Services on Demand
Journal
Article
Indicators
-
Cited by SciELO
-
Access statistics
Related links
-
Cited by Google
-
Similars in SciELO
-
Similars in Google
Share
Agronomía Colombiana
Print version ISSN 0120-9965
Agron. colomb. vol.30 no.1 Bogotá Jan./Apr. 2012
1 Laboratory of Plant Physiology and Biochemistry, Department of Biology, Universidad Nacional de Colombia. Bogota (Colombia).
2 Forestry Division, Pizano. Zambrano (Colombia).
3 Corresponding author. lmmelgarejom@unal.edu.co
Received for publication: 17 August, 2011. Accepted for publication: 1 March, 2012.
ABSTRACT
Gmelina is an important forest species because of its adaptability to different tropical environments, rapid growth and high quality wood for many uses. Although the species thrives in lowlands, both wet and dry, water availability is the main limiting factor for production in the latter. The transpiration rate, stomatal resistance, water potential and chlorophyll and carotenoid pigments content were monitored for three climatic seasons (rainy, transitional and dry) and three ages (seedling (2- 10 months), juvenile (10-16 months) and adult (48-60 months)), in order to observe the physiological response of gmelina to conditions in northern Colombia. Transpiration rates decreased with the age of the trees and the critical value of leaf water potential, that generates stomatal closure, was observed below -2.6 MPa. The dry season resulted in increased carotenoid content, in contrast to the content of chlorophyll A, B and total.
Key words: ecophysiology, water relations, pigments, forestal.
RESUMEN
Gmelina es una especie de importancia forestal debido a su adaptabilidad a diferentes ambientes tropicales, su rápido crecimiento y su alta calidad de madera para múltiples usos. Si bien la especie prospera en las tierras bajas tanto húmedas como secas, es en éstas donde la disponibilidad hídrica constituye la principal limitante para la producción. En tres épocas climáticas (lluviosa, transición de lluviosa a seca y seca) y tres edades (plántula (2-10 meses), juvenil (10-16 meses) y adulto (48-60 meses)), se monitoreó la tasa de transpiración, la resistencia estomática, el potencial hídrico y el contenido de los pigmentos clorofila y carotenoides con el fin de conocer la respuesta fisiológica de gmelina frente a las condiciones de la zona norte de Colombia. Las tasas de transpiración disminuyeron con la edad de los árboles y el valor crítico del potencial hídrico foliar que genera cierre estomático se encontró por debajo de -2,6 MPa. En época seca se dio aumento en el contenido de carotenoides, en contraste a los contenidos de clorofila A, B y total.
Palabras clave: ecofisiología, relaciones hídricas, pigmentos, forestal.
Introduction
Gmelina arborea Roxb. is a broadleaved species native to Asia; in Colombia, it has been planted commercially in the dry plains of the Caribbean, north coast (Zambrano, Colombia) since 1983 by the company Pizano because of its rapid growth and quality of wood that allows multiple uses in the forest industry.
In the formation of dry forests in the Caribbean region, the bimodal pattern of precipitation means trees are exposed to a period of low to nonexistent water availability, whose range oscillates between 80 to 140 d, meaning water supply is the main limiting factor for productivity of the species used for reforestation. The plant physiological strategies in response to the microclimatic cycles guide reduced water loss due to natural processes, one of which is stomatal closure, which reduces water loss by transpiration but also restricts the entry of CO2, and thereby decreases the rate of photosynthesis and translocation of photoassimilates to different organs of the plant, thus decreasing the pressure gradient necessary for the entry of nutrients through the root (Breda et al., 2006). In tropical environments, the effects of low water availability are increased by high temperatures and radiation, which is why water deficit can be understood as a multidimensional metabolic stress (DaMatta, 2003). When the available water content in the environment gradually decreases, stomatal conductance decreases substantially, reducing transpiration, but without significantly affecting photosynthesis, because stomatal closure reduces the flow of water vapor more than the flow of CO2 (Kozlowski and Pallardy, 1997), resulting in reduced leaf area and growth, decreased root development and expansion, affecting plant height and canopy establishment (Martínez et al., 2002). Another response to the level of energy dissipation and regulation of the contents of carbon and nitrogen is the increase in carotenoid content, thus increasing receptor molecules of excess energy and the use of chlorophyll as a source of carbon and nitrogen (Tanaka and Tanaka, 2007).
