, considering rates of 2 and 5% (Ørskov and McDonald, 1979).
For NDF degradation, the model proposed by Waldo et al. (1972) was used, according to the formula: Ŷ = b × e(−ct) + I, in which Ŷ is the undegradable residue at time t (%); b is the potentially degradable fraction of the fiber (%); c is the degradation rate of b (h‒1); t is the incubation time (h‒1); and I is the insoluble, undegradable fraction (%).
Statistical analysis
Data were analyzed as a 2 × 2 factorial arrangement in a completely randomized design using the MIXED procedure of SAS® software, version 9.3 (SAS Institute, Inc., Cary, NC, USA; 2011). The degradation curve of DM and NDF for each treatment was adjusted by the respective models using the Marquardt Regression procedure to generate the parameter estimates. Homogeneity of variances among treatments was assumed and degrees of freedom were estimated using the Kenward-Roger method. Data were subjected to analysis of variance, and means compared by the F test, with 0.05 as the critical probability level for a type I error.
Results
All chemical composition variables and mold and yeast counts were affected (p<0.05) by CaO × EC (Table 2). Treated coffee hulls showed higher DM (p<0.05) compared with the untreated ones in both aerobic and anaerobic environments (Table 2), and higher temperatures were recorded in this material after treatment with CaO (data not shown). Untreated hulls under anaerobic condition showed higher DM than those in the aerobic condition, while CaO- treated hulls did not show differences between the environment conditions for DM concentration. Treating hulls with CaO promoted a reduction (p<0.05) in CP, NDFap, HEM and LIG fractions and populations of molds and yeasts in both the aerobic and anaerobic conditions (Table 2). Under anaerobic condition, there was a reduction (p<0.05) in CP, NDFap, CEL and LIG contents and populations of molds and yeasts of the untreated and treated hulls when compared with the aerobic environment. Hemicellulose had a greater reduction (p<0.05) under aerobiosis in both treated and untreated hulls (Table 2).
For DM degradation kinetic parameters, except for fraction b, potential degradability (PD) and effective degradability (ED) were affected (p<0.05) by CaO × EC (Table 3). Coffee hulls treated with CaO showed higher (p<0.05) soluble fraction (a) values in comparison with the untreated hulls under both environments (Table 3). The environments did not affect (p>0.05) the potentially degradable fraction b, with a mean of 32.35%. The use of CaO increased (p<0.05) this fraction in 3.26 percentage points when compared with its absence (Table 3). No difference was observed (p>0.05) between degradation rates (c) of fraction b with the use of CaO in both environments compared with untreated hulls. Higher degradation rate (p<0.05) was obtained for untreated hulls in anaerobic than in the aerobic environment, with respective mean values of 0.05 and 0.03%/h (Table 3). The PD result demonstrated the effectiveness of the alkali used in the treatment of hulls (Table 3). The CaO provided an increase (p<0.05) in PD compared with the untreated hulls in both environments. Higher PD (p<0.05) was also found for treated hulls under anaerobiosis compared with the aerobic condition, with values of 67.58 and 65.15%, respectively (Table 3).
Figure 1 shows degradation curves adjusted by the model proposed for DM. Higher degradability was seen in hulls treated with CaO under anaerobiosis. After 72 h incubation, DM degradability tended to remain constant in all treatments.
No effect (p>0.05) of CaO × EC was detected on the estimated degradation parameters of NDF. The potentially degradable fraction (b) was affected (p<0.05) by the environment and CaO. The CaO increased this fraction (p<0.05) by 4.08 percentage points when compared with the untreated hulls (Table 4). Undegradable fraction I was affected (p<0.05) by the environment and CaO. The CaO led to a reduction (p<0.05) of this fraction, with values of 66.30 and 61.93% for the untreated and treated hulls, respectively (Table 4). Hulls under anaerobiosis showed an increase (p<0.05) in fraction b and a reduction (p<0.05) of undegradable fraction I as compared with the aerobic environment (Table 4). No effect was observed (p>0.05) for the use of CaO or the type of environment on degradation rates of fraction b, indicating that neither CaO nor the environment interferes with that variable (Table 4).
Table 2 Chemical composition of coffee hulls treated or untreated with calcium oxide in aerobic or anaerobic conditions.
