Introduction
Traumatic brain injury (TBI) is a public health problem and its outcome is associated with the organization of trauma care and emergency services, including the possibility to access early diagnosis and timely surgery. A global mapping of the problem was recently completed and TBI was identified as the leading cause of the demand for neurosurgical intervention in most countries, particularly in middle and low-income areas. Traffic accidents are the major cause of the problem with an economic impact on the younger population, while falls are the most significant cause among the elderly.1 Computed tomography (CT)-scan for the initial assessment of TBI allows for an early identification of the primary injury (structural brain tissue damage) and facilitates the selection of patients who could benefit from early surgery.2 However, the role of CT in the identification of the secondary injury and to assess cell response (death or recovery) is limited and with poor specificity. The evolution of the secondary injury (changes on intracranial pressure, brain oxygenation, brain perfusion, etc.), is usually identified with advanced neuromonitoring systems.3,4
Keeping in mind that brain tissue damage may be associated with deficiencies in the healthcare process that may further aggravate the secondary injury, it would be important to learn about the systems to identify these molecular disorders affecting the outcome of all the above-mentioned processes: survival (recovery) or programmed cell death (apoptosis). A high-cost and technically complex diagnostic method such as cerebral microdialysis, helps to measure the metabolites in the microenvironment of the injured brain tissue; however, due to its high cost and technical complexity, this system is only accessible to research centers in very high income countries.5
There has been a recent growing search for biomarkers that enable the identification of the evolution of tissue injury and that may even foresee the magnitude of the brain damage before the identification of the primary injury using imaging. The objective of this review is to discuss some considerations around the most representative biomarkers in determining the magnitude of the TBI and their practical application in therapeutic decision-making protocols.
Biomarkers in traumatic brain injury
Among the large number of biomarkers studied, Protein S-100 beta (S-100ß), Ubiquitin C-terminal hydrolase-L1 (UCH-L1) and glial fibrillary acidic protein (GFAP) have proven to be the most sensitive and more helpful in the study of patients with TBI.6-8
A direct sample taken from the injured brain parenchyma or from the parenchyma at risk, could enable the study of the only available source of biomarkers, with direct information about the changes experienced after TBI, but it is not a feasible approach.
The cerebrospinal fluid (CSF), being closer to the injury, is also an excellent sample to measure. However, it requires aggressive maneuvers and is contraindicated in most patients with severe TBI.
For this reason, most biomarkers are studied in the peripheral blood since this method allows to make serial determinations with a simple and minimally invasive procedure (Fig. 1).9
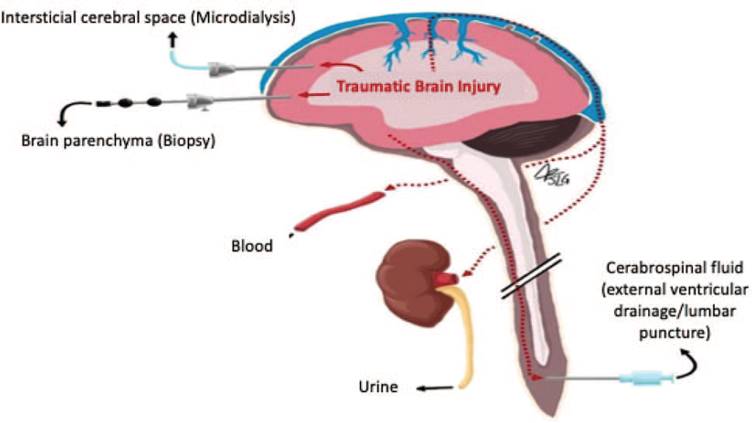
Source. Authors.
Figure 1 Potential sources of samples for measuring biomarkers. The diagram illustrates the different approaches to measure biomarkers. Although the CSF could provide measurements closer to the site of the traumatic injury, collecting a sample of CSF generates much more bleeding than obtaining peripheral blood. CSF = cerebrospinal fluid.
Calcium-binding protein S-100ß
Calcium-binding protein S-100ß produced in the astrocytes, is more available in the central nervous system, where it participates in regulating the signal-transduction pathways, cell morphology, and astrocyte proliferation through the interaction with transcription factors. After an injury, protein is released into the CSF and through the arachnoid villi, crosses into the blood stream. The presence in plasma of this protein is an indication of both neuronal damage, as increased blood-brain barrier patency.