The objective of this study was to determine the physiological response of G. arborea to the dry climatic conditions of the Colombian Caribbean, from the seedling to adult stages.
Materials and methods
We evaluated the response of G. arborea under field conditions, in experimental plots of 0.5 ha in which the trees were planted with a spacing of 3.5 by 2.5 m. The study area, Hacienda Monterrey belonging to Pizano located in Zambrano (Bolivar), is located at 9°44' N and 74°50' W, and has an average temperature of 28°C; during the study period rainfall totaled 2010 mm and evapotranspiration 3585 mm, with the seasons differentiated as: rainy season (high water availability and 72.27% RH), transitional (intermediate water availability and 77.0% RH) and dry season (low water availability and 35.79% RH). We evaluated three stages of development of the species: seedling (2-10 months), juvenile (10-16 months) and adult (48-60 months). The measurements taken during daily cycles, with intervals of 2 h, between 0800 and 1800 HR for transpiration rates (E) and stomatal resistance (rs), and between 0600 and 1800 HR for water potential (.), in three healthy leaves that were found among the first three nodes of a selected branch from the middle of three trees, one for each of the three age groups, for each time interval. Transpiration rate and stomatal resistance were measured with a steady-state porometer (LI-1600 Li-Cor Inc. Lincoln, Nebraska) and the water potential of the plants (the water status indicator of the plants) with a pressure chamber instrument (Model 650, PMS Instruments, Oregon, USA). 10 leaves were collected for the respective ages, seasons, and sampling times (at 0900 HR for the 0800 to 1000 HR range, 1100 HR for the 1000 HR to 1200 hr range, and 1300 HR for the 1200 to 1400 HR range), and were macerated with liquid N2 to a fine powder, weighed to 0.101 g of fresh tissue dander, and subjected to acetone extraction (80% v/v -10°C) and chlorophyll A, B, and total and carotenoid were determined as described by Lichtenthaler (1987). The methods were standardized and described in detail by Melgarejo et al. (2010).
Statistical analysis. The obtained data were processed using Statistix 9.0 software. The differences between experimental variables were assessed by one-way ANOVA.
Results and discussion
Transpiration rates, stomatal resistance and water potential
The transpiration rate of G. arborea (Fig. 1, left) decreases with age. In the rainy season, the seedlings had transpiration rates with an average of 30 µg H2O cm-2 s-1, while in adults the average transpiration rates were around 13 µg H2O cm-2 s-1. Juvenile trees expressed intermediate behavior. Stomatal resistance had an inverse behavior (Fig. 1, right), in the adult state average resistance was close to 5 s cm-1, while in the seedling stage this variable was close to zero. Some studies suggest that differences in transpiration rates at different ages may be because water must be transported to a significantly higher height in older trees, so to maintain a functional water transport homeostasis occurs in the potential water gradient, consequently decreasing stomatal conductance and transpiration (Delzon and Loustau, 2005). In the sampling carried out in the transitional season, values and trends in transpiration were similar to those found in the rainy season.
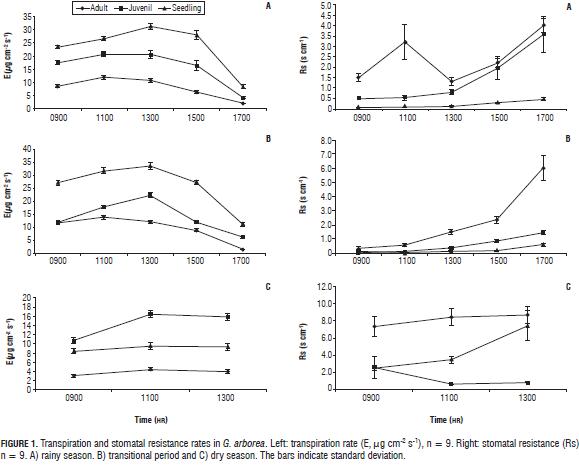
In the dry season, during the day, the transpiration rate was highest in the morning between 0800 and 1000 HR; it was also found that with increased temperature and decreased relative humidity the vapor pressure deficit increased and stomatal closure was generated, reducing transpiration. Similarly, the stomatal resistance values increased, as did the average temperature, up to 38°C, 5 degrees higher than the averages for the rainy and transitional periods (Tab. 1).