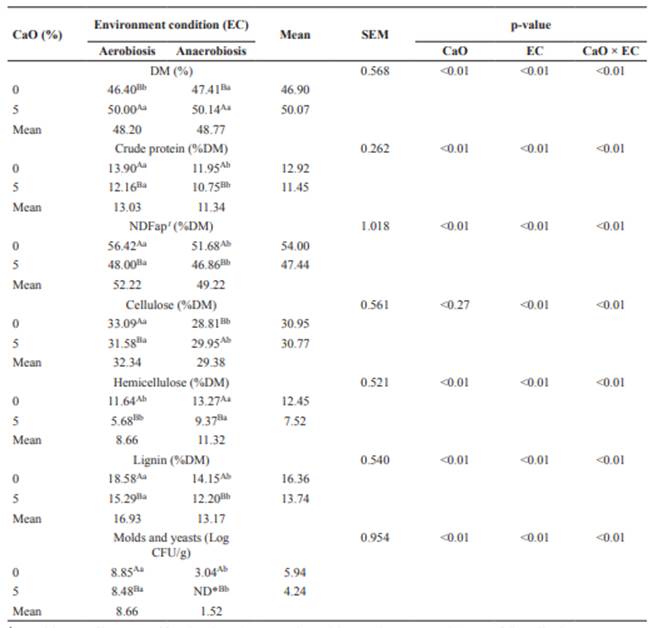
1Neutral detergent fiber corrected for ash and protein. ND, not detected (lower value 1 Log CFU/g). Means followed by the same uppercase superscript letters (A, B) within columns and lowercase superscript letters (a, b) within rows do not differ (p>0.05) by the F test.
Table 3 Degradation parameters and in situ degradability of dry matter of coffee hulls treated or untreated with calcium oxide in aerobic or anaerobic conditions.
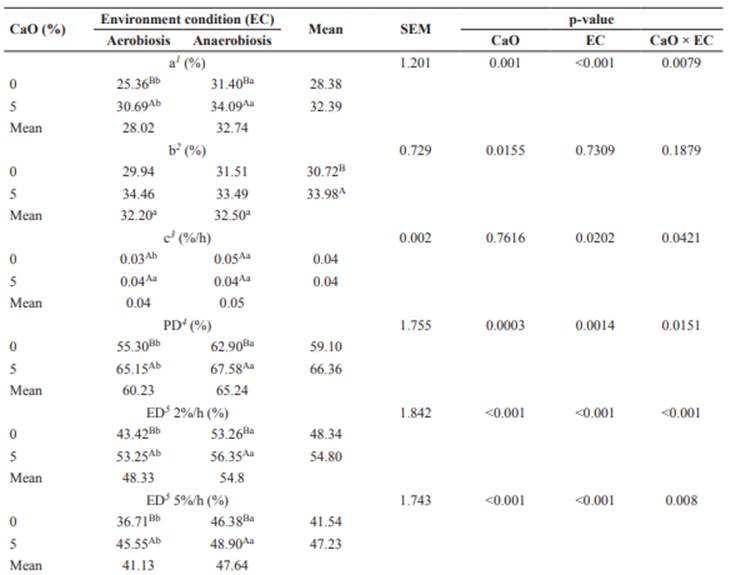
1 Soluble fraction. 2 Potentially degradable fraction. 3 Degradation rate of fraction b. 4 Potential degradability (fraction a + b). 5 Effective degradability. Means followed by the same uppercase superscript letters (A, B) within columns and lowercase superscript letters (a, b) within rows do not differ (p>0.05) by the F test.
Figure 2 shows the degradation curves adjusted by the model proposed for NDF. The treatments with CaO in anaerobic conditions led to a greater NDF degradation, while untreated hulls under aerobiosis showed the lowest degradability for this component. After 72 h, fiber degradation remained constant in all treatments.
Discussion
The high DM contents observed in the treated coffee hulls were attributed to the high DM concentration of CaO (approximately 96%). Additionally, quicklime reduced water activity in the treated hulls, thus reducing growth of molds and yeasts and nutrient losses (Table 2).
The lowest CP content (10.75%) was recorded when hulls were treated with CaO under anaerobiosis, which is close to that observed for fresh hulls before moisture adjustment (Table 1). According to Sampaio et al. (2010), 10% CP is a level that optimizes the use of energy substrates from roughages and provides conditions for the rumen microorganisms to have full capacity to degrade fibrous carbohydrates. Higher CP values observed in the other treatments can be attributed to the contribution of microbial protein present in the material; in this regard, reduced microbial activity (molds and yeasts) was observed in hulls treated under anaerobiosis (Table 2).
