Introduction
Agricultural activities have the potential to bring about major changes in the areas where they are carried out. Such anthropogenic interference significantly influences the dynamics of the global carbon flux. The conventional preparation of soil, with most tillage performed with plows and harrows, causes carbon losses, especially when soil physically protected in aggregates and is destroyed by agricultural implements (Loss, Pereira, Costa, and Beutler, 2014).
Agroecological management helps to improve the physical and chemical attributes of soil, with potential for good crop development and for maintaining quality. In this system, the contribution of soil organic matter (SOM) is highlighted in quantities that favor aggregation, nutrient availability, and plant biodiversity. In addition, agroecological management favors the use of soil cover (living or dead), reducing the heating of the surface layer and maintaining the humidity at adequate levels. This practice favors infiltration, which reduces erosion losses, and minimizes external inputs into the production system.
Aggregation is directly influenced by the SOM dynamics, and starts with the union of particles through the action of biological, physical. and chemical cycles, culminating in the formation of microaggregates (Tisdall and Oades, 1982). Important factors that influence soil aggregation include; soil fauna, soil microorganisms, roots; inorganic agents, and environmental variables. Many interactions occur among these factors, resulting in the formation and stabilization of aggregates (Bronick and Lal, 2005). Agricultural management, soil type, and soil aggregation may favor the physical protection of organic matter, thus reducing its decomposition (Pullman and Marinissen, 2004). Impacts on the environment, soil, and biological activity result in alterations in the formation of biogenic and physiogenic aggregates.
One method used to quantify the labile compartment of SOM involves the determination of mineralizable carbon, originating from the breakdown of organic molecules, which are converted to biomass and energy through microbial respiration, reflecting the activity of the soil biota in the decomposition of SOM (Rosa, Olszevski, Mendona, Costa, Correia, 2003; Bossuyt et al., 2002). In this way, high respiration rates (mineralizable carbon) can result in greater carbon cycling and nutrients for the plants (D’Andréa, Silva, Curi, Siqueira, and Carneiro, 2002).
The levels of mineralizable C in the soil can be modified by the adopted agricultural management system. The objective of the present study was to quantify the mineralizable C in aggregates of different formation pathways (biogenic and physiogenic) in agroecological management systems in the city of Seropédica, state of Rio de Janeiro, Brazil.
Material and methods
The study was carried out with samples collected in the area of the Integrated Agroecological Production System (SIPA), also known as Agroecological Farm Km 47, located in the Embrapa Agrobiology in the city of Seropédica, 22° 44' 38" S, 43°42'28' W, at an altitude of 33 m. The climate is classified as Aw, according to Koppen. The soil was classified as Red-Yellow Argisol (Santos et al., 2013), located in an area with smooth undulating relief with sandy loam texture in the surface horizon, with 784, 168, and 48 g/kg (0-0.05 m) sand, clay and silt, respectively (Loss, De Lima, Pereira, Ribeiro and Cunha, 2013b), and it is regularly cultivated with vegetables and fruits.
Samples were collected in May of 2014 and five areas were selected, with the following history of use: (1) agroforestry system (AFS), with 10 years of planting, consisting of banana (Musa sapientum), jussara palm (Euterpe edulis), cocoa (Theobroma cacao), papaya (Carica papaya), guapuruvu (Schizolobium parahyba), urucum (Bxa orellana L), and a^aí (Euterpe olearacea); (2) 15 years of perennial coffee cultivation (Coffea canephora) in full sun (C-SUN); (3) 15 years of perennial coffee cultivation (Coffea canephora), shaded with Gliricidia (C-SHA), the coffee areas receive annual placement fertilization with Bokashi in the pit; (4) 10 years of cultivation in alleys of Flemingia (Flemingia macrophylla) with bean pods (Phaseolus vulgaris var. Vulgaris) (AL-FLE); (5) 6 years of notill planting of corn (Zea mays)/eggplant (Solanum melongena) (NT), furrow fertilization is performed in this area at the time of planting with cattle manure (doses between 50 and 100 kg/ha of N), and top-dressing fertilization with poultry litter (doses between 100 and 200 kg/ha of N).
