INTRODUCTION
Endophytic fungi are microorganisms that colonize plant tissues intercellularly and intracellularly throughout their life cycle, or part of it, and do not cause any disease in the host (Hyde and Soytong, 2008). They maintain stable relations with their host, since they live asymptomatically with the plant (Pamphile and Azevedo, 2002). Azevedo and Araújo, (2007) define endophytes as any microorganism, cultivated or not, that colonizes the interior of the host plant without causing apparent damages or visible external structures. Mendes and Azevedo, (2008) extended the concept of endophytes and classified them into two main types: Type 1, which do not produce structures external to their hosts, and Type II, which produce external structures such as mycorrhizal fungi and bacteria that produce nitrogen fixation nodules.
Endophytic microorganisms have great biotechnological interest. Their properties apply to different areas and are highly useful in agriculture in by plant growth promoting, biological pest control and increasing the resistance of plants to stress conditions (Ikram et al., 2018; Li etal., 2018). Several studies indicate that endophytes reduce the attack of insects and pathogenic fungi against the host plant (Landum et al., 2016; Jaber and Ownley, 2018). Further, the interaction between endophytes and plants involves the production of several secondary metabolites or bioactive substances, with industrial interest for the in development of pathogen control systems or with diverse biological activities (Nisa et al., 2015; Venugopalan and Srivastava, 2015).
Certain endophytes are uncultivable; they are difficult to maintain in a physiologically stable state without host cells, being more complex than other free-living fungi (Dissanayake et al., 2018). However, a considerable number of endophytes are cultivable, and the production of bioactive compounds from host plants by these endophytes is important from ecological, molecular and biochemical points of view (Bungtongdee et al., 2018). This is because it is easier to access microorganism cultivation conditions and optimize the process, thus reducing costs, and increasing productivity and reproducibility, without any adverse impact on the environment (Kusari etal., 2012). Therefore, endophytic micro-organisms are acknowledged sources of new natural products to be exploited by medicine, agriculture, and industry (Pimentel et al., 2011; Nisa et al., 2015). The application of endophytic microorganisms in the biological control of pests and insects has shown promising results; however, methods for the effective application on the field must be further developed. The interference of external factors, such as climatic conditions in different regions, interactions with other plants and microbial species, the number of endophytes to be applied, as well as the need to demonstrate the phytosanitary safety of the strains are factors that currently limit the application of endophytic microorganisms in biological control (Polonio et al., 2015).
Enzymes are used in various industrial processes such as textiles, cosmetics, foods, and beverages. Microbial enzymes are not subject to production or supply limitations, and their diversity increases daily due to the discovery of new enzymes and applications (Corrêa et al., 2014). The use of enzymes improves the quality of a product and makes it easier to obtain it. Moreover, each substance has specific enzymes that degrade it (Robinson, 2015).
Microorganism-produced enzymes have great interest in several areas, including food processing (amylases, pectinases, proteases and cellulases), the tissue industry (cellulases), the leather industry (proteases and lipases), the manufacture of detergents (proteases and lipases), pharmaceuticals, medical therapy and in the field of molecular biology (Nielsen, 1998; Corrêa et al., 2014).
The Laboratory of Microbial Biotechnology of the State University of Maringa, Maringá PR Brazil, has isolated and studied endophytes from different plant hosts during the last decade, including Sapindus saponaria Linnaeus 1753, that is used in popular medicine as a tranquilizer, astringent, diuretic, and expectorant and the fruit extracts have activity as antifungal (Garcia et al., 2012; Alberto et al., 2016). Several endophytes have not yet been analyzed, albeit maintained under optimal laboratory conditions. Therefore, the current study objectives the bioprospection of several S. saponaria endophytes for the enzymatic production of amylase, cellulase, and pectinase, and the antagonistic activity against phytopathogens Fusarium solani (Mart.) Sacc. 1881 , Glomerella sp. Spauld. & H. Schrenk 1903, and Moniliophthora perniciosa (Stahel) Aime & Phillips-Mora 2006. Although these phytopathogens are not specifically associated with S. saponaria, they affect crops of agronomic importance such as soybean, grapevine, and cocoa, respectively.
