Services on Demand
Journal
Article
Indicators
-
Cited by SciELO
-
Access statistics
Related links
-
Cited by Google
-
Similars in SciELO
-
Similars in Google
Share
Biomédica
Print version ISSN 0120-4157
Biomédica vol.33 no.3 Bogotá july/Sept. 2013
https://doi.org/10.7705/biomedica.v33i3.1434
ARTÍCULO ORIGINAL
doi: http://dx.doi.org/10.7705/biomedica.v33i3.1434
1 Grupo de Investigación en Sustancias Bioactivas, GISB, Facultad de Química Farmacéutica, Universidad de Antioquia, Medellín, Colombia
2 Biología de la Conservación y Biotecnología, Facultad de Ciencias Básicas, Corporación Universitaria Santa Rosa de Cabal, Santa Rosa de Cabal, Colombia
3 Programa de Estudio y Control de Enfermedades Tropicales, PECET, Universidad de Antioquia, Medellín, Colombia
Author contributions:
Edison Osorio: obtención y caracterización de alcaloides aporfínicos.
Carolina Aguilera y Edison Osorio: ensayos enzimáticos.
Carlos Muskus, Nelson Naranjo, Edison Osorio y Marcel Marín: actividades de biología molecular.
Recibido: 25/10/12; aceptado:15/04/13
Introduction. Dihydrofolate reductase (DHFR) has been used successfully as a drug target in the area of anti-bacterial, anti-cancer and anti-malarial therapy. Although this bifunctional enzyme is also a potential drug target for treatment of leishmaniasis, there have been no reports on its efficacy against Leishmania ( Viannia ) species .
Materials and methods. The gene encoding the bifunctional DHFR and thymidylate synthase (TS) of Le. (V.) braziliensis was isolated and expressed in E. coli. The enzyme was purified and characterized. The inhibitory effects of antifolates and four aporphine alkaloids on its activity were evaluated.
Results. The full-length gene consists of a 1560-bp open reading frame encoding a 58 kDa translated peptide containing DHFR and TS domains linked together in a single polypeptide chain. The recombinant DHFR-TS enzyme revealed K m and V max values of 55.35 ± 4.02 µ M (mean ± SE) and 0.02 ± 5.34 x 10 -4 µ M/min respectively for dihydrofolic acid (H 2 F). The Le. braziliensis rDHFR-TS have Ki values for antimicrobial antifolates in the µM range. Methotrexate (MTX) was a more-potent inhibitor of enzymatic activity ( Ki = 22.0 µM) than trimethoprim ( Ki = 33 µM) and pyrimethamine ( Ki = 68 µM). These Ki values are significantly lower than those obtained for the aporphine alkaloids.
Conclusion. The results of the study show the inhibitory effect of antifolate drugs on enzymatic activity, indicating that Le. braziliensis rDHFR-TS could be a model to studying antifolate compounds as potential antiprotozoal drugs.
Keywords: Leishmania braziliensis, tetrahydrofolate dehydrogenase, thymidylate synthase, folic acid antagonists.
doi: http://dx.doi.org/10.7705/biomedica.v33i3.1434
Caracterización bioquímica de la enzima bifuncional dihidrofolato reductasa-timidilato sintasa de Leishmania ( Viannia ) y su evaluación como blanco molecular
Introducción. La dihidrofolato reductasa (DHFR) se ha utilizado como blanco molecular en tratamientos antibacterianos, anticancerígenos y antipalúdicos. También, actúa como blanco molecular en Leishmania ; sin embargo, no existen reportes de la enzima bifuncional en especies de Leishmania ( Viannia ).
Materiales y métodos. Se ha aislado y expresado en Escherichia coli el gen que codifica para la enzima bifuncional DHFR y la timidilato-sintasa (TS) de Leishmania braziliensis . La enzima recombinante se purificó y caracterizó, y se evaluó el efecto inhibitorio de algunos antifolatos, así como de cuatro alcaloides aporfínicos.
Resultados. El gen se compone de aproximadamente 1.560 pb y codifica un péptido de 58 kDa que contiene los dominios DHFR y TS ligados en una sola cadena polipeptídica. La enzima recombinante DHFR-TS, utilizando el dihidrofolato (H2F) como sustrato, presentó valores de K m y V max de 55,35 ± 4,02 (media ± el error estándar de la media) y de 0,02 ± 5,34 x 10 -4, respectivamente. La enzima rDHFR-TS de L. braziliensis presentó valores de Ki para los antifolatos en el rango de micras. El metotrexato fue el inhibidor más potente de la actividad enzimática ( Ki =22,0 mM) en comparación del trimetoprim ( Ki =33 mM) y la pirimetamina ( Ki =68 mM). Estos valores de Ki son significativamente más bajos en comparación con los obtenidos para los alcaloides aporfínicos.
