Introduction
Agricultural activity in the mid-north area of the Mato Grosso state is characterized by the cultivation of soybeans (Glycine max L.) as the main crop followed by maize (Zea mays L.) in agricultural succession; and this is carried out through direct sowing system (DSS), i.e. without soil mobilization and with fertilization carried out on a yearly basis (Valadão, dos Santos, Júnior, Scapinelli, Deina & Bianchini, 2015). These practices are adopted in large rural properties, to provide greater operational efficiency, flexibility during the sowing period and, mainly, anticipation in maize cultivation.
In spite of the productive emphasis of Mato Grosso (CONAB, 2017), this system is put to the test regarding agronomic use-efficiency of fertilizers, because it causes fertility gradients in superficial soil layers (Valadão et al., 2015), as well as by the use of fixed amounts of N, P and K by producers who fear crop yield reduction (de Jesus-Lacerda, Resende, Neto, Hickmann & da Conceição, 2015).
There are also reports of superficial soil compaction due to soybean harvesting and maize sowing operations that are carried out during rainy periods, usually in late January and early February. These procedures tend to reduce soil macroporosity to levels below 10%, which are considered critical because they reduce water infiltration and availability for plants (Rossetti & Centurion, 2013).
An increasing concern for natural resources protection encourages the study of productive chains, aiming at more efficient cultivation and management of agricultural activities. Some of the challenges for grain cultivation in the Brazilian Cerrado is the improvement of the use efficiency of fertilizers integrated into soils and replacing chemical nitrogen and calcium carbonate, which are inputs with high greenhouse gas emitting (GGE) capacity (de Freitas-Silva, Moitinho, Teixeira, Pereira & Júnior, 2014; Guareschi, Perin & Gazolla, 2013).
In order to cope with this environmental need, the Ministry of Agriculture launched in 2010 the Low Carbon Agricultural Plan, known as the "ABC Plan", to facilitate capacity building, improve technical assistance and provide special credit lines for farmers adopting low-carbon agricultural practices, biological nitrogen fixation, restoration of degraded pastures, commercial forest plantations and treatment of animal waste (Amaral, Cordeiro & Galerani, 2011).
In this perspective, several studies report the efficiency in the use of animal waste in agriculture, and among these we find liquid swine manure (LSM), applied as a source of nutrients for soybeans, maize, beans and wheat (Peng, Zhu, Xie, Darboux & Holden, 2016; Sartor, Assmann, Assmann, Bigolin, Miyazawa & Carvalho, 2012). In addition to representing an ecological form of disposal, the use of LSM as a biofertilizer becomes feasible as it reduces production costs, mainly by reducing the use of mineral fertilizers, which in some cases represents ca. 40 % of the total crop cost. Studies have recommended the application of 40 m3.ha-1 of LSM together with urea for maize to obtain 10.03 Mg.ha-1 of grain yield. Sartor et al. (2012), verified better responses in soybean when applying 60 m3.ha-1 of LSM. However, few studies report the effect of LSM management on physical and chemical soil attributes, especially in commercial grain production areas in Mato Grosso state that uses DSS.
In view of this lack of information and considering the hypothesis that LSM is an adequate option to improve soil structure and fertility in the mid-north region of the Mato Grosso state, the aim of this study was to evaluate the physical and chemical quality of the soil and the productive potential of soybean and maize under different doses of LSM as fertilizer, comparing them with the use of the mineral NPK fertilizer.
Materials and methods
This study was conducted in commercial soybean and maize production areas located in the municipality of Vera, mid-northern area of the Mato Grosso state with the following geographical coordinates: 12°18' 21" S and 55°19' 01" W at 383 m.a.s.l. According to the climatic classification of Kõppen-Geiger, the region is categorized as Aw, i.e. has a tropical wet savannah type climate. Annual precipitations and temperatures range from 1,700 to 2,200 mm and 24 to 34 °C, respectively.
Soils are classified as Dystrophic Red-Yellow Latosols (LVAd) by the Brazilian Soil Science Society, or Udox by the American Commission Soil Survey Staff, with a very clayey texture, values of 601 g.kg-1 of clay, 94 g.kg-1 of silt and 305 g.kg-1 of sand in the 0-0.20 m layer. The area has a flat relief with 0 to 3% slope. Soil fertility in the 0-0.20 m layer is shown in Table 1.