Clods were collected with the aid of a hoe at a depth of 0-0.05 m by opening transverse tr enches by the seeding lines. After collection, the clods were manually separated and deposited in a set of sieves with mesh diameters of 9.7 and 8.0 mm for subsequent sieving. Retained clods were retained and taken to the laboratory for further sorting, where they were analyzed under a magnifying glass and separated by hand according to the morphological standards established by Bullock, Federoff, Jongerius, Stoops, and Tursina (1985). Two classes of aggregates were obtained: biogenic (rounded forms, originating from the intestinal tract of the soil macrofauna organisms, especially Oligochaetes [ earthworms and/or those where it is possible to identify root activity]) and physiogenic (angular forms, formed by long cycles of wetting and drying). The levels of Ca2+, Mg2+, K, Na+, Al3+, H+Al, P and pH in water of the aggregates was determined as described by Donagema, Campos, Calderano, T eixeira and Viana (2011) (Table 1).
Table 1 Chemical characterization of biogenic and physiogenic aggregates in agroecological management systems at a depth of 0-0.05 m. Seropédica, Brazil.
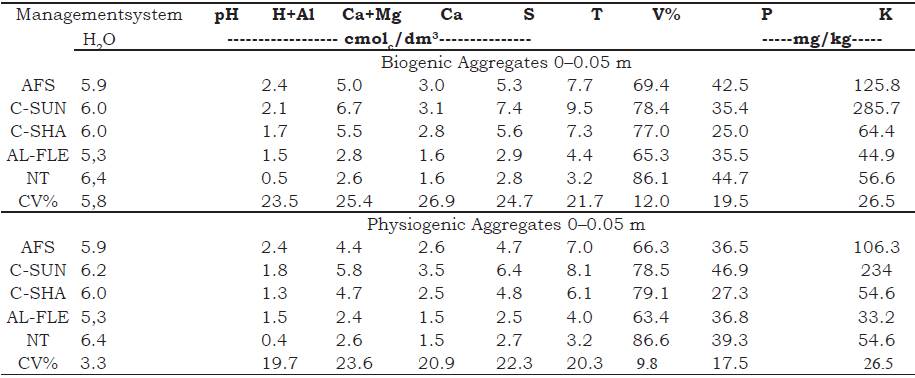
Air-dried samples of aggregates were remoistened by respraying water twice daily over 2 weeks to reactivate the activity of the organisms (Gonçalves, Monteiro, Guerra and De-Polli, 2002). Then, the field capacity (FC) was determined through the funnel method (Loss, Pereira, Beutler, Perin and Anjos, 2013a), which involves weighing 25 g of each aggregate sample and placing them in a 10 cm diameter plastic funnel sealed with glass wool at the base (to avoid loss of material). Then, the aggregates were saturated with distilled water and the funnels were covered with plastic film to reduce water loss through evaporation. After 4 hours, to drain excess water, approximately 5 g of each aggregate sample was placed in an oven at 105°C until a constant mass was reached. The field capacity was obtained using the following equation 1:
where: M1= mass of the wet sample (g). M2 = mass of the dry sample (g).