Conclusión. Los resultados muestran el efecto inhibitorio de los antifolatos sobre la actividad enzimática, lo cual indica que la rDHFR-TS de L. braziliensis podría ser un modelo para estudiar moléculas antiprotozoarias potenciales.
Palabras clave: Leishmania braziliensis, tetrahidrofolato deshidrogenasa, timidilato sintasa, antagonistas del ácido fólico.
doi: http://dx.doi.org/10.7705/biomedica.v33i3.1434
Leishmaniasis is a parasitic disease caused by different species of Leishmania and transmitted by phlebotomine sand flies of the genus Lutzomyia in the Americas and Phlebotomus in the Old World. The disease constitutes a severe public health problem in at least 98 countries, as well as several that are non-endemic but are currently experiencing increased numbers of imported cases as a consequence of international tourism (1-4). An estimated 14 million people are infected and about two million new cases occur each year (5). In Latin America, Leishmania species of the subgenus Viannia are widespread and occur in several orders of mammals, including humans. The species Le. braziliensis is considered to be the main etiological agent of cutaneous leishmaniasis (CL) in at least 15 countries of the Americas and is also responsible for most mucocutaneous cases of the disease (6). In Colombia a second species, Le. (V.) panamensis, is also present and sometimes involved in mucocutaneous cases (7).
Control of the disease currently relies primarily on chemotherapy and to a lesser extent on vector control measures. A limited number of drugs is available and each has various shortcomings. Traditional antileishmanial systemic agents such as antimonials, pentamidine and amphotericin are limited by severe toxic side effects, emerging drug resistance and their requirement for parenteral administration (8-12). Newer agents such as oral miltefosine have shown efficacy and tolerability, although the efficacy of this compound has not been demonstrated for all Leishmania species. There is an urgent need to look for new alternatives (11,13).
One of the first steps in the drug development process is the identification of essential molecules which can act as potential targets. The genes encoding for the enzymes dihydrofolate reductase (DHFR) and thymidylate synthase (TS) have undergone a fusion event generating a single polypeptide but conserving the two functions in trypanosomatids (14,15). Dihydrofolate reductase (DHFR; EC 1.5.1.3) is an essential enzyme in the tetrahydrofolate pathway which catalyzes the NADPH-dependent reduction of 7,8-dihydrofolate (H 2 F) to the 5,6,7,8-tetrahydrofolate (H 4 F) needed to maintain intracellular pools of H 4 F and its derivatives; these are essential cofactors in the biosynthesis of purines, pyrimidines and several amino acids. As a result, it is the target enzyme of a group of antifolate drugs, such as methotrexate (MTX), trimethoprim, and pyrimethamine, which are already in use as anti-tumor and antimicrobial agents (15,16). This bifunctional enzyme is attractive as a drug target for several reasons; i) the metabolic pathway in which it participates is a critical route in parasite survival due to its role in synthesis of purine and pyrimidine nucleotides; ii ) as a result of the fusion event, the DHFR and TS activities are condensed into a single protein, so inhibition of a single polypeptide would block two steps in the nucleotide synthesis - this is in contrast to the human counterpart, where DHFR and TS enzymes occur as two separate monofunctional proteins. iii ) structural differences between the fusion protein DHFR-TS in trypanosomatids and the individual polypeptides in humans make this protein an attractive target for rational drug design (17).
In the present study a 1.5 Kbp coding for the bifunctional DHFR-TS from Le. braziliensis and Le. panamensis was amplified and cloned. The complete coding sequence (CD) of Le. panamensis was sequenced and aligned against the sequence annotated in the Le. braziliensis genome (GeneDB). The gene encoding the DHFR-TS from Le. braziliensis was expressed as recombinant protein in E. coli. The enzymatic activity and kinetic parameters of the purified Le. braziliensis rDHFR-TS were determined using spectrophotometry. Finally, the inhibitory activity of four aporphine alkaloids and some 2,4- diamino-pyrimidine antifolates were evaluated with the rDHFR-TS recombinant protein.
Materials and methods
Amplification of the rDHFR-TS gene
The complete coding sequence of the DHFR-TS gene from Le. braziliensis and Le. panamensis strains were amplified using DNA isolated from promastigotes in a PCR System 9700 thermocycler (Applied Biosystems). The genomic DNA was prepared using the Wizard DNA extraction kit (Promega), following the manufacturer's instructions. DNA from Trypanosoma cruzi was kindly provided by the Chagas' Disease Laboratory of the University of Antioquia. The ORF was amplified using the sense primer 5´-cgc ggatcc atgtccagggcagctgcga-3´ containing the BamHI site and the antisense primer 5´-ccc aagctt ctatacggccatctccatctt-3´ containing the HindIII site. The restriction sites included allowed directional cloning of the genes in the pQE30 expression vector (Qiagen). The amplification reaction contained 200-300 ng of genomic DNA, 1X reaction buffer, 2 mM MgCl 2, 0.2 mM of each dNTP and 0.05U/µl of Taq DNA polymerase in 25 µl of total volume. The PCR cycling condition consisted of a single cycle at 95°C for 5 min, followed by 35 cycles of 94°C for 1 min, primer annealing at 60°C for 45 s and extension at 72°C for 2 min. The PCR included a final extension cycle at 72°C for 7 min. An aliquot of the amplified products was visualized by electrophoresis on 1% agarose gel containing 0.5 µg/ml of ethidium bromide.