MATERIALS AND METHODS
Microbial strains
Strains of endophytic fungi, used in the current assay (Table 1), were isolated from S. saponaria L. (Sapindaceae), retrieved from the campus of the State University of Maringa, Maringá PR Brazil, by Garcia et al., (2012), and are included in the stock of the Microbial Biotechnology Laboratory (LBIOMIC) of the Department ofBiotechnology, Genetics and Cell Biology (DBC) of the State University of Maringa, Maringá PR Brazil. The pathogenic fungus strains used were F. solani, M. perniciosa (Laboratory of Genetics of Microorganisms, ESALQ-USP, São Paulo, Brazil) and Glomeralla sp. (CNPUV378, Embrapa Grape and Wine, Bento Gonçalves RS Brazil).
Molecular Taxonomy and Phylogenetic analysis
So that endophytes could be molecularly identified, DNA was extracted using the PowerSoil DNA Isolation Kit (MO BIO Laboratories, Inc., Carlsbad CA, USA), following the manufacturer's instructions. The amplification of ribosomal DNA region, ITS1-5.8S-ITS2, using the primers ITS1 (5'-TCCGTAGGTGAACCTGCGG-3') and ITS4 (5'-TCCCCGCTTATTGATATGC-3'), were performed as described by White et al., (1990).
The amplified regions of isolates were purified by enzyme shrimp alkaline phosphatase (SAP, Phosphatase, Alkaline shrimp - Sigma Aldrich, Saint Louis, USA) and exonuclease I (EXO, Exonuclease I, E. coli-BioLabs, New England, USA). Purification reactions were performed with 8 μL PCR product, 0.5 μL EXO (10 U/L) and 1 μL SAP (1 U/L) and incubated in a thermocycler for 1 h at 37 °C, followed by 15 min at 80 °C. Extracted DNA was then subjected to quantification on 1 % agarose gel, and photographed. PCR products were sequenced using the ABI-PRISM 3100 Genetic Analyzer equipment (Applied Biosystems).
Nucleotide sequences were compared with those deposited in the NCBI database (National Center for Biotechnology Information) for taxonomic identification, initially determined by the best identity rate obtained. Phylogenetic analysis was performed as described by Garcia et al., (2012). Sequences with greater NCBI similarity and other genetically distant sequences were used as external groups to force alignment.
In vitro antagonist activity of endophytic fungi
Antagonist activity against pathogenic fungus F. solani, M. perniciosa and Glomeralla sp. was evaluated by a dual-culture technique described by Campanile et al., (2007), with modifications described by Polonio et al., (2015).
The in vitro competition interactions between endophytes and pathogens were analyzed according to scale by Badalyan et al., (2004), with modifications, based on three types of interactions: A, B, and C, with C being divided into subcategories. The interaction types are: A = the inhibition of mycelial growth with contact; B = inhibition from a distance; C = endophytic growth on the pathogen without initial inhibition; CA1 and CA2 = partial and complete endophytic growth, respectively, on the pathogen after initial inhibition with mycelial contact; CB1 and CB2 = partial and complete endophytic growth, respectively, on the pathogen after initial inhibition from a distance.
Analysis of enzymatic production
The enzymatic assay was performed as described by Ribeiro et al., (2018) with modifications: In Mancini solution, 0.5 % of inductive substrate was added and pH was adjusted for each enzyme: amylase (starch soluble, pH 6.0, HiMedia, Mumbai, India); cellulase (Carboxymethylcellulose or CMC, pH 5.0, Sigma Aldrich, Saint Louis, USA) and pectinase (pectin, pH 5.0, Sigma Aldrich, Saint Louis, USA).
The culture media was modified for the detection of each enzyme: starch agar medium (18 g/L agar; 10 g/L starch; 0.1 M citrate-phosphate buffer, pH 5.0) for amylase; CMC-agar (18 g/L agar; 10 g/L CMC; 0.1 M sodium acetate buffer, pH 5.0) for cellulase; and pectin-agar (18 g/L agar; 10 g/L pectin; 0.1 M sodium acetate buffer, pH 5.0) for pectinase.