Table 1 Chemical characterization of a Dystrophic Red-Yellow Latosol.1

1Chemical attributes include measurements of phosphorus, sulfur, zinc, copper, iron, manganese and boron (mg.dm-3), potassium, calcium and magnesium (cmol.dm-3), soil organic matter (g.kg-1) and pH
In the last 10 years the study area has been cultivated with soybean (Glycine max L.) as the main DSS crop and maize (Zea mays L.) is in second place, and therefore, we chose these species for our study.
We divided the area into experimental units (plots) of 5 m x 11 m (55 m2). The LSM used in the experiment was produced in a nearby location where manure is properly treated with biodigesters and later stored in mortars. Mean nutrient contents are described in Table 2.
Table 2 Macro and micronutrient contents in liquid swine manure.1

1Chemical attributes include measurements of nitrogen, phosphorus pentoxide, potassium oxide, calcium, magnesium, sulfur and organic matter (g.L-1), zinc, copper, iron, manganese and boron (mg L-1), pH and carbon/ nitrogen ratio
LSM doses were established following the criterion of total K2O supply, since the macronutrient showed the lowest amounts in the area. Thus, the calculations were based on the equation 1:
where: "x" is the amount of nutrients applied (kg.ha-1); "a" is the amount of liquid swine manure to be used (m3.ha-1); "b" is the concentration of the nutrient in the waste (kg.m-3); and "c" is the nutrient release efficiency for plants (SBCS, 1995).
Subsequently, the experiment was conducted in a randomized complete block design with 5 treatments and 4 replicates. Treatments were established according to LSM rates applied in soybean and maize, as follows: T1 = 0 m3.ha-1, T2 = 40 m3.ha-1, T3 = 80 m3.ha-1 and T4 = 160 m3.ha-1. Treatment T5 for soybean used the same fertilization used in commercial production areas, i.e. 200 kg.ha-1 of the mineral formulation NPK 02-20-15 in the sowing furrow, plus 280 kg.ha-1 of 0-20-20, 35 days after planting. For maize, T5 was fertilization with 300 kg.ha-1 of the mineral formulation 0-20-20, 35 days after planting.
For soybean (TMG132RR), spacing of 0.45 m between rows with 16 plants per meter was used, while for maize (AG7088), spacing of 0.45 m between rows and 4 plants per meter was employed. The management practices of both crops followed the technical cultivation recommendations carried out homogeneously throughout the experimental area.
After soybean harvest, soil composite samples (resulting from 6 simple samples of deformed structure) were extracted from each experimental unit from the following layers: 0.00-0.10 m and 0.10-0.20 m. These samples were used to determine available levels of phosphorus (P) and exchangeable potassium (K), copper (Cu), iron (Fe), manganese (Mn) and zinc (Zn) (Embrapa, 2011), sulfur (S) and boron (B) (van Raij et al., 2001). From these, total organic carbon (TOC) and total carbon stock (TCS) was also established (Embrapa, 2011).
Physical attributes of soil were analyzed in samples collected after maize harvest. For this, 4 trenches were opened in each experimental unit from which samples of preserved soil structure were extracted from the following layers: 0.00-0.05 m, 0.05-0.10 m, and 0.10-0.20 m, using metallic volumetric rings of 100 cm3. Afterwards, samples were taken to the laboratory where they were saturated and matric potential of -100 hPa in sandbox were established. After stabilization, these were weighed and soil resistance to penetration (PR) was measured using an electronic penetrometer bench, as recommended by Tormena, da Silva and Libardi (1998). Samples were then dried in the oven at 105 °C for 48 hours and reweighed to establish macroporosity (MA), microporosity (MI), total porosity (TP) and apparent bulk density (BD) (Embrapa, 2011).
Furthermore, after maize harvest and to evaluate the stability of aggregates in water, 8 soil monoliths were collected in each experimental unit, four in the layer of 0.00-0.10 m and the other four in the 0.10-0.20 m layer. From these monoliths three samples of aggregates of 30 g with a size between 8 and 4 mm were removed, two for aggregation and one for humidity measurements. Then, samples were moistened through capillarity on a filter paper and placed on a set of sieves with meshes of the following sizes: 4.0, 2.0, 1.0, 0.5, 0.25 and 0.125 mm, to separate aggregates per size classes. Sieve assembly was then submerged in a Yoder tank and subjected to vertical shaking for 15 min at 30 oscillations per minute. Weighted mean diameter (WMD) and geometric mean diameter (GMD) of aggregates were adopted as aggregation indices (Kemper & Rosenau, 1986).