Cloning of the DHFR-TS gene
The 1.5 Kbp PCR product was purified from the agarose gel and initially cloned into the pGEM-TEasy vector (Promega) following the manufacturer's instructions, transformed into DH5 a E. coli competent cells and plated on LB agar medium containing 100 µg/ml of ampicillin. Bacteria clones were screened for the presence of the 1.5 Kbp-insert by restriction enzyme and/or PCR using the DHFRTS specific primers. The insert was subcloned in the BamHI/HindIII restriction sites, presented in the pQE30 his-tagged expression vector (Qiagen) and expressed as a recombinant protein in the E. coli M15-pREP4 strain upon induction with isopropyl ß -D-thiogalactopyranoside (IPTG), as described below.
Sequencing of the DHFR-TS gene of Le. panamensis
The PCR product of Le. panamensis previously cloned in the pGEM-T-Easy vector was sequenced by both chains using the primers T7 and SP6 which flank the multicloning site. The forward and reverse sequences were edited using the Chromas Lite program (Technelysium Pty Ltd).
Expression and purification of the recombinant DHFR-TS protein
Single colonies of E. coli M15(pREP4) harboring the pQE30 construct were grown overnight in 10 ml of Luria-Bertoni medium with ampicillin (100 µg/ml) and kanamycin (25 µg/ml) in a thermal shaker. Five mL of the overnight culture was used to grow up 1 L of bacteria in the same medium under the same conditions. The cells were grown at 25ºC with shaking until an OD of 0.4-0.6 at A 600 was reached. Protein expression was induced with IPTG to a final concentration of 1 mM and 2mM for 24 h, prior to harvesting of cells by centrifugation at 8000×g for 10 min at 4°C. Aliquots of 200 µl were taken from the culture at 6, 12 and 24 h post-induction to evaluate protein expression in a 12% SDS-PAGE gel. The cell pellet obtained from 1 L-culture was re-suspended in binding buffer (10 mM imidazole, 300 mM NaCl, and 50 mM NaH 2 PO 4, pH 8.0) and lysed by sonication at 2500 db. After centrifugation at 18 000 g at 4°C for 20 min, the supernatant was collected and loaded onto a precharged nickel affinity column (Ni-NTA resin). The column was washed once with binding buffer (300 mM NaCl, and 50 mM NaH 2 PO 4, pH 8.0) and once with 20 mM imidazole. The recombinant protein was eluted under native conditions with 250 mM imidazole. Each step of the purification procedure was monitored on 12% SDS-PAGE gels and stained with Coomassie blue. Protein concentration was determined by (18) using a protein assay kit from Bio-Rad Laboratories (Hercules, Calif.). The pH was adjusted to 6.0 to stabilize enzyme activity.
Enzyme activity assay and kinetic evaluation
The activity of the recombinant enzyme rDHFR-TS of Le. braziliensis was determined spectrophotometrically (CaryBio 50) by monitoring the decrease of NADPH absorbance at 340 nm and 28°C. The assay was developed based on the basic DHFR assay described by Reche et al. (19) with minor modifications. All procedures were conducted in sealed PMMA cuvettes. The standard reaction mix (1.0 ml) consisted of 25 µM of the rDHFR-TS in a buffer solution (pH 6.0), containing NADPH 100 µM, H 2 F 50 µM and BSA 1 mg/ml. The Michaelis-Menten constants (K m ) were determined at constant concentration of NADPH and varying the H 2 F concentration in a range 25 to 100 µM. The kinetic data were analyzed by a nonlinear least squares fit using the software GraphPadPrism® Version 4.00 for Windows.
Assay for sensitivity of DHFR-TS to antifolates and aporphine compounds
Four aporphine alkaloids from Rollinia pittieri and Pseudomalmea boyacana (Annonaceae) (20) were evaluated as potential inhibitors of the rDHFR-TS enzyme (Table 1). The antifolates methrotexate (MTX), trimethoprim and pyrimethamine were included as inhibition controls. Inhibition experiments were conducted at constant concentrations of NADPH (100 µM) and various H 2 F concentrations ranging from 25 to 100 µM, in the presence of different concentrations of the inhibitors. For determinations of the IC 50 values, initial velocities were measured at five or more concentrations, revealing <10% to >100% of the control activity. Assays were started by the addition of dihydrofolic acid after pre-incubation of the enzyme with each inhibitor dissolved in assay buffer. Inhibition constants ( K i ) of the compounds were calculated from a nonlinear least square equation for competitive or noncompetitive inhibition. The type of inhibition by the compounds against rDHFR-TS was analyzed by standard double reciprocal plots.