Reduction of NDFap, HEM, and LIG when using CaO was probably due to hydrolytic action ofthealkalionthechemicalbondsbetweenfibrous carbohydrates and lignin present in the cell wall. During hydrolysis, lime reacts with water from the residue and the formation of a strong base occurs, calcium hydroxide (Ca(OH)2), causing hydrogen bridge bonds to break, resulting in partial solubilization of cell wall constituents. Concentration of CEL was reduced only under anaerobiosis (Table 2). According to Jackson (1977), when treated with alkaline agents, CEL is not dissolved, but expands, reducing the resistance of intermolecular bonds of hydrogen bridges. Jackson (1977) also emphasized that the proportion of CEL in the treated waste is larger than in its untreated version. In the present experiment, this response was observed only under anaerobiosis, in which material showed a CEL content of 29.95 versus 28.81% for the untreated hulls (Table 2). This finding agrees with Chaudhry (2000), who observed an increase in CEL content of wheat straw treated with CaO under anaerobiosis of 45.10 and 52.80% in the treated and untreated straw, respectively.
The reduction of HEM is possibly related to degradation, since aerobiosis favored the growth of molds and yeasts (Table 2). McDonald et al. (1991) stated thatthe substrate used for respiration depends on the type of microorganisms and that yeasts consume only soluble compounds (sugars and fermentation productions), whereas molds degrade a wide range of nutrients, including structural carbohydrates and lignin.
As for DM degradation parameters, it is evident that the increase of fraction a was due to an action of the alkaline agent through a break of bonds between lignin and cell wall carbohydrates, or even hydrolysis of cell wall polysaccharides, resulting in the release of soluble sugars.
Table 4 Degradation parameters of neutral detergent fiber in coffee hulls treated or untreated with calcium oxide in aerobic or anaerobic conditions.
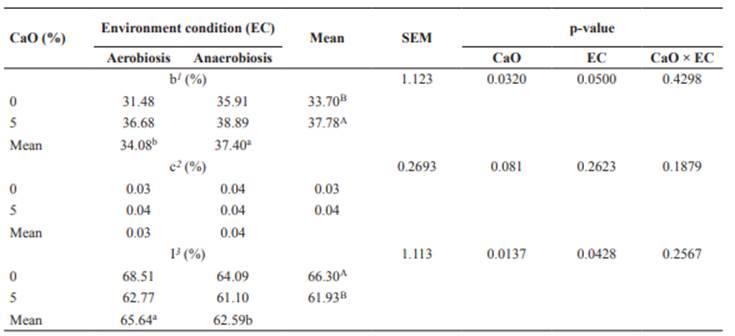
1 Potentially degradable fraction. 2 Degradation rate of fraction b. 3 Undegradable fraction. Means followed by the same uppercase superscript letters (A, B) within columns and lowercase superscript letters (a, b) within rows do not differ (p>0.05) by the F test.
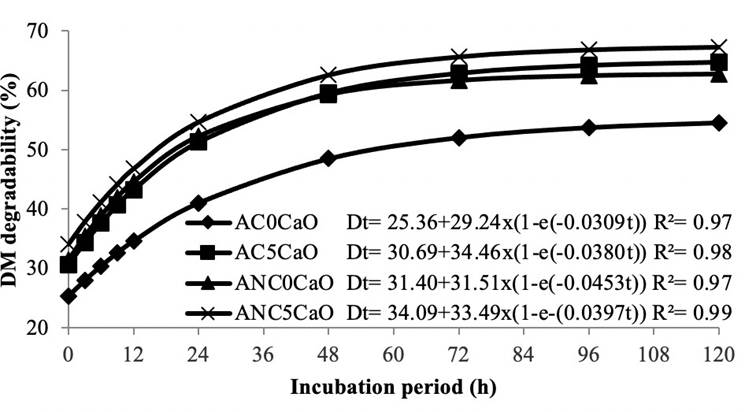
Figure 1 Curves and equations of dry matter degradability in coffee hulls treated or untreated with calcium oxide under aerobic or anaerobic conditions. AC0CaO: aerobic condition, 0% CaO; AC5CaO: aerobic condition, 5% CaO; ANC0CaO: anaerobic condition, 0% CaO; ANC5CaO: anaerobic condition, 5% CaO.