A positive control experiment was performed using a commercially available enzyme (cellulase from Aspergillus niger, α-amylase from porcine pancreas, pectinase from Aspergillus, Sigma Aldrich, Saint Louis, USA) at 1 mg/mL. Plates were incubated at 28 °C for 24 h and then stained with 0.1 % Congo red dye (cellulase), iodine solution 1 N (amylase) and hydrochloric acid solution 5 N (pectinase).
Determination of enzymatic activity
Determination of enzymatic activity was performed as described by Ribeiro et al., (2018), with modifications: Induction solution (1 % w/v) was adjusted for each enzyme (CMC solution in sodium citrate buffer 50 mM, pH 4.8; starch solution in acetate buffer 0.2 M, pH 5.0; pectin solution in citrate buffer 0.1 M, pH 4.0).
Statistical analysis
The means obtained in antagonist activity and enzymatic production were compared by the Scott-Knott test (p<0.05) using the statistical program Sisvar 5.5 (Ferreira, 2008).
RESULTS
Phylogenetic Analysis
BLAST and phylogenetic analyses based on the ITS1-5.8S-ITS2 sequencing data of18 endophytic fungi isolated from S. saponaria, together with those with the highest identity in the NCBI database, resulted in the molecular taxonomy based on the identification of the genera Phomopsis, Sordariomycetes, Diaporthe and Colletotrichum (Table 1, Fig. 1). Phomopsis was the most prevalent genus endophytic isolates of the analyzed samples of our microbial collection.
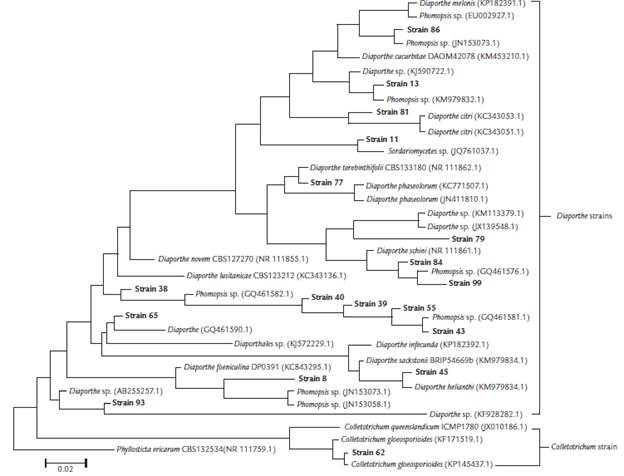
Figure 1 Phylogenetic tree of foliar endophytic fungi of Sapindus saponaria with other fungi obtained from the GenBank database, constructed by MEGA 6.05, with neighbor-joining method, using p-distance for nucleotides with the pairwise gap deletion. The numbers on the tree indicate the percentage of times the group on the right occurred on the same node during consensus evaluation (bootstrap with 10,000 replications). The strain Phyllosticta ericarum CBS 132534 (NR 111759.1) was used as an external group.
Antagonistic activity
Table 2 shows endophyte antagonistic activity against phytopathogens. The best performance was observed against F. solani with an inhibition rate (Im %) between 41.8 % and 67.5 %. The endophytic strain with the best result was SS81 (Diaporthe citri), with a 67.5 % inhibition rate. The best inhibition rate (57.4 %) against Glomerella sp. was obtained for endophyte SS79 (Diaporthe sp.) and against M. perniciosa for SS77 (Diaporthe phaseolorum) with 64.8 %. Most of the in vitro interactions were type A, the inhibition of mycelial growth with contact.
Enzymatic activity
After the growth of S. saponaria endophytic fungi in a liquid medium and cup plate, halos measuring 15.53 mm for cellulase, 8.66 mm for amylase (only endophyte SS77 presented amylase results in this test) and 11.33 mm for pectinase were obtained (Table 3). The endophytes that produced pectinase comprised SS65 (Diaporthe sp.), SS77 (Diaporthe phaseolorum) and SS84 (Phomopsis sp.), while strains that produced cellulase were SS08 (Phomopsis sp.), SS13 (Phomopsis sp.), SS62 (Colletotrichum gloeosporioides), SS65 (Diaporthe sp.) and SS93 (Diaporthe sp.).