The evolution of C-CO2 - potentially mineralizable carbon - was evaluated in the laboratory as described by Mendonça and Matos (2005). Aggregates (25 g) retained in the 4 mm sieve were weighed and placed in 500 cm3 glass containers, which were hermetically sealed. The humidity of the aggregates was adjusted to 65% of the previously determined field capacity. Two plastic cups were added to each container: one containing 30 mL of 0.5 mol/l NaOH solution to capture the C-CO2 from the soil biota activity and one with 30 mL of distilled water to maintain constant moisture. Samples were evaluated in November and December 2015 at 24-hour intervals for the first 7 days, 48 hours between the 8th and 17th days, and 96 hours between the 18th and 37th days. Each container was opened for exactly 15 min to allow air exchange (including two blanks, only with the NaOH cups and distilled water, both with 30 mL). During air exchange, NaOH was titrated with HCl 0.25 mol/l; 10 mL of the NaOH cup was pipetted into a 125 mL Erlenmeyer flask, after which 10 mL of BaCl2 0.05 mol/l and three drops of phenolphthalein indicator 1% were added. The endpoint occurred when pink turned to white, noting the amount of HCl used. Following titration and 15 min of air exchange, another 30 mL of 0.5 mol/l NaOH was added, and the containers were closed for further incubation. This method is based on the principle that CO2 from soil organisms reacts with the titrated NaOH. The amount of evolved C-CO2 is obtained in mg 100/cm3 of soil, during the monitoring interval. The calculation used to obtain this value is as follows equation 2:
where: B = volume of HCl used for the blank titration; V = volume of HCl used for the sample titration (mL); 6 = atomic mass of carbon (12) divided by moles of CO2 that react with NaOH; V1 = total volume of NaOH used for CO2 capture; V2 = volume of NaOH used for the titration (mL).
Total organic carbon (TOC) was determined by wet oxidation with potassium dichromate at a concentration (0.167 mol/l) and titration with ammonium ferrous sulphate (0.20 mol/l), as described by Yeomans and Bremner (1988). Total N was determined by digestion with H2SO4 and H2O2; the extract obtained was subjected to steam distillation (Kjeldahl) with NaOH and the titration of the collected was made with boric acid (Tedesco, Gianello, Bissani, Bohen, and Volkweiss, 1995).
The results were analyzed for data normality and homogeneity using the Lilliefors test and the Cochran and Bartlett test (Ribeiro Júnior, 2001), respectively. Subsequently, data were analyzed as a completely randomized design with five treatments, two formation pathways, and four replicates. The results were submitted to analysis of variance using the F test, and mean values, when significant, were compared by the Scott-Knott test at 5% probability using the statistical software SAEG version 9.0 (Statistical and Genetic Analysis System - UFV, Federal University of Vinosa).
Results and discussion
The relative percentage of biogenic and physiogenic aggregates from the evaluated agroecological management systems are presented in Table 2. No significant differences were observed between the relative compositions of biogenic and physiogenic aggregates. For the AFS and NT systems, the physiogenic aggregates presented higher percentages than the biogenic aggregates.
Table 2 Mean values of biogenic and physiogenic aggregates (%) in agroecological management systems at a depth of 0-0.05 m. Seropédica, Brazil.
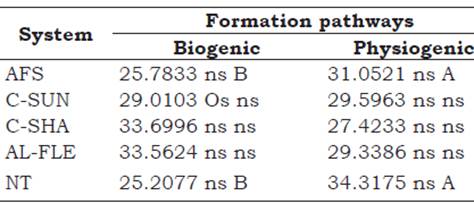
Means followed by the same uppercase letter in the row do not differ among the types of aggregates, and those with the same lowercase letter in the column do not differ among the evaluated systems by the Scott-Knott test at 5%. AFS: agroforestry system, C-SUN: coffee grown in full sun, C-SHA: coffee grown in the shade, AL-FLE: cultivation in alleys, NT: notill planting system.
Studies investigating aggregate type (biogenic, intermediate, and physiogenic) and stability in a secondary forest area at different recovery stages, and in a mixed pasture area managed in the upper-third of Haplic Cambisol in the city of Pinheiral-RJ, revealed no differences between the mass composition of each type of aggregate formed by the different pathways when evaluating areas of secondary forest in advanced stage and in pasture areas. However, differences were observed in areas of secondary forest in the middle and early stages (Silva-Neto, Pereira, Fernandes, and Corréa-Neto, 2016). In all studied areas, the physiogenic aggregates contributed more to the total mass of the aggregates compared with the biogenic and intermediate aggregates.
In studies on fertility and organic matter fractions in aggregates under different management systems (forest, pasture for 30 years, conventional planting for 56 years, and notill planting for 15 years) in Red Nitisol, southwest of Paraná, Loss et al. (2014) confirmed higher contributions of physiogenic aggregates compared with biogenic aggregates up to depths of 0.10 m. Those authors only observed biogenic aggregates in the area managed with conventional cultivation.