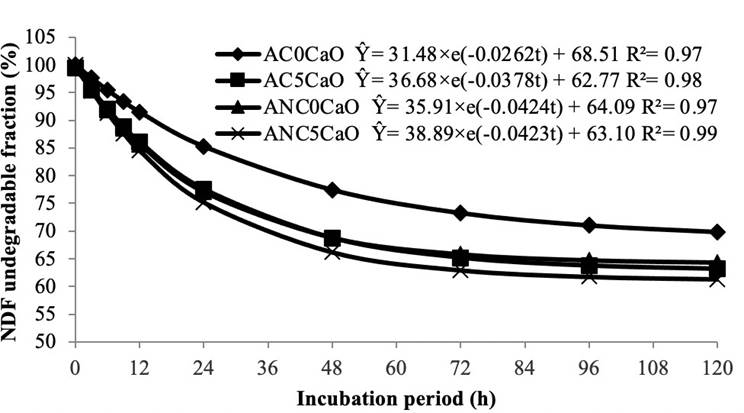
Figure 2 Curves and equations for the undegradable fraction of neutral detergent fiber in coffee hulls treated or untreated with calcium oxide under aerobic or anaerobic conditions. AC0CaO: aerobic condition, 0% CaO; AC5CaO: aerobic condition, 5% CaO; ANC0CaO: anaerobic condition, 0% CaO; ANC5CaO: anaerobic condition, 5% CaO.
This leads to an increase in water-soluble compounds and enzyme-substrate interaction (Wang et al., 2012). Greater participation of fraction a (p<0.05) under anaerobiosis was observed for treated and untreated hulls compared to the aerobic condition (Table 3). The use of soluble components by microorganisms in the hulls under aerobiosis likely caused the reduction of this fraction (Table 2).
Chaudhry (2000) evaluated in situ degradability of wheat straw treated with CaO (160 g kg‒1 in the DM) in an anaerobic environment and found increased soluble fraction, recording 10.8 and 27.9% for untreated and treated straw, respectively. The same pattern was reported by Monção et al. (2014), who treated banana peels with CaO (40 g kg‒1 fresh matter) obtaining 46.26 and 41.65% in situ degradability for the treated and untreated peels respectively. The CaO increased fraction b (Table 3). When CaO was used (30 g kg‒1 fresh matter) for sugarcane hydrolysis, Romão et al. (2013) observed 11.4 percentage points increase in fraction b in comparison with fresh sugarcane. Accordingly, CaO increases the digestible fractions when used for the treatment of fibrous roughages.
A high PD value was recorded under anaerobiosis (Table 3). The reaction temperature might have had a positive influence on this outcome, since 3 h after the hulls were treated with CaO, higher temperatures were recorded (43.37 ºC) compared with the aerobic environment (37.52 ºC). This higher temperature may reflect a better environment within the closed container. Because heat from exothermal reaction of the chemical treatment is retained, these factors may interact with the substrate, therefore improving the results. Chaudhry (2000) treated wheat straw with CaO (160 g kg‒1 DM) under anaerobiosis, observing a 92.3% PD of DM in the treated, and 54.1% in the untreated straw, which represents 38.2 percentage points increase in the treated material. In the present study, this increase in PD was 4.68 percentage points for the treated hulls under anaerobiosis compared with the untreated material in the same environment (Table 3). The difference in PD increase is probably due to the CaO dose used. Considering the dose of 160 g kg‒1 DM as high, Chaudhry (2000) suggested further studies for reducing the amount of CaO without affecting the alkali action on fiber components.
A similar response to that of PD was observed for ED at the different rates used (Table 3). The ED value (rates: 2 and 5%/h) for hulls treated with CaO under anaerobiosis in this study was higher than the ED of corn silage reported by Cavalcante et al. (2012) at the same respective passage rates. This demonstrates the potential usage of this residue in diets for different animal categories.
Regarding estimated NDF parameters, the increase of fraction b and reduction of fraction I when using CaO (Table 4) can be explained by the action of the alkaline agent in the cell wall components, reflecting in solubilization of part of the undegradable constituents (Balieiro Neto et al., 2007; Romão et al., 2013) thereby increasing fiber degradation (Chaudhry, 2000). As for the different environments, these fractions presented higher values in anaerobiosis. This effect may be due to the action of molds and yeasts, which grow rapidly in aerobiosis, consuming part of the nutrients present in the substrate (McDonald et al., 1991).
The use of CaO for the treatment of coffee hulls reduces the cell wall components and undegradable fiber fraction and increases PD and ED of DM. Anaerobiosis was the best environment for CaO-treated hulls. Anaerobiosis also promotes improvements in untreated hulls. Further studies are needed to evaluate its effects on intake and productive performance of ruminants.