Numerous studies have focused specifically on the prognostic role of serum S-100ß protein levels in TBI, correlating serum protein elevations with a worse prognosis.10,11) There are however, several extracerebral sources of S-100ß, such as chondrocytes, adipocytes, skeletal muscle tissue, bone marrow, and melanoma cells that may be confounding factors in tests of patients with TBI and other multiple trauma injuries.12,13
Ubiquitin C-terminal hydrolase-L1
UCH-L1 is a protease primarily released in the brain. Its role is the degradation of other proteins through the ubiquitin-proteasome system, either due to increased amounts, or because of the presence of damaged or malformed proteins. When following a TBI, blood is released into the injured tissues (with a peak 12 hours after the brain injury), the probability of abnormal CT findings may be predicted with a high probability. CH-L1 has been said to be highly specific for the human brain, and increased levels of CH-L1 have been associated with severity and worse TBI outcomes.14
Glial fibrillary acidic protein
GFAP participates in the production of intermediate filaments of the cellular cytoskeleton, particularly the astroglia. Following a TBI, this protein rises to increase support, movement, shape and function of the astroglia. Consequently, GFAP is marker of astrocyte injury and, together with the heart-type fatty acid-binding protein, elevated serum levels have been correlated in patients with positive findings in the CT, and are therefore considered useful biomarkers for early TBI diagnosis of varying degrees of severity, with a 100% sensibility and 45.9% specificity.(7,8)
Other brain biomarkers in TBI
Interleukin-1 (IL-1) is a peripheral and central inflammation mediator cytokine. It is made up by a number of agonists such as IL-1α and IL-1ß, with the latter being more related to TBI. Normally, IL-1ß is found in low concentrations in the brain; however, in TBI, the levels increase until they become toxic, which further aggravates the traumatic injury. When IL-1ß increases, other cytokines are released, hence activating the inflammation cascade, disrupting the blood-brain barrier and allowing the passage of inflammatory cells from the blood to the brain tissue.15,16
Microtubule-associated protein tau (MAPT) is found in the compartment of the neuronal axons. When binding to the axonal microtubules, its stability is modulated. Likewise, MAPT also contributes to neuronal development and polarity. Following a TBI, there is phosphorylation and excision of the MAPT, which rapidly elevate the levels of fragment C-terminal of tau. Its plasma concentration has proven to be of predictive value in patients after experiencing a TBI.17-19
Neuron-specific enolase (NSE) is a glycolytic enzyme allowing for the increase of neuronal chloride when it becomes activated. Its γ-γ isoform is found in the cytoplasm of neurons. NSE is the only biomarker that directly determines neuronal function damage. During the first 12 hours after a TBI, there is passive release due to cell destruction and a second peak is associated with increased mortality. However, NSE is also elevated as a result of hemolysis, which leads to false positive results.20
Phosphodiesterases are a group of enzymes that cause inactivation of cyclic adenosine monophosphate (AMPc), which is involved in physiological mechanisms including cell survival, inflammation, and synaptic plasticity. During TBI, cell damage increases proinflammatory cytokines (IL-1ß and Tumour Necrosis Factor (TNF)-α), then finally result in cell damage. Between 1 and 3 days after the injury AMPc levels drop and practically return to their baseline level.20
With regards to the prognosis of mild TBI-associated injuries, multiple biomarkers have been studied related to axonal damage; for instance, "calpain-cleaved αII-spectrin N-terminal fragment". High levels of this plasma protein are strongly associated to the risk of white matter injury; these lesions may be identified using diffusion tensor imaging.21
Clinical utility of biomarkers
Imaging relationship of biomarkers
Neuroimaging is considered the test of choice to identify intracranial injury following TBI, which involves a pressing demand for resources in medical centers. This has led to a number of studies focusing on reducing the number of brain trauma-associated images. Several lines of research have been opened in the field of neuro-proteomics, in order to establish the relationship of the plasma levels of certain biomarkers with imaging findings. A value below the threshold level may reduce the requirement for resources.