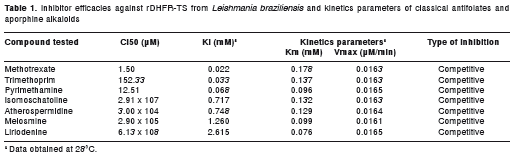
Results
PCR amplification, cloning and sequencing of the ORF of the DHFR-TS gene
The complete ORF of the DHFR-TS enzyme was amplified in two species of the Viannia subgenus as well as in T. cruzi . The reaction yielded a PCR product of approximately 1.5 Kbp (Fig. 1). The amplified products of Le. panamensis were purified and cloned directionally in the BamHI and Hind III restriction site of the pQE30 expression vector (data not shown). The Le. panamensis DHFR-TS sequence of the UA140 Colombian strain (the nucleotide sequence has been assigned the accession number KC846139 by GenBank) was aligned against the Le. braziliensis (LbrM.06.0830), sequence obtained from geneDB, Sanger Institute, and DHFR-TS sequences of Le. major (XM_001680805.1), Le. infantum (FR796438.1) and Le. amazonensis (X51735.1) , which were downloaded from GenBank. A high conservation at nucleotide level was observed among species, ranging from 85-98% depending on the species.
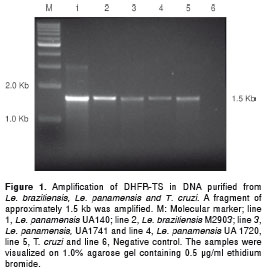
Induction and purification of the recombinant protein
Induction of the expression with 1 and 2 mM of IPTG resulted in a specific protein band of the expected size (58 kDa) either at 12 or 24 h postinduction (Fig. 2a). Purification was performed by nickel-nitrylotriacetic acid (Ni-NTA) metal-affinity chromatography. The protein displayed a 58-kDa band on 12% SDS-PAGE (figure 2b) which is in agreement with the expected size from the amino acid sequence (21). The purified protein was stable at pH 6.0, over a period of 1 month when stored at -20°C. A good amount of the rDHFR-TS protein was obtained after purification on the Ni-NTA resin.
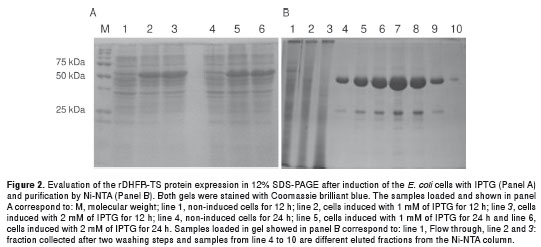
Enzymatic activity and kinetics parameters of the recombinant enzyme
To evaluate the enzymatic activity, 25 µM of rDHFRTS was added to the reaction mixture and changes in absorbance at 340 nm were monitored. A fast drop in the absorbance was observed decreasing from 0.34 to 0.15 (Fig. 3), providing evidence that rDHFR-TS catalyzes the NADPH-dependent reduction of H 2 F to H 4 F at 28ºC in 60 min. The time and temperature to perform the assays was 30 min and 28ºC; under these conditions the cofactor NADPH retains its initial absorption spectrum. As control, an enzyme-free reaction mixture was evaluated and no changes in absorbance values were observed. Based on this enzyme activity, we proceeded to determine the steady-state kinetic parameters for the enzyme substrate and the cofactor (H 2 F and NADPH). The K m and V max values of the rDHFR-TS from Le. braziliensis measured in the H 2 F reaction were 55.35 ± 4.02 µ M and 0.02 ± 5.34 x 10 -4 µ M/min, respectively. One unit of the enzyme was defined as the amount required to catalyze the generation of 1 µM of product per minute, equivalent to 4.30 x 10 -4 . The specific activity was determined to be 0.0087 U/mg (data not shown).