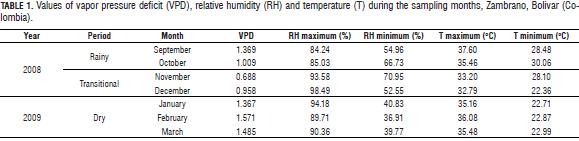
In Prunus armenica, similar responses have been found in reduced transpiration when trees are subjected to controlled drought stress, likewise stomatal conductance reached the lowest values at the end of the deficit period, showing a strong relationship between vapor pressure deficit and water vapor exchange which is controlled by leaf conductance. Furthermore, P. armenica trees vary daily patterns of transpiration and conductance according to light conditions (Barradas et al., 2005).
It was noted during the rainy and transitional periods that the leaves of G. arborea lost cell turgor and changed orientation, maximizing vertical position. The greater the angle of the leaf, the lower the irradiance received in the noon hours is, and water loss is lessened, similar to that reported for other species (Elheringer and Werk, 1986; Gindaba et al., 2004). After 2 weeks of the dry season, plants began the leaf abscission process in response to the water deficit, a characteristic considered as a resistance mechanism, because it reduces water loss through transpiration; similar results have been reported in other species (Medrano and Flexas, 2004; Gindaba et al., 2004).
Daily leaf temperatures showed a similar behavior as that of transpiration (data not shown) in the rainy and transitional periods, so the higher the temperature, the higher the transpiration rate (0800-1000 HR leaf temperature 31°C, E 15.1 µg H2O cm-2 s-1; 1000-1200 HR leaf temperature 33°C, E 18.5 µg H2O cm-2 s-1), in the dry season the opposite was seen, the temperatures rose toward noon and transpiration decreased (0800-1000 HR leaf temperature 32.5°C, E 1.9 µg H2O cm-2 s-1; 1000-1200 HR leaf temperature 36.8°C, E 1.2 µg H2O cm-2 s-1). In the rainy and transitional periods, when there was water availability, higher temperatures appeared with a lower relative humidity, generating a greater potential gradient and greater vapor pressure deficit that triggered increased transpiration, but in the dry season, a high vapor pressure deficit together with low soil moisture generated stomatal closure and decreased transpiration (Tab. 1).
Stomatal opening in G. arborea (Fig. 1, right) in times of increased water loss is considered by some authors as an indicator of the absence of water stress, as the plants would control their transpiration in response to a water deficit by decreasing stomatal conductance (Rabaioli and Rebello, 2007). This agrees with the findings of Osonubi and Davies (1980a, b) who referenced maintaining open stomata due to an insensitivity to a vapor pressure deficit.
In the daily cycle, it was observed that even at the point where the water potential was the most negative, (10001200 HR adult and juvenile seedlings 1000-1400 HR) (Fig. 2), no reduction was generated in transpiration in the rainy and transitional periods, on the other hand, this was when the highest rates of transpiration were found, due to a temporary imbalance between differences in root absorption rates, xylem transport and water loss rate through transpiration (Medrano and Flexas, 2004). The -1.5 MPa value was rarely reached by G. arborea in the rainy and transitional periods, and in the dry season values of leaf water potential of -2.6 to -2.8 MPa were observed, which generate stomatal closure in seedlings. Johnson and Ferrell (1983), in Pseudotsuga menziessi trees, found minimum values of -1.5 MPa in plants under conditions of good irrigation, while under water deficit conditions, found values of -3.0 MPa. The direct response of stomata to changes in leaf water potential must significantly impact the ability of G. arborea to withstand long periods of drought associated with high evaporative demands, this behavior would be advantageous allowing more efficient use of water and survival as the availability of water in the soil decreases, as described for other species (Pinheiro et al., 2005). Although the trends of increasing transpiration usually match increased PAR and decreased water potential, in certain cases the trend was not clear, indicating that other factors are probably involved with the control of stomatal closure in G. arborea, such as CO2 and chemical signals (Farquhar and Sharkey, 1982).