Table 3 Evaluation of enzymatic production by endophytic fungi isolated from Sapindus saponaria in cup plate test.
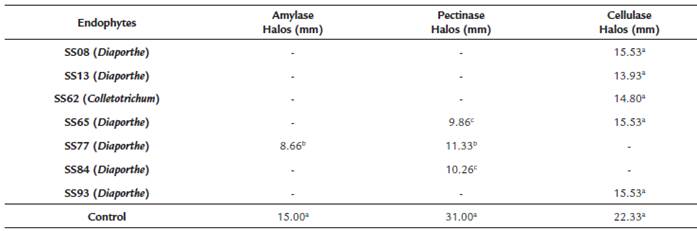
Mean of areas followed by the same letter do not differ by Scott-Knott test (p < 0.05).
Endophytes with positive results in enzyme production were evaluated by spectrophotometer. Several strains with no visible halos in the cup plate had positive results in spectrophotometric evaluation: three in the test for amylase and pectinase (SS65, SS77, and SS84), and five in the test for cellulase (SS08, SS13, SS62, SS65, SS93). The 3,5-dinitrosalicylic acid (DNS) assay is a robust colorimetric technique for the quantification of enzymatic activity based on the liberation of reducing sugars and is widely used in several papers (Sharma et al., 2016; Salim et al., 2017), facilitating comparison of the obtained results. Current analysis demonstrated that strain SS65 produced 1.16 UI μmol/min of amylase, SS77 produced 2.74 UI μmol/min of pectinase, and SS08 produced 1.51 UI μmol/min of cellulose (Table 4).
DISCUSSION
The genus Phomopsis is represented by approximately 1000 species of anamorphic ascomycetes and its teleomorphic phase is represented by the genus Diaporthe (Pandey et al., 2003; Murali et al., 2006). In the current study, it was the most abundant genus. Similar results were found in other research works (Rhoden et al., 2012; Sebastianes et al., 2013; Ribeiro et al., 2018). Sebastianes et al., (2013) analyzed the diversity of endophytic fungi isolated from the leaves and branches of Rhizophora mangle, Avicennia schaueriana and Laguncularia racemosa from two mangroves in the state of São Paulo. Molecular identification showed that the fungus community associated with these mangroves was composed of at least 34 different genera, with Diaporthe as the most frequent (22.67 %), coupled with Colletotrichum (19.19 %), Fusarium (11.34 %), Trichoderma (8.72 %) and Xylaria (7.56 %) (Sebastianes et al., 2013).
The endophyte of the genus Diaporthe is commonly isolated from several plant families. Ribeiro et al., (2018) demonstrated that the endophytic genus was the most abundant (78 %) in the foliar tissue of Pachystachys lutea.Rhoden et al., (2012) also observed an 84.3 % abundance of Diaporthe sp. endophytic isolates in the leaves of Trichilia elegans. In crops with high economic value, such as cocoa, coffee, soybean, and common bean, this genus has also been isolated as an endophyte (Ribeiro et al., 2018).
The current study also demonstrated the ability of Diaporthe to reduce the growth of phytopathogens F. solani, Glomerella sp. and M. perniciosa. Since the type of interaction between endophytes and pathogens was the inhibition of mycelial growth with contact, the above suggests that competition for space featured the inhibition mechanism (Gao et al., 2010; Ribeiro et al., 2018). Studies on endophytic Diaporthe citri isolated from guaco (Mikania glomerata Spreng.) obtained 60 % inhibition rates against F. solani and 66 % against D. bryoniae, with the same type of competitive interaction (Polonio et al., 2015).
Agriculture has undergone severe production losses due to phytopathogens. Although decreasing the attack of phytopathogenic insects and microorganisms, xenobiotic pesticides represent high risks for humans, with deleterious environmental impacts (Jayaraj et al., 2016). The natural and biological control of insect pests and diseases that affect crop plants has been focused during the last decades to reduce the use of pesticides in agriculture (Lacava et al., 2014). Biocontrol has often been used in tropical countries, including Brazil (Lacava et al., 2014). There has been increasing interest in endophytes as biological control agents because they inhibit plant pathogens (Bogner et al., 2016; Bahroun et al., 2017). Most interesting are observations which suggest that endophytes may play some role in the protection of the host plant from plant pathogens or other agents of destruction including insects and herbivores, these microorganisms produce substances (antibiotics and enzymes) that directly inhibit pathogens or induce systemic resistance in the host (Gao et al., 2010; Strobel, 2018).