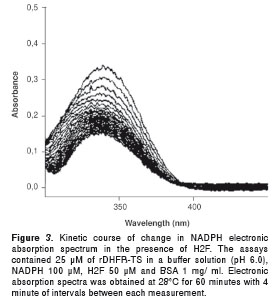
Evaluation of enzyme inhibition
The kinetics of enzyme inhibition by three 2,4- diamino-pyrimidine classical antifolates (MTX, trimethoprim and pyrimethamine) as well as four aporphine alkaloids (liriodenine, melosmine, atherospermidine and isomoschatoline) were evaluated as inhibitors of the purified rDHFR-TS from Le. braziliensis . H 2 F, the substrate for the DHFR enzyme, shares structural similarities in its chemical structure with the classical antifolates via their common aminopyrimidine ring (Fig. 4). We would therefore expect these drugs to compete for the active site in the DHFR domain, potentially leading to an inhibitory effect. To examine this possibility, the kinetic results of enzyme inhibition of the compounds were transformed using a Lineweaver Burk plot. Indeed, MTX, pyrimethamine, and trimethoprim all inhibited the enzymatic activity in a dose-dependent manner, MTX being the best inhibitor (IC 50 = 1.5 µM) (Fig. 5). The Ki values of the classical antifolates were in the micromolar range, whereas the aporphine compounds inhibited the target enzyme at the mM level (Table 1). In addition, the Lineweaver Burk plots indicate that all the evaluated antifolate compounds are competitive inhibitors for the enzyme.
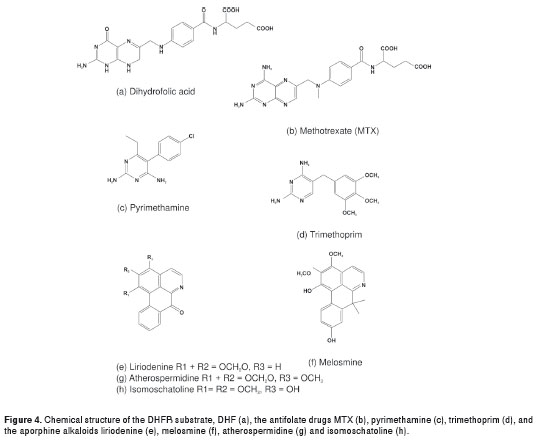
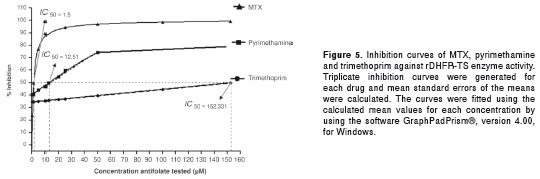
Discussion
DHFR is a key enzyme in folate metabolism, linked to the production of thymidine (12). In several species of protozoa, the catalytic activities for the enzymes DHFR and TS reside on a single polypeptide chain, constituting a bifunctional DHFR-TS enzyme (14,15). The ORF of the DHFR-TS has been amplified, cloned and sequenced; the sequences showed a high degree of conservation, both at nucleotide (96%) and protein (90%) level. The bifunctional enzyme has been thus considered as a potential drug target (12,15). Nevertheless, there are no reports on the bifunctional enzyme on Le. ( V. ) species. A few inhibition studies using different compounds have been conducted on a few Leishmania species, including Le. major and Le. mexicana , as well as T. brucei and T. cruzi (22-30). It was considered interesting to evaluate the activity of the rDHFRTS in the presence of classical antifolates. The rDHFR-TS enzyme purified in this study showed good activity, some of the compounds evaluated being inhibitors of the recombinant enzyme. In this sense, MTX was the best inhibitor (IC 50 = 1.50 µM; Ki = 22.0 µM). In previous studies, MTX has been shown to be a potent inhibitor of Le. major and T. cruzi DHFR-TS and also to show activity against promastigotes of different Leishmania species (24,26). The classical anti-microbial DHFR inhibitors trimethoprim (IC 50 = 152.33 µM; Ki = 33 µM) and pyrimethamine (IC 50 = 12.51 µM; Ki = 68 µM) were, in general, not very selective for the analogous leishmanial enzyme compared to MTX. Trimethoprim and pyrimethamine have been reported as moderately selective for L e. major and T. cruzi DHFR (25,31). The aminopteridine ring of MTX and the aminopyrimidine ring of pyrimethamine and trimethoprim closely resemble those of the substrate, H 2 F (Fig. 4). We would thus expect these antifolate compounds to compete with the substrate by binding to amino acid residues in the hydrophobic pocket of the active site of the rDHFR-TS enzyme, producing the observed inhibitory effect. MTX is a particularly potent inhibitor compared to pyrimethamine and trimethoprim because the molecule, by having a pteridine ring and glutamic acid, is an only slightly modified version of DHF, the normal substrate of DHFR. It thus competes effectively with the substrate for the rDHFR-TS active site. The results obtained indicate that the structural requirements for DHFR inhibition are critically important in determining the possible inhibitory activity of different compounds tested and in the design of selective antifolates for parasite chemotherapy.