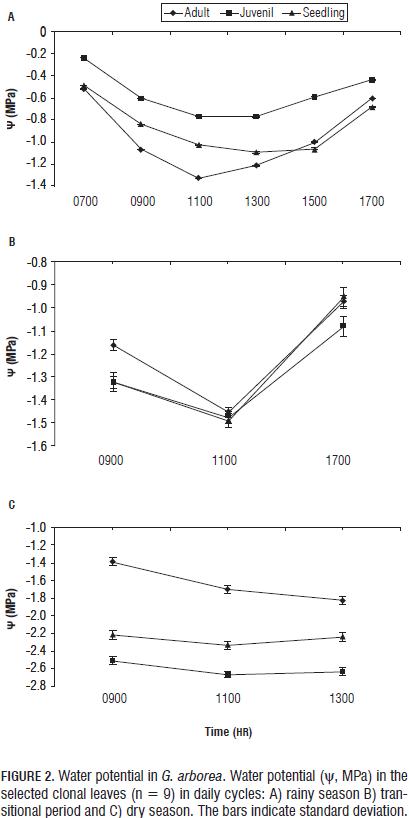
Water potential measurements carried out in the predawn during the dry season with values below-1.2 MPa indicated that a certain degree of water stress may be generated due to a water deficit in G. arborea.
According to the data obtained, G. arborea behaves like an isohydric or anisohydric plant, depending on the existing conditions (McDowell et al., 2008). For anisohydric plants, when water availability is high, transpiration reaches high values and leaf water potential values are very low with decreases in soil water potential, in the case of isohydric plants, when water availability is low, transpiration values decrease, leaf water potential values remain constant over the course of the day (Tardieu and Simonneau, 1998; McDowell et al., 2008) and potential values are not more negative at noon.
Chlorophyll A, B, and total carotenoids
In G. arborea, reductions in levels of chlorophylls and increased carotenoids were observed with increasing water deficit during the climatic periods (Fig. 3). Patterns similar to those obtained in chlorophyll have been reported in other plant species under water stress (Nayyar and Gupta, 2006; Sircelj et al., 2005; Jung, 2004). It was also found that the chlorophyll content was higher in seedlings, followed by juveniles and finally adults, which means that chlorophyll content is also dependent on age. The carotenoid content (Fig. 4) increased with an advancing water deficit, showing an energy dissipation strategy via the xanthophyll cycle (Demming-Adams and Adams, 1996; Müller et al., 2006), and regulation of the nitrogen and carbon content at the expense of chlorophyll as a source of carbon and nitrogen (Tanaka and Tanaka, 2007).
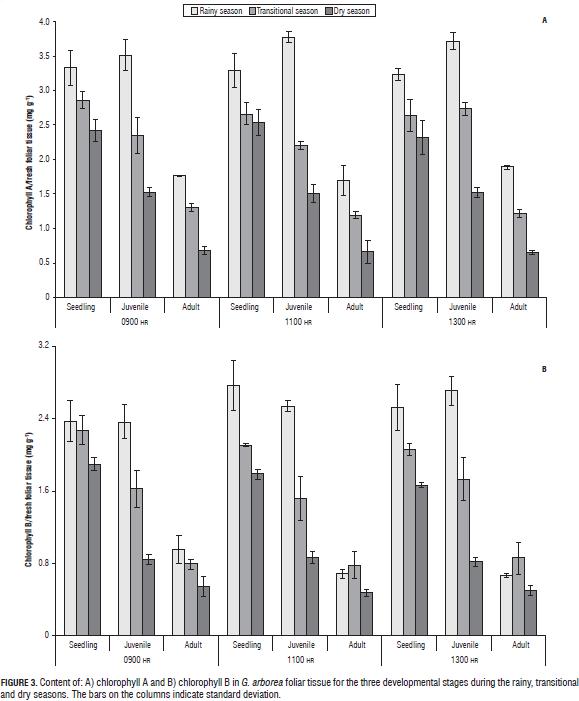
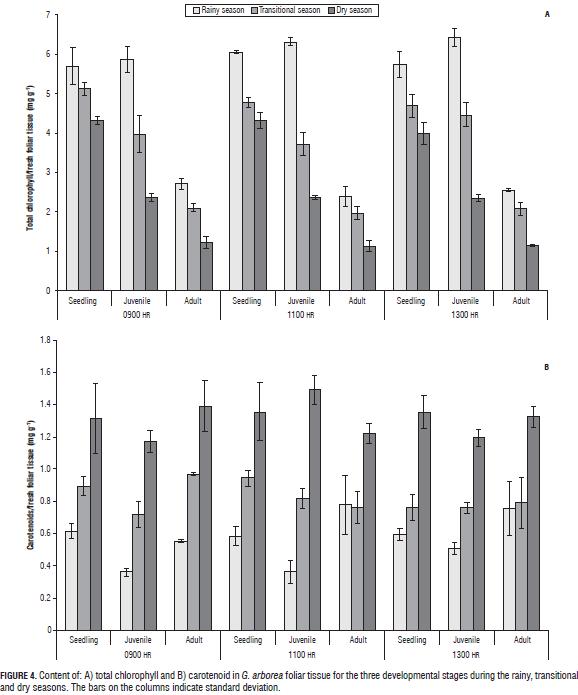
Conclusions
Transpiration rates decrease with the age of the tree, additionally, in the daily cycle, water potential is more negative at the times with higher transpiration rates, generating a water gradient which is influenced by environmental factors.
Pigment determination can be used as physiological indicators of plant responses to water deficit conditions in G. arborea because they give information about the stress event; as water stress increases, an inverse relationship between chlorophyll and carotenoid content is seen.
Acknowledgements
This work was funded by the Ministerio de Agricultura y Desarrollo Rural (Ministry of Agriculture and Rural Development) (contract 2007K7494-943-IICA-UNAL MADR 057/2007), División de Investigaciones Bogotá DIB, Universidad Nacional de Colombia, and Pizano.