A recent multicenter study (ALERT-TBI) assess the utility of GFAP and UCH-L1 to predict brain injury in cranial CT of patients who experienced TBI. This assessment studied both proteins combined, with previously established cut-points; the sensitivity was 97% and the specificity was 99%, to detect intracranial injury in patients with TBI.6
Prognostic value of biomarkers
The most important use of biomarkers has been during the acute phase of trauma and over the development of the disease at 6 and 12 months. Most studies have found a relationship that enables to predict the prognosis of these patients.22
In case of protein S-100ß, blood values >0.7ng/mL have shown a relationship to mortality and functional outcomes (according to the Glasgow Outcome Scale), 6 months after the TBI.23 GFAP has also been considered a feasible predictor of outcome and mortality at 6 months.24,25
By evaluating protein UCH-L1 together with GFAP, it is possible to predict as well the evolution of patients on the second day after the traumatic injury.24,25
Correlation between biomarkers and the need for surgery
The behavior of the different biomarkers in patients with TBI has been studied, in an attempt to correlate the severity of the traumatic injury with the need to adopt a surgical approach in these patients.
The study of the level of GFAP and UCH-L1 biomarkers, together with CT, has helped to establish which patients present with potentially surgical injuries, with a 100% sensitivity.6
Furthermore, the use of S-100ß to predict the patients that require a surgical intervention has not been highly sensitive (Table 1).25
Table 1 Sensitivity and specificity of the principal biomarkers in TBI and the cut-point in blood samples.
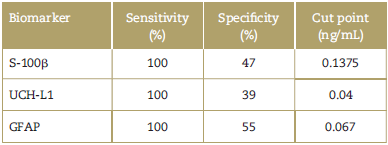
GFAP=glial fibrillary acidic protein, S-100ß = S-100 beta, TBI = traumatic brain injury, UCH-L1 = Ubiquitin C-terminal hydrolase-L1.
Source: Authors.
Table 1 summarizes the sensitivity and specificity of the biomarkers S-100ß, UCH-L1 and GFAP in TBI, with regard to their cut-point in blood samples, according to the reports by different trials. Similarly, a study with biomarker S-100ß showed a specificity of 47.3% and a sensitivity of 100%, with a cut-point of 0.1375 ng/mL, in patients with mild TBI.26
In terms of the predictive value of UCH-L1, it has shown a good relationship with TBI over the first 6 hours after the initiation of trauma. However, the studies and the meta-analysis on this biomarker still exhibit some biases to determine the severity of the trauma and its reference value varies among the different studies.27
With regard to biomarker GFAP measured in blood, with a cut-point of 0.067 ng/mL, its specificity is 55% and its sensitivity is 100%, to detect CT injuries in patients with skull fractures.28
Banyan protocols
In 2018, the Food and Drug Administration approved the use of the first kit of biomarkers developed by Banyan Biomarkers, Inc., for assessing patients with TBI. The kit provides semiquantitative measures of the serum concentrations of UCH-L1 and GFAP, using chemo-luminescent assays in blood tests. It is expected that the implementation of this new approach will reduce the use of brain CT for the assessment of TBI. This test, with threshold values of UCH-L1 of 327pg/mL and of GFAP of 22 pg/mL, has a sensitivity of 97.5%, a specificity of 36.5% and a negative predictive value of 99.6%.29,30
Curve of biomarkers in the follow-up of TBI
The major pathophysiological processes reflected by glial or neuronal biomarkers are the blood-brain barrier disruption and neuronal injury. Keeping these concepts in mind, Mondello et al, suggest the advisability of having a panel of complementary biomarkers showing different temporary profiles reflecting different pathophysiological conditions after a TBI (Fig. 2).31
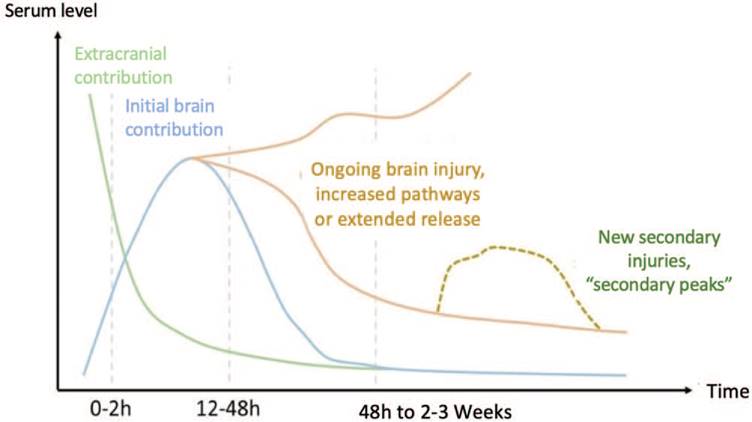
Source: Authors.