In contrast, no studies have addressed the inhibitory effects of aporphine alkaloids on rDHFR-TS from Leishmania . Thus, we were also interested in evaluating these non-classical analogues as inhibitors of Le. braziliensis DHFRTS. The aporphine alkaloids were weak inhibitors of enzymatic activity, as indicated by their high Ki values. However, our kinetics studies showed that the most active compound was isomoschatoline ( Ki = 0.72 mM), followed by atherospermidine ( Ki = 0.75 mM). The aporphine alkaloid showed some degree of activity when it was assayed against Le. braziliensis promastigotes (M2903) with IC 50 s of less than 23 µg/ml (32). This indicates that its possible mode of action is not related to the inhibition of DHFR. Folate analogues that inhibit DHFR generally contain 2,4-diamino substitution in the pyrimidine ring, typified by MTX. In contrast, folate analogues that inhibit TS generally contain an oxo- or methyl-oxo substitution as in Pemetrexed and Raltiterxed, both already in clinical use (33). The aporphine alkaloids evaluated present some of the structural requirements necessary to inhibit TS, although further studies are required to check its activity. In addition, it should be noted that the aporphine and oxoaporphine alkaloids are isoquinoline compounds known to have various pharmacological properties, including antiparasitic activity. Another mode of action could thus be involved (34). The mechanism of action of aporphine alkaloids seems to be related, at least in part, to the inhibition of topoisomerase II by DNA intercalation or minor groove binding (35,36). The identification of liriodenine as a strong topoisomerase II catalytic inhibitor and a topoisomerase II poison has led to the search for other topoisomerase II inhibitors among the aporphine alkaloids (35).
In conclusion, this study reports the first isolation, cloning, and expression of the Le. braziliensis DHFR-TS gene, providing a corresponding purified rDHFR-TS enzyme of approximately 58 kDa that is catalytically active. Additionally, the results of the study show the inhibitory effect of antifolate drugs on enzymatic activity, indicating that Le. braziliensis rDHFR-TS could be a model for studying antifolate compounds as potential antiprotozoal drugs. The following sections will elaborate on the overall structure of the enzymes, the interactions with the substrate and inhibitors and future structurebased drug design goals. One aspect to consider is the several potential resistance mechanisms to DHFR that have been discovered including overexpression of the enzymes DHFR-TS and pteridine reductase 1 (PTR1) (37,38). The enzyme PTR1 is predominantly involved in reduction of biopterin to dihydrobiopterin and tetrahydrobiopterin but also capable of reducing dihydrofolate to tetrahydrofolate (38). Hence, a combined strategy to target both DHFR and PTR1 will be more effective as a true antileishmanial drug (12).
The authors express that there are no conflicts of interest with this manuscript.
This work was supported by COLCIENCIAS (Agreement No. RC108-2003) and CODI-Estrategia de Sostenibilidad GISB 2011-2012.
Corresponding author:
Carlos Muskus, Sede de Investigación Universitaria-SIU, laboratorio 632, Calle 62 N° 52-59, Medellín, Colombia Phone: (574) 219 6507; fax: (574) 219 6511 carmusk@yahoo.com
1. Lawn SD, Whetham J, Chiodini PL, Kanagalingam J, Watson J, Behrens RH, et al . New world mucosal and cutaneous leishmaniasis: An emerging health problem among British travellers. QJM. 2004;97:781-8. http://dx.doi.org/10.1093/qjmed/hch127 [ Links ]
2. Antinori S, Gianelli E, Calattini S, Longhi E, Gramiccia M, Corbellino M. Cutaneous leishmaniasis: An increasing threat for travellers. Clin Microbiol Infect. 2005;11:343-6. http://dx.doi.org/10.1111/j.1469-0691.2004.01046.x [ Links ]
3. Scarisbrick JJ, Chiodini PL, Watson J, Moody A, Armstrong M, Lockwood D, et al . Clinical features and diagnosis of 42 travellers with cutaneous leishmaniasis. Travel Med Infect Dis. 2006;4:14-21. http://dx.doi.org/10.1016/j.tmaid.2004.11.002 [ Links ]