Landum et al., (2016) also verified bio-control with endophytes isolated from Olea europaea against Colletotrichum acutatum. All fungal isolates showed some inhibitory action on the growth of the phytopathogen. The same authors reported that volatile substances produced by these isolates could play a significant role in reducing C. acutatum. Borgiono et al., (2015) evaluated the endophytic fungi isolated from organic coffee against phytopathogens Glomerella sp. and Colletotrichum sp. and obtained inhibition rate ranging between 46.21 % and 60.86 %. The biotechnological potential of endophytic fungi of the grapevine (Vitis labrusca L.) was tested in vitro for the control of phytopathogenic of vines, including Alternaria sp., Sphaceloma sp. and Glomerella sp., with more than 50 % inhibition (Felber et al., 2015). It is important to point out that, although the genera Diaporthe and Colletotrichum could be found as phytopathogens, they were isolated in the endophytic condition in the present work, i.e., they do not cause any symptoms of diseases and could be used in the safely biotechnological process.
The data and results obtained in the current study confirmed that endophytic fungi are promising agents for biological control and may inhibit or reduce phytopathogen growth in several ways, including mycoparasitism, antibiosis, the production of metabolites, competition for nutrients, or resistance induction in plants (Terhonen et al., 2016; Ribeiro et al., 2018). The use of endophytes in crops presents advantages as these organisms can colonize and establish a long-lasting interaction with their hosts. Besides this, liquid formulations can be developed for foliar applications or seed treatments. However, there were some limitations, such as biotic and abiotic factors, as well as the preparation of farmers and the storage of formulations and the need for further developments to endophytic applications (Sessitsch et al., in press).
Endophytic fungi from S. saponaria also produced amylase, pectinase, and cellulase, detected by the cup plate technique. The measurement of the halos formed after the addition of the dyes showed clearly defined zones, indicating enzymatic activity. The enzymatic production by endophytic fungi is variable since it is related to the specificity between the host plant and fungus (Tan and Zou, 2001). Enzyme production facilitates the penetration of endophytes in the host plant and is also involved in the competition with other microorganisms, such as pathogenic fungi (Fouda et al., 2015; Ribeiro et al., 2018). They are mutualists, but some may become pathogenic on occasion when environmental and physiological conditions of the plant may allow (Strobel, 2018). Endophytes are a new source of many interesting industrial enzymes, such as lipases, phytases, amylases, proteases and cellulases (Corrêa et al., 2014).
One of the most studied models of cellulase production is Trichoderma reesei; the production by this species may vary between 0.2 and 137 μmol/min/mg (Gutiérrez-Rojas et al., 2015). In this study, strain SS08 (Diaporthe sp.) produced 1.51 U μmol/min of cellulase. For amylase, the best production was 1.16 U μmol/min for SS65 (Diaporthe sp.). Patil et al., (2015) analyzed extracellular enzymatic activities of endophytic fungi isolated from various medicinal plants, and three endophytes were able to produce extracellular amylase ranging between 0.1 and 4.1 U/ml; the best result was produced by Rhizoctonia sp., with 2.74 U μmol/min of pectinase being produced by SS77 (Diaporthe sp.). Hegde and Srinivas, (2017) evaluated the production of pectinase by endophytic fungi isolated from medicinal plants; the best result was 0.20 μmol/min produced by Talaomyces sp. These results show that endophytic fungi from S. saponaria are promising for enzymatic production and may be optimized to increase efficiency.
CONCLUSIONS
The current study shows that endophytes from S. saponaria L. may be a biological control agent against all analyzed phytopathogens and useful for enzymatic production, reinforcing the need to evaluate the isolated endophytes of plant hosts and the importance of the conservation of these strains in optimal stock conditions.