Morphological soybean and maize attributes were established using plants collected in three central lines of each experimental unit, scraping one meter of the borders. Thus, a collection area of 12.15 m2 was considered useful. Variables registered for soybean were: number of leaves (NL) number of pods per plant (NP), one-hundred grain mass (GM), and grain yield (GY), with adjusted values for humidity conditions to 13%. Moreover, variables for maize were: plant height (PH), ear insertion height (IH), stalk diameter (SD), GM and GY, with moisture values also adjusted to 13%.
All attributes analyzed were analyzed with the Shapiro-Wilk normality test at p < .05 of probability; moreover, in the presence of outliers, those with higher values than the average as well as four standard deviations were removed, respecting the 10 % limit of total observations (Castione, Souza, Silva, Campos & da Cunha, 2015). Subsequently, normal data were submitted to analysis of variance with an F test (p < .05).
Furthermore, when analyzing the physical and chemical attributes of soil, a subdivided plot scheme was used, with the main factor being fertilization (LSM doses and mineral NPK fertilizer), and the secondary factor was soil layers (0.00-0.10 and 0.10-0.20 m for chemical attributes, and 0.00-0.05, 0.05-0.10 and 0.10 0.20 m for physical attributes). When F was significant, the t-Test (p < .05) was used for comparison of means.
In the morphological attributes analysis of the plants, only the fertilization factor was used. When F was significant, polynomial regression analysis was performed using the highest coefficient of determination (R 2 ), the lowest sum of squares of the residues, and the significance of the parameters of the equation (p < .05).
Results
The best total porosity (TP) conditions of were observed in treatments with 40 and 80 m3.ha-1 of LSM and fertilization with NPK, with respective averages of 0.42, 0.44 and 0.44 m3.m-3 (Figure 1). The results of MA reaffirm the effect of fertilizations on porosity. Only the control treatment was statistically different, showing the lowest volume of 0.07 m3.m-3 (Figure 1). However, only T2 had a MA volume above 0.10 m3.m-3.
In relation to MI, means did not differ between treatments T1, T3, T4 and T5, with amplitude in micropore volume between 0.31 and 0.34 cm3. cm-3. For BD there was no significant difference as a function of the fertilizations and depths evaluated. Thus, the overall mean of1.13 Mg.m-3 may represent the condition of the experimental station in its entirety.
Considering the overall mean, resistance to penetration (PR) was higher than 2 MPa, among the treatments proposed, and T1 that did not receive fertilization had the highest mean (3.13 MPa); moreover, T3 and T4 treatments were not statistically different.
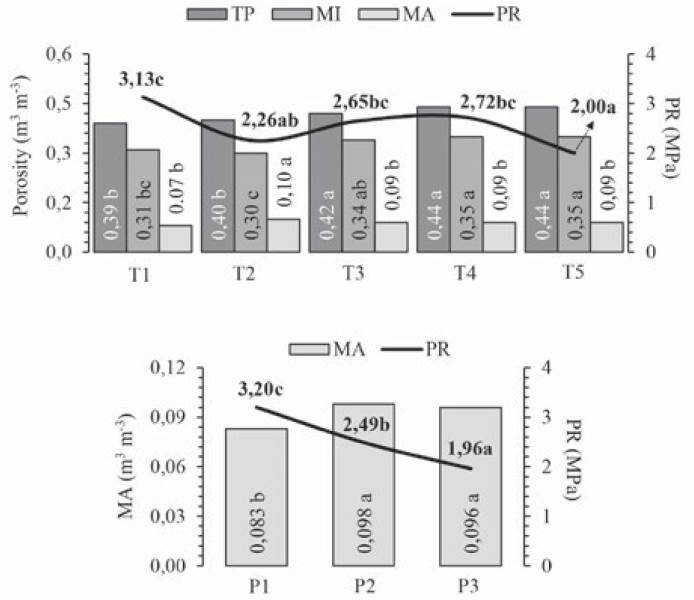
Figure 1 Mean values of soil physical attributes as a function of soil depth and fertilization with liquid swine manure and mineral fertilizer.
MA and PR were different with depth. The best soil physical condition was observed in the 0.10-0.20 m layer (P3), with averages of MA and PR of 0.098 m3.m-3 and 1.96 MPa, respectively (Figure 1).
However, there was no significant difference in the stability of aggregates (WMD and GMD) after fertilization with LSM and NPK in the two layers evaluated.