The daily evolution of C-CO2 in the biogenic and physiogenic aggregates is presented in Figure 1. In all evaluated systems, the highest rates of C mineralization in the biogenic and physiogenic aggregates were observed at the beginning of the incubation and gradually decreased until almost constant values were obtained from day 35 onwards. Changes in soil conditions, mainly in moisture content, causes microbes to respond quickly following periods of low activity. Additionally, these results agree with those obtained by Brookes (1995); Carvalho et al. (2008); Loss et al. (2013a); and Loss et al. (2013b), whereby the decomposition of the most labile organic materials, which are present in the most soluble fraction in water, was observed during the initial phase. After this phase, gradual mineralization begins and with more constant rates of compounds that are more resistant to the microbial attack.
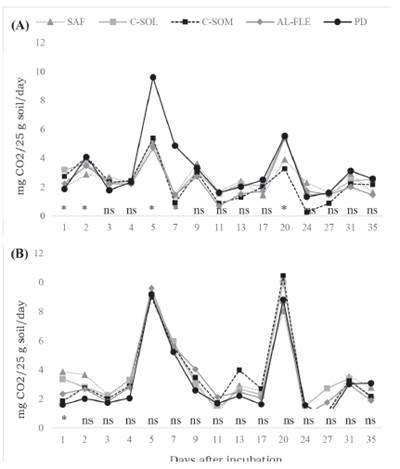
Figure 1. Daily evolution of C-CO2 in samples of biogenic and physiogenic aggregates collected in agroecological management systems at depths of 0-0.05 m, incubated for 35 days. Seropédica, Brazil. A) Biogenic aggregates, (B) physiogenic aggregates. AFS: agroforestry system, C-SUN: coffee grown in full sun, C-SHA: coffee grown in the shade, AL-FLE: cultivation in alleys, NT: no-till planting system.
No differences in C-CO2 release were observed for the physiogenic aggregates during the incubation period in the evaluated production systems, while differences were observed between systems for biogenic aggregates following 1, 2, 5, 7, and 20 days of incubation, with higher C-CO2 values for the NT system. The lowest TOC levels were found in this area (Table 3), which can be explained by the shorter implantation time with the NT sys tem, and because it is an area with a constant crop renewal. However, the low C/N ratio (Table 4) found in this system may be favoring higher releases of C-CO2 compared with the NT system. The lower C/N ratio found in the NT system can be attributed to the absence of an arboreal or shrub component, which is present in the other systems studied, and to the application of cattle manure and poultry litter in this area.
Table 3 C-CO2 accumulation after 35 days of incubation and the C/N ratio of biogenic and physiogenic aggregates in agroecological management systems at the depth of 0-0.05 m. Seropédica, Brazil.
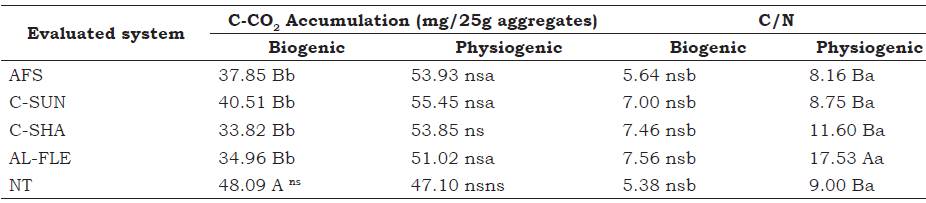
Means followed by the same lowercase letter in a row do not differ among the types of aggregates and those with the same uppercase letter in a column do not differ among the evaluated systems by the Scott-Knott test at 5%.
AFS: agroforestry system, C-SUN: coffee grown in full sun, C-SHA: coffee grown in the shade, AL-FLE: cultivation in alleys, NT: notill planting system.