4. Stark D, van Hal S, Lee R, Marriott D, Harkness J. Leishmaniasis, an emerging imported infection: Report of 20 cases from Australia. J Travel Med. 2008;15:351-4. http://dx.doi.org/10.1111/j.1708-8305.2008.00223.x [ Links ]
5. World Health Organization. Control of leishmaniasis. Geneva: Sixtieth World Health Assembly; 2007. Fecha de consulta: 10 de enero de 2012. Disponible en: http://www.who.int/gb/ebwha/pdf_files/WHA60/A60_10-en.pdf. [ Links ]
6. Cuervo P, de Jesús JB, Junqueira M, Mendoça-Lima L, Gonzáles LJ, Betancourt L, et al . Proteome analysis of Leishmania ( Viannia ) braziliensis by two-dimensional gel electrophoresis and mass spectrometry. Mol Biochem Parasitol. 2007;154:6-21. http://dx.doi.org/10.1016/j.molbiopara.2007.03.013 [ Links ]
7. Osorio LE, Castillo CM, Ochoa MT. Mucosal leishmaniasis due to Leishmania ( Viannia ) panamensis in Colombia: Clinical characteristics. Am J Trop Med Hyg. 1998;59:49-52. [ Links ]
8. Lawn SD, Armstrong M, Chilton D, Whitty CJ. Electrocardiographic and biochemical adverse effects of sodium stibogluconate during treatment of cutaneous and mucosal leishmaniasis among returned travellers. Trans R Soc Trop Med Hyg. 2006;100:264-9. [ Links ]
9. Santos DO, Coutinho CE, Madeira MF, Bottino CG, Vieira RT, Nascimento SB, et al . Leishmaniasis treatment a challenge that remains: A review. Parasitol Res. 2008;103:1-10. http://dx.doi.org/10.1007/s00436-008-0943-2. [ Links ]
10. Chakravarty J, Sundar S. Drug resistance in leishmaniasis. J Glob Infect Dis. 2010;2:167-76. http://dx.doi.org/10.4103/0974-777X.62887 [ Links ]
11. Freitas-Junior LH, Chatelain E, Kim HA, Siqueira-Neto JL. Visceral leishmaniasis treatment: What do we have, what do we need and how to deliver it? Int J Parasitol Drugs Drug Resist. 2012;2:11-9. http://dx.doi.org/10.1016/j.ijpddr.2012.01.003 [ Links ]
12. Singh N, Kumar M, Kumar Singh R. Leishmaniasis: Current status of available drugs and new potential drug targets. Asian Pac J Trop Med. 2012;5:485-97. http://dx.doi.org/10.1016/S1995-7645(12)60084-4 [ Links ]
13. Ouellette M, Drummelsmith J, Papadopoulou B. Leishmaniasis: Drugs in the clinic, resistance and new developments. Drug Resist Updat. 2004;7:257-66. http://dx.doi.org/10.1016/j.drup.2004.07.002 [ Links ]
14. Ivanetich KM, Santi DV. Bifunctional thymidylate synthase-dihydrofolate reductase in protozoa. FASEB J. 1990;4:1591-7. [ Links ]
15. Maganti L, Manoharan P, Ghoshal N. Probing the structure of Leishmania donovani chagasi DHFR-TS: Comparative protein modeling and protein-ligand interaction studies. J Mol Model. 2010;16:153947. http://dx.doi.org/10.1007/s00894-010-0649-0 [ Links ]
16. Watkins, WM, Mberu EK, Winstanley PA, Plowe CV. The efficacy of antifolate antimalarial combinations in Africa: A predictive model based on pharmacodynamic and pharmacokinetic analyses. Parasitol Today. 1997;13:459-64. http://dx.doi.org/10.1016/S0169-4758(97)01124- [ Links ]
17. Anderson AC. Targeting DHFR in parasitic protozoa. Drug Discov Today. 2005;10:121-8. http://dx.doi.org/10.1016/S1359-6446(04)03308-2 [ Links ]
18. Bradford, M. A rapid and sensitive method for the quantitation of microgram quantities of protein utilizing the principle of protein-dye binding. Anal Biochem. 1976;72:248-54. http://dx.doi.org/10.1016/0003-2697(76)90527-3 [ Links ]
19. Reche P, Arrebola R, Santi D, Pacanowska D, Ruiz L. Expression and characterization of the Trypanosoma cruzi dihydrofolate reductase domain. Mol Biochem Parasitol. 1996;76:175-85. http://dx.doi.org/10.1016/0166-6851(95)02557-X [ Links ]
20. Osorio E, Montoya G, Muñoz K, Arango G. Actividad antiplasmodial de alcaloides aporfínicos de Rollinia pittieri y Pseudomalmea boyacana (Annonaceae). Vitae. 2006;13:45-50. [ Links ]
21. Arrebola R, Olmo A, Reche P, Garvey EP, Santi DV, Ruiz-Pérez LM, et al . Isolation and characterization of a mutant dihydrofolate reductase-thymidylate synthase from methotrexate-resistant Leishmania cells. J Biol Chem. 1994;269:10590-6. [ Links ]