Literature cited
Breda, N., R. Huc, A. Granier, and E. Dreyer. 2006. Temperate forest trees and stands under severe drought: a review of ecophysiological responses, adaptation processes and long-term consequences. Ann. For. Sci. 63, 625-644. [ Links ]
Barradas, V.L., E. Nicolás, A. Torrecillas, and J.J. Alarcón. 2005. Transpiration and canopy conductance in young apricot (Prunus armenica L.) trees subjected to different PAR levels and water stress. Agric. Water Manage. 77, 323-333. [ Links ]
DaMatta, F.M. 2003. Drought as a multidimensional stress affecting photosynthesis in tropical tree crops. Adv. Plant Physiol. 5, 227-265. [ Links ]
Delzon, S. and D. Loustau. 2005. Age-related decline in stand water use: sap flow and transpiration in a pine forest chronosequence. Agric. Forest Meteorol. 129, 105-119. [ Links ]
Demming-Adams, B. and W. Adams. 1996. The role of xanthophyll cycle carotenoids in the protection of photosynthesis. Trends Plant Sci. 1, 21-26. [ Links ]
Elheringer, J.R. and K.S. Werk. 1986. Modifications of solar-radiation absorption patterns and implications for carbon gain at the leaf level. pp. 57-81. En: Givnish, T.J. (ed.). On the economy of plant form and function: Proceedings of the Sixth Maria Moors Cabot Symposium, Evolutionary Constraints on Primary Productivity, and Adaptive Patterns of Energy Capture in Plants. Cambridge University Press, London. [ Links ]
Farquhar, G.D. and T.D. Sharkey. 1982. Stomatal conductance and photosynthesis. Annu. Rev. Plant Physiol. 33, 317-345 [ Links ]
Gindaba, J., A. Rozanov, and L. Negash. 2004. Response of seedlings of two Eucalyptus and three deciduous tree species from Ethiopia to severe water stress. For. Ecol. Manage. 201, 119-129. [ Links ]
Johnson, J.D. and W.K. Ferrel. 1983. Stomatal response to vapour pressure deficit and the effect of plant water stress. Plant Cell Environ. 6, 451-456. [ Links ]
Jung, S. 2004. Variation in antioxidant metabolism of young and mature leaves of Arabidopsis thaliana subjected to drought. Plant Sci. 166, 459-466. [ Links ]
Kozlowski, T.T. and S.G. Pallardy. 1997. Physiology of woody plants. 2nd ed. Academic Press, San Diego, CA. [ Links ]
Lichtenthaler, H.K. 1987. Methods in enzymology, Colowick, S.P., N.O. Kaplan. Academic Press. San Diego, CA. [ Links ]
Martínez, T., J. Vargas, A. Muñoz, and J. López. 2002. Respuesta al déficit hídrico en Pinus leiophylla: consumo de agua y crecimiento en plántulas de diferentes poblaciones. Agrociencia 36, 365-376. [ Links ]