Furthermore, low coefficients of variation (<15 %) were observed for most of the physical attributes of soil evaluated. Only PR and MA presented mean variability, 15 % < CV < 35%.
Regarding soil fertility characterization, the criteria for Brazilian Cerrado soils were used. There was a significant effect of fertilization on soil P, K and S contents. K and S had adequate levels in all treatments and depths evaluated. The highest concentration of exchangeable K was observed in the fertilization with 80 m3.ha-1 of LSM (T3), but did not differ statistically from treatments T2 and T4 (Figure 2). Highest concentrations of S were observed in treatments T1 and T3, with averages of 10.72 and 7.12 mg.dm-3, respectively. As for depth, the highest concentration of S was observed in the 0.10-0.20 m layer (Figure 2).
Furthermore, available P levels in treatments T1, T2, T4 and T5 (Figure 2) were lower than the one obtained in the initial fertility analysis that was performed before the experiment was set up (Table 1).
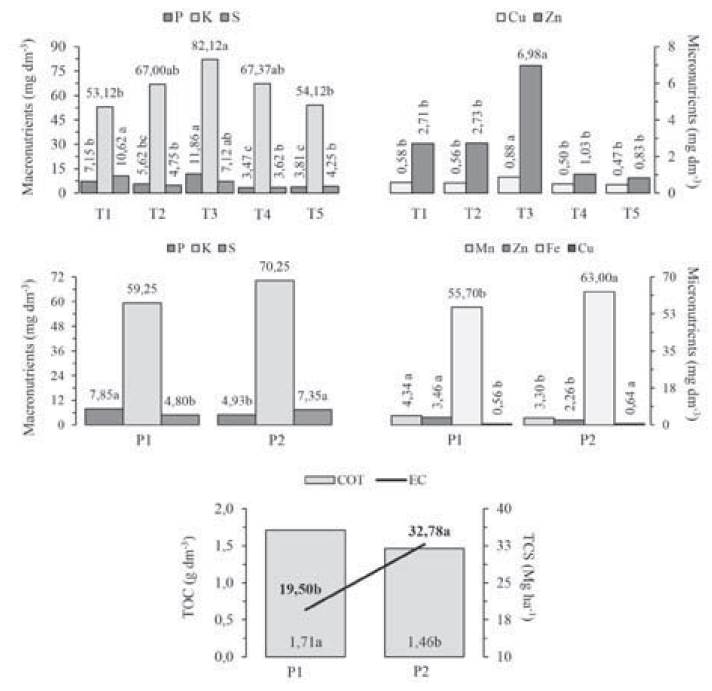
Figure 2 Mean values of soil chemical attributes as a function of fertilization with liquid swine manure and mineral fertilizer
Only T3 with fertilization of 80 m3.ha-1 of LSM provided increases in P concentration, differing statistically from the others. In this type of soil P was classified as high (> 8.0 mg.dm 3) only in T3, adequate (5.5 < P < 8.0 mg.dm-3) in T1 and T2 and P1 depth, and low (P < 5.5 mg.dm-3) in T4, T5 and P2. As for depth, the availability of P was higher in the topsoil with 7.85 mg.dm-3.
There was significant interaction between fertilization and depth factors for available P contents. Moreover, the presence of the highest availability of P for all evaluated treatments was established in the 0.00-0.10 m layer (Table 3).
Table 3 Deployment of the levels of phosphorus as a function of depth and fertilization with liquid swine manure and mineral fertilizer.

1Means followed by the same letter, uppercase in the columns and lowercase in the rows, do not differ statistically from each other by the f-Test at p < .05 probability.
Fertilization with 80 m3.ha-1 of liquid swine manure (T3) provided the highest P content in both layers evaluated with averages of 15.25 and 8.47 mg.dm-3 respectively. Treatments T4 and T5 showed the lowest P levels in the two layers evaluated, however, not statistically differing from T2.
In addition, fertilizers altered the concentrations of some micronutrients in the soil with increases in the exchangeable levels of Zn and Cu in the dose of 80 m3.ha-1 of LSM were observed (Figure 2). There was no significant difference for the other treatments, however, all were classified as having high availability of Zn (> 2.2 mg.dm-3) and low for Cu (0.40-0.79 mg.dm-3). Only T3 presented medium availability of Cu (0.8-1.2 mg.dm-3).