When evaluating microbial activity in Red Latosol aggregates under different systems used in the Cerrado and when incubated in the laboratory, Loss et al. (2013a) observed higher values of C-CO2 in areas of NT that presented a C/N ratio of 7.83 and 6.92 before and after incubation, respectively, and higher TOC and N contents after the restoration of aggregate moisture and incubation. In areas under a NTS in the city of Marmeleiro in the south west of Paraná, Loss et al. (2014) presented the highest C-CO2 values in aggregates incubated in the laboratory, and at tributed this to the higher TOC contents deriving from the decomposition of ryegrass straw and soybean, associated with N from the biological nitrogen fixation (BNF) of soybeans. In this way, SOM has been more promptly available for the soil microbiota.
Among the agroecological systems studied, there were alternations in high C-CO2 release values, characterized by an increase followed by a decrease from the beginning of incubation until approximately day 20, followed by stabilization. This oscillation in C-CO2 evaluation is caused by the consumption of SOM by the microbiota present in soil (Carvalho et al., 2008). These microorganisms decompose the available SOM and release C-CO2, culminating in the observed release values (peaks). This release occurs until the substrate is depleted and the microorganisms die, resulting in a decrease in C-CO2 evaluation, which will be used as an energy source by the more resistant microorganisms. These will then multiply and generate new peaks of C-CO2 evolution (Carvalho et al., 2008).
Farias et al. (2005) investigated the evolution of C-CO2 and the influence of humic substances on samples of Red-Yellow Latosol up to 65 days of incubation, and observed C-CO2 peaks between days 25 and 30, when microbial activity began to stabilize. In an experiment assessing the incubation and evolution of C-CO2 in aggregates from areas under different agroecological management in the State of Rio de Janeiro, Loss et al. (2013b) observed an increase and decrease in the evolution of C-CO2 up to day 9, after which, C-CO2 in the aggregates stabilized.
The highest accumulation of C-CO2 (Table 4) in biogenic aggregates was observed in the area with the NT system of corn and eggplant. No differences in C-CO2 accumulation were observed in the physiogenic aggregates prior to stabilization at 35 days of incubation. Loss et al. (2013b) observed low levels of accumulations when they studied aggregates in areas with NT systems of corn and eggplant under agroecological management. Those authors attribute this pattern to the better compartmentalization of carbon, which reduces microbial in this system. This supports the results observed in the present study for the physiogenic aggregates, whereby the type of C stored is more recalcitrant compared with the more labile C stored in aggregates formed by the biogenic pathway.
The values of total organic carbon (TOC) and total nitrogen (TN) before and after incubation of the biogenic and physiogenic aggregates are presented in Table 4. Among the evaluated sys tems, the highest levels of TOC were observed in the C-SUN and C-SHA areas, followed by the AFS and AL-FLE areas, and the lowest levels were observed in the NT area for biogenic and physiogenic aggregates before and after incu bation. These results can be explained by the system implantation time. The coffee areas were the oldest among those studied, with 15 years of implementation. In addition to the contribution of coffee to the OM input in the soil, in the C-SHA area, there remains cover from the Gliricidia, In the C-SUN area, grass vegetation is maintained between coffee lines, which helps to preserve soil moisture and in the efficient contribution of carbon, both at the surface and subsurface. In addition, among the studied areas, soil stirring is lowest in the perennial coffee cultivations, which helps to preserve SOM.
Several studies investigating aggregates formed by different pathways have revealed higher levels of TOC in biogenic aggregates compared with physiogenic aggregates (Batista et al., 2013; Loss et al., 2014). Bossuyt, Six and Hendrix (2005) studied the role of earthworms in the physical protection of carbon using 13C-labelled sorghum and found that the contribution of bio genic aggregates was 22% higher than that of physiogenic aggregates.
Conclusions
Full sun and shade coffee systems favor an increase in the proportions of biogenic aggregates in the soil, total organic carbon content, and total soil nitrogen before incubation up to a depth of 0.05 m. The biogenic and physiogenic aggregates of the evaluated agroecological management systems presented peaks of C-CO2 evolution up to day 20 of incubation, with stabilization on day 35 of evaluation. The area cultivated with a notill system under agroecological management presented higher C-CO2 accumulation in biogenic aggregates and lower accumulation in physiogenic aggregates compared with the other evaluated systems.