Figure 2 Expression and behavior of biomarkers following a TBI. At the onset of a TBI, the serum levels of biomarkers start to rise over the first few hours. However, the finding during this initial period has predominantly an extracranial contribution (green line). Later on, the concentration of biomarkers is reversed and brain proteins become more significant (blue line). As of the first 2 days of the brain injury, the lesion continues its natural evolution (orange line), during which secondary or tertiary injuries may develop that once again rise the biomarkers contributed by the brain (yellow dotted line). TBI = traumatic brain injury.
Proteomic studies using electrophoresis to identify the concentrations of the different biomarkers help us understand the most affected brain pathways during a TBI.32
Protein S-100ß in cell models is released 15 seconds after the traumatic event. In humans, it has been possible to detect the S-100ß 30 minutes post-trauma, with a half-life of 97 minutes. The peak presents on day 0 and the concentrations drop around day 6, both in the CSF and in serúm.33,35
UCH-L1 rises in the CSF over a 7-day period in patients with severe TBI. The increase is higher in patients with a low Glasgow Coma Score at 24 hours and in post-trauma complications. Consequently, this marker helps to determine the severity of the TBI.34
GFAP has been found to be elevated in patients with mild complicated and non-complicated TBI, after 90 minutes and 6 hours of the injury and normalizes at 24 hours. Therefore, GFAP could be more sensitive than S-100ß for the identification of intracranial injuries.35,36
Time elapsed from the TBI and the measurement of biomarkers
Timely assessment of the kinetics of the various biomarkers is essential for the development of a potential algorithm that facilitates an early diagnosis, in addition to making an assessment of the severity of the TBI in the emergency department.
Several studies have shown that following a TBI, the UCH-L1 and S-100ß levels rise over the first few hours and slowly start to decline after 12 hours. In contrast, the rise of GFAP levels occurs at a later stage, but remain high for several days.)36
Further studies are required to develop a possible algorithm for the use of biomarkers in neurotrauma, in order to shorten the time to collect the first sample, which according to the current information, is between 4.24hours (Table 2).
Table 2 Clinical studies assessing the behavior of the different biomarkers in TBI over time.
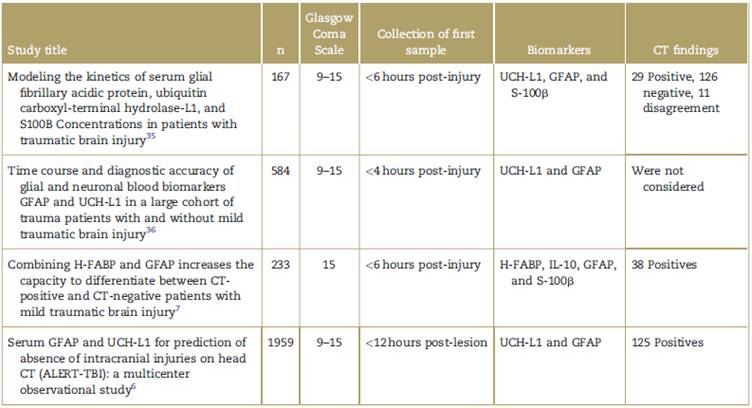
CT=computed tomography, GFAP = glial fibrillary acidic protein, H-FABP=heart-type fatty acid-binding protein, S-100ß = S-100 beta, TBI = traumatic brain injury, UCH-L1 =Ubiquitin C-terminal hydrolase-L1.
Source: Authors.
Conclusion
TBI is a clinical entity with significant socioeconomic and public health impact worldwide. The means currently available for the accurate diagnosis of patients with TBI have led to improved outcomes of the disease; however, it has not yet been possible to consolidate predictive models which are reliable enough and enable the implementation of effective therapeutic strategies and improved outcomes. Over the last few decades, various biomarkers for brain injury have been studied, with a view to develop a new diagnostic and prognostic tool for TBI.
Scientific evidence has already shown the sensitivity of some markers for potentially surgical injuries. The implementation of these biomarkers in protocols for TBI patients will allow for a reduced exposure to unnecessary radiations, optimization of care in the emergency department and then, predicting patient outcomes.