The highest concentration of Mn and Zn in the surface layer and Fe in the sub-surface soil layer was also observed (Figure 2). However, the Mn and Fe contents were not influenced by fertilization.
There was a significant effect of LSM levels on PH, GM, NL and GY of soybean, as well as on PH, IH, SD, GM and GY of maize. Low coefficients of variation were found for most of the evaluated attributes, except for NP of soybean, which was > 35 %, and did not differ statistically among treatments.
Nonetheless, NL in soybean decreased with the application of low doses of LSM of up to 22.62 m3.ha-1, however, it showed an increasing response curve up to the dose of 110.53 m3.ha-1, which provided the maximum number of 26 leaves (Figure 3a). As for PH and GM of soybean, the best responses were 89.34 cm and 16.66 g, at the doses of 66.7 and 54.18 m3 ha-1, respectively.
The minimum and maximum points for NT were found in doses similar to those of minimum and maximum grain yield, 28.39 and 104.93 m3 ha-1, respectively. The minimum GY was 3.63 Mg ha-1 at a dose of 28.39 m3 ha-1 and a maximum of 4.75 Mg ha-1 with application of104.93 m3 ha-1 of LSM. Fertilizer with NPK mineral fertilizer indicated soybean GY of 3.22 Mg ha-1, with 0.99 Mg ha-1 lower than the control treatment (Figure 3a).
Morphological characteristics of IH and PH in maize were described by quadratic functions, which were positively influenced until doses of 69.00 and 76.66 m3.ha-1, respectively (Figure 3b). On average, they were 13.5 % higher than the values obtained by the treatment without LSM application, which were 1.35 m for IH and 2.52 m for PH. However, the highest SD value was 31.48 cm, and was obtained with application of 48.5 m3.ha-1 of LSM.
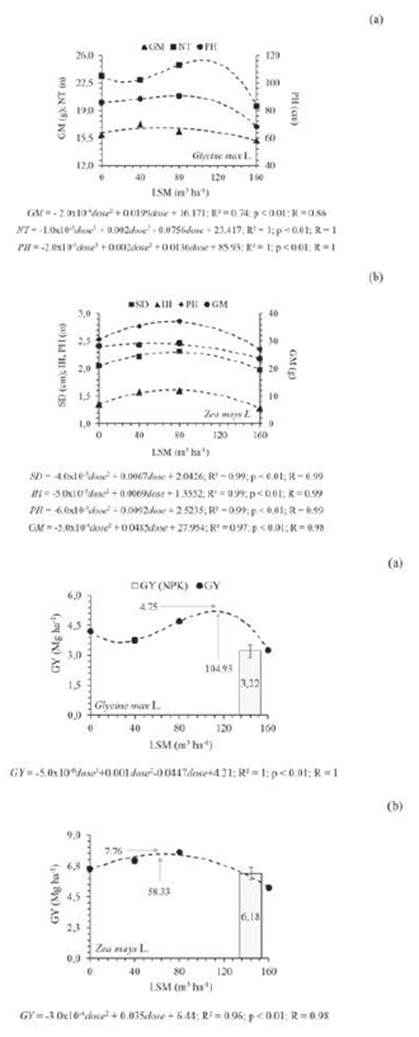
Figure 3 a) Morphological attributes, and b) soybean and maize grain yield as a function of fertilization with liquid swine manure (LSM).
As for maize GY application of LSM positively influenced the dose of 58.33 m3.ha-1, with a maximum of 7.76 Mg.ha-1 (Figure 3b). Fertilization with NPK in maize indicated higher GY than seen in the control and doses of LSM > 136 m3.ha-1.
Discussion
The TP averages increased as a function of the LSM rates applied to the soil. This increase can be justified by the improvement of the edaphic environment, due to the formation of "biopores" after plant root decomposition (Lima, Oliveira, Serafim, Curi & Evangelista, 2012). However, only T2 had an MA volume above 0.10 m3.m-3, below which plant root growth is impaired by aeration restrictions (Rossetti & Centurion, 2013). In general, average PR was above 2 MPa, and was considered restrictive to plant root growth by Tormena, da Silva and Libardi (1998). Thus, we can say that all treatments were affected by the DSS management system (Figure 1). The behavior of the MA and PR variables in the 0.05-0.10 m (P1) layer confirms soil compaction at critical DSS levels, with a reduction of1.3 % in macroporosity and 1.24 MPa in resistance to penetration, when compared to P3. PR and MA were the most sensitive attributes to DSS due to the average spatial variability, 15 % < CV < 35 %.