22. Scott DA, Coombs GH, Sanderson BE. Effects of methotrexate and other antifolates on the growth and dihydrofolate reductase activity of leishmania promastigotes. Biochem Pharmacol. 1987;36:2043-5. http://dx.doi.org/10.1016/0006-2952(87)90508-9 [ Links ]
23. Berman JD, King M, Edwards N. Antileishmanial activities of 2,4-diaminoquinazoline putative dihydrofolate reductase inhibitors. Antimicrob Agents Chemother. 1989;33:1860-3. http://dx.doi.org/10.1128/AAC.33.11.1860 [ Links ]
24. Hardy LW, Matthews W, Nare B, Beverley SM. Biochemical and genetic tests for inhibitors of Leishmania pteridine pathways. Exp Parasitol. 1997;87:157-69. [ Links ]
25. Chowdhury SF, Villamor VB, Guerrero RH, Leal I, Brun R, Croft SL, et al . Design, synthesis, and evaluation of inhibitors of trypanosomal and leishmanial dihydrofolate reductase. J Med Chem. 1999;42:4300-12. http://dx.doi.org/10.1016/j.bmc.2005.01.025 [ Links ]
26. Zuccotto F, Brun R, Pacanowska DG, Pérez LMR, Gilbert IH. The structure-based design and synthesis of selective inhibitor of Trypanosoma cruzi dihydrofolate reductase. Bioorg Med Chem Lett. 1999;9:1463-8. http://dx.doi.org/10.1016/S0960-894X(99)00213-9 [ Links ]
27. Zuccotto F, Zvelebil M, Brun R, Chowdhury SF, Lucrezia RD, Leal I, et al . Novel inhibitors of Trypanosoma cruzi dihydrofolate reductase. Eur J Med Chem. 2001;36:395-405. http://dx.doi.org/10.1016/S0223-5234(01)01235-1 [ Links ]
28. Chowdhury SF, Guerrero RH, Brun R, Ruiz-Pérez LM, Pacanowska DG, Gilbert IH. Synthesis and testing of 5-benzyl-2,4-diaminopyrimidines as potential inhibitors of leishmanial and trypanosomal dihydrofolate reductase. J Enzyme Inhib Med Chem. 2002;17:293-302. [ Links ]
29. Pez D, Leal I, Zuccotto F, Boussard C, Brun R, Croft SL, et al . 2,4-Diaminopyrimidines as inhibitors of leishmanial and trypanosomal dihydrofolate reductase. Bioorg Med Chem. 2003;11:4693-711. http://dx.doi.org/10.1016/j.bmc.2003.08.012 [ Links ]
30. Khabnadideh S, Pez D, Musso A, Brun R, Ruiz-Pérez LM, González-Pacanowska D, et al . Design, synthesis and evaluation of 2,4-diaminoquinazolines as inhibitors of trypanosomal and leishmanial dihydrofolate reductase. Bioorg Med Chem. 2005;13:2637-49. http://dx.doi.org/10.1016/j.bmc.2005.01.025 [ Links ]
31. Gamarro F, Yu PL, Zhao J, Edman U, Greene PJ, Santi D. Trypanosoma brucei dihydrofolate reductase-thymidylate synthase: Gene isolation and expression and characterization of the enzyme. Mol Biochem Parasitol. 1995;72:11-22. http://dx.doi.org/10.1016/0166-6851(95)00059-A [ Links ]
32. Osorio E. Evaluación de la actividad antiparasitaria de extractos y compuestos alcaloidales de especies de la familia Annonaceae (tesis). Medellín: Universidad de Antioquia; 2005. p. 136-40. [ Links ]
33. Gangjee A, Qiu Y, Li W, Kisliuk RL. Potent dual thymidylate synthase and dihydrofolate reductase inhibitors: Classical and nonclassical 2-amino-4-oxo-5-arylthio-substituted-6-methylthieno[2,3-d]pyrimidine antifolates. J Med Chem. 2008;51:5789-97. http://dx.doi.org/10.1021/jm8006933. [ Links ]
34. Osorio E, Robledo SM, Bastida J. Alkaloids with antiprotozoal activity. En: Cordell GA, editor. The alkaloids: Chemistry and biology. The Netherlands: Academic Press; 2008. p. 113-90. [ Links ]
35. Woo SH, Sun NJ, Cassady JM, Snapka RM. Topoisomerase II inhibition by aporphine alkaloids. Biochem Pharmacol. 1999;57:1141-5. http://dx.doi.org/10.1016/S0006-2952(99)00018-0 [ Links ]
36. Hoet S, Stévigny C, Block S, Opperdoes F, Colson P, aldeyrou B, et al. Alkaloids from Cassytha filiformis and related aporphines: Antitrypanosomal activity, cytotoxicity, and interaction with DNA and topoisomerases. Planta Med. 2004;70:407-13. http://dx.doi.org/10.1055/s-2004-818967 [ Links ]
37. Wang J, Leblanc E, Chang CF, Papadopoulou B, Bray T, Whiteley JM, et al. Pterin and folate reduction by the Leishmania tarentolae H Locus short-chain dehydrogenase/reductase PTR1. Arch Biochem Biophys. 1997;342:197-202. http://dx.doi.org/10.1006/abbi.1997.0126 [ Links ]
38. Vickers TJ, Beverley SM. Folate metabolic pathways in Leishmania. Essays Biochem. 2011;51:63-80. http://dx.doi.org/10.1042/bse0510063. [ Links ]