McDowell, N., W.T. Pockman, C.D. Allen, D.D. Breshears, N. Cobb, T. Kolb, J. Plaut, J. Sperry, A. West, D.G. Williams, and E.A. Yepez. 2008. Tansley review. Mechanisms of plant survival and mortality during drought: why do some plants survive while others succumb to drought? New Phytologist 178, 719-739. [ Links ]
Medrano, H. and J. Flexas. 2004. Respuesta de las plantas al estrés hídrico. pp. 253-286. In: Reigosa, M.J, N. Pedrol, and A. Sánchez-Moreiras. (eds.). La ecofisiología vegetal: una ciencia de síntesis. Editorial Thomson, Madrid. [ Links ]
Melgarejo, L.M., M. Romero, S. Hernández, J. Barrera, M.E. Sol- arte, D. Suárez, L.V. Pérez, A. Rojas, M. Cruz, A.L. Moreno, S.C. Crespo, and W.H Pérez. 2010. Experimentos en fisiología vegetal. Universidad Nacional de Colombia, Bogota. [ Links ]
Müller, M., I. Hernandez, L. Alegre, and S. Munné-Bosch. 2006. Enhanced a-tocopherol quinone levels and xanthophyll cycle de-epoxidation in rosemary plants exposed to water deficit during a Mediterranean winter. J. Plant Physiol. 163, 601-606. [ Links ]
Nayyar, H. and D. Gupta. 2006. Differential sensitivity of C3 and C4 plants to water deficit stress: Association with oxidative stress and antioxidants. Environ. Exp. Bot. 58, 106-113. [ Links ]
Osonubi, O. and W.J. Davies. 1980a. The influence of water stress on the photosynthetic performance and stomatal behaviour of tree seedlings subjected to variation in temperature and irradiance. Oecologia 45, 3-10. [ Links ]
Osonubi, O. and W.J. Davies. 1980b. The influence of plant water stress on stomatal control of gas exchange at different levels of atmospheric humidity. Oecologia 46, 1-6. [ Links ]
Pinheiro, H.A, F.M. DaMatta, A.R.M. Chaves, M.E. Loureiro, and C. Ducatti. 2005. Drought tolerance is associated with rooting depth and stomatal control of water use in clones of Coffea canephora. Ann. Bot. 96, 101-108. [ Links ]
Rabaioli, L.G. and L. Rebello. 2007. Water relations of tree species growing on a rock outcrop in the "Parque stadual de Itapua" RS. Rev. Bras. Bot. 30, 703-711. [ Links ]
Sircelj, H., M., Tausz, D. Grill, and F. Batic. 2005. Biochemical responses in leaves of two apple tree cultivars subjected to progressing drought. J. Plant Physiol. 162, 1218-1308. [ Links ]
Tanaka, R. and A. Tanaka. 2007. Tetrapyrrole Biosynthesis in Higher Plants. Annu. Rev. Plant Biol. 58, 321-346. [ Links ]
Tardieu, F. and T. Simonneau. 1998. Variability among species of stomatal control under fluctuating soil water status and evaporative demand: modelling isohydric and anisohydric behaviours. J. Exp. Bot. 49, 419-432. [ Links ]