Based on the results of WMD and GMD after fertilization with LSM and NPK in two soil layers evaluated, it was assumed that soil changes tend to occur gradually over the years after application of LSM. Peng et al. (2016) verified the effect of applying LSM three years later on the stability of aggregates of an Argisol in a peanut cultivated area. Moreover, authors point out that such modification occurred due to erosion reduction, which is common in areas located in the subtropical regions of China.
It is assumed that the application of LSM in soybeans is an appropriate option for supplying K to plants, as well as their soil increase. Control and fertilization with NPK in the area showed the same means, which proved to be a soil with built in fertility. As for the results obtained for S regarding higher averages in the 0.10-0.20 m layer, it is known that SO4 2- moves freely in the arable soil layer when properly corrected and fertilized with P, which is common in areas of commercial soybean and maize cultivation in the northern region of the Mato Grosso state. Moreover, studies carried out by Camargo and Raij (1989) have shown that liming increases the negative charges of the soil exchange complex, which results in higher repulsion of the SO4 2- ions and, consequently, their displacement in the soil profile. This possibly occurred more intensely in the 0.00-0.10 m layer, although the soil CTC was not evaluated in this study.
Regarding the availability of P in depth, a higher concentration in the superficial layer of the soil with 7.85 mg.dm-3 was observed. Similar results were observed by Bezerra, Loss, Pereira and Perin (2015), evaluating the organic and inorganic fractions of P in DSS, crop-livestock integration and native vegetation of the Cerrados. The authors concluded that the highest P availability occurred in the soil surface layer for DSS due to the contribution of organic matter that is restricted to the 0.00-0.10 m layer.
Assessing the results obtained for micronutrients, contamination risks of clayey soils should be considered since the dose of 80 m3.ha-1 of LSM increased the level of Zn from 2.40 mg.dm-3 (Table 1) to 6.98 mg.dm-3 (Figure 2), and Cu from 0.50 mg.dm-3 to 0.88 mg.dm-3.
Nonetheless, micronutrients supply to foliar plants is recommended for clayey soils in two to four annual applications with zinc sulfate, borax, boric acid or FTE solutions. On the other hand, Rosolem and Boaretto (1989) indicated that fertilization with Zn and Cu can be done through the soil, however, this must be done together with corrective fertilization or basic fertilization; moreover, both should be carried out in the planting furrow, as high phosphorus concentration in the substrate decreases uptake of Zn and Cu by plants. Both liming and phosphate fertilization at high doses may decrease the availability of Zn to soybeans.
Although BD did not show significant differences between the evaluated layers, TOC was superior in the superficial layer of the soil with 1.71 g.dm-3. Furthermore, a higher amount of carbon stored in the 0.10-0.20 m layer was observed with 33 Mg.ha-1. Some studies also show that significant changes in CS occurs more intensely at depths below 0.20 m (Leite, Galvão, Holanda Neto, Araujo & Iwata, 2010).
As for soybean attributes, NL influence on PG can be described by the number of axillary floral buds, which allows a greater number of pods per plant, and, consequently, higher number of grains are produced. Although the number of grains per pod was not evaluated, it is assumed that with high LSM doses (> 80 m3.ha-1), there is a higher production of pods with 3 and 4 grains. Thus, competition for nutrients and photo-assimilates within the pods increases and, as a consequence, unit mass of the soybeans is reduced. This may explain the decrease in MC at doses above 54.18 m3.ha-1.
Similar results were obtained by Sartor et al. (2012), when they found a reduction of PG of soybean with doses of up to 22.25 m3.ha-1 of LSM. Subsequently, better results were obtained (2.86 Mg.ha-1) with the maximum tested dose of 60 m3.ha-1 of LSM.
Conclusion
Fertilization with liquid swine manure and NPK provide improvements in porosity and decreases soil penetration resistance. Moreover, soil levels of P, K, Zn and Cu increase with the application of 80 m3.ha-1 of liquid swine manure.
Regardless of the type of fertilization, the contents of P, Mn and Zn are higher in the 0.00 0.10 m layer, while the content of S and Fe are higher in the 0.10-0.20 m layer.
Application of liquid swine manure provides increases in soybean and maize grain yield, and can be recommended instead of NPK mineral fertilizer at a dose of 58.33 m3.ha-1 for maize, and 104.93 m3.ha-1 for soybeans, in integrated fertility soils.