Services on Demand
Journal
Article
Indicators
-
Cited by SciELO
-
Access statistics
Related links
-
Cited by Google
-
Similars in SciELO
-
Similars in Google
Share
Agronomía Colombiana
Print version ISSN 0120-9965
Agron. colomb. vol.33 no.2 Bogotá May/Aug. 2015
https://doi.org/10.15446/agron.colomb.v33nv33n2.51546
Doi: 10.15446/agron.colomb.v33nv33n2.51546
1 Department of Agronomy, Faculty of Agricultural Sciences, Universidad Nacional de Colombia. Bogota (Colombia). gfischer@unal.edu.co
2 Division Urban Plant Ecophysiology, Faculty of Life Sciences, Humboldt-Universität zu Berlin. Berlin (Germany).
3 Research and Development, Compo Expert GmbH. Munster (Germany).
Received for publication: 26 April, 2015. Accepted for publication: 30 June, 2015.
ABSTRACT
Although the cape gooseberry has become the second most important export fruit in Colombia, information is scarce for its carbohydrate partitioning, which plays a major role in plant productivity. Seed-propagated Colombia ecotypes were kept in a greenhouse in 2.5-L plastic containers filled with washed quartz sand and were ferti-irrigated. The plants were pruned to one main vegetative stem with two generative stems. Dry matter (DM) partitioning during the initial plant growth showed the highest accumulation rate in the roots during the first 20 days, whereas, at a later stage of development, the shoot DM gain was higher and the leaf DM gain was lower than that of the roots. Sixty days after transplant, the plant parts were quantified and analyzed for glucose, fructose, sucrose, and starch. The roots were the largest carbohydrate pool for starch, but the sucrose content was lower in the roots than in the vegetative stem and the lower part of the reproductive stems. At 5-15 cm of the vegetative stem base, 6.4 mg of starch, 1.4 mg of monosaccharides and 5.3 mg/100 g of DM sucrose were found, indicating that this lower organ is also important for starch accumulation and, especially, for sucrose transport. In the two reproductive stems, the starch contents were much higher in the base part than in the apical part; the same relationship was found in the leaves. The monosaccharide content was the highest in the apical stem position with 8.2 mg/100 g DM. In contrast, the apical-positioned 10-day-old fruits had maximum starch concentrations (11.6 mg/100 g DM), possibly due to the assimilatory starch from green fruit photosynthesis, whereas the mature basal fruits (60-day-old) mainly accumulated sucrose (25.7 mg) and monosaccharides (21.2 mg/100 g DM).
Keywords: photosynthates, starch, sucrose, glucose, fructose.
RESUMEN
Aunque la uchuva se ha convertido en la segunda fruta de importancia en la exportación de Colombia, existe escasa información sobre el papel que juega el reparto de carbohidratos en la productividad de la planta. En invernadero, plantas del ecotipo Colombia, propagadas por semilla, se cultivaron en materas de 2,5 L, en arena de cuarzo lavada y con ferti-irrigación. Las plantas fueron podadas hasta el tallo vegetativo principal con dos tallos reproductivos. La distribución de materia seca (MS) durante los primeros 20 días de la planta mostró la mayor tasa de acumulación en raíces, mientras que en la etapa posterior la ganancia de MS fue mayor en tallos y menor en hojas que en las raíces. Sesenta días después del trasplante, se determinó el contenido de glucosa, fructosa, sacarosa y almidón para cada parte de la planta. Las raíces fueron el mayor vertedero de almidón, pero los contenidos de sacarosa fueron bajos tanto en el tallo vegetativo como de los reproductivos. Entre 5-15 cm de la base del tallo vegetativo se encontró un 6,4 mg de almidón, 1,4 mg de monosacáridos y 5,3 mg/100 g MS de sacarosa, indicando lo importante que es este órgano basal para la acumulación de almidón y, especialmente, para el transporte de sacarosa. En los dos tallos reproductivos, el contenido de almidón fue mayor en la base que en el ápice; la misma relación se encontró en las hojas. El contenido de monosacáridos fue mayor en la zona apical del tallo reproductivo, con 8,2 mg/100 g MS. Por el contrario, en los frutos apicales y pequeños, con 10 días de edad, se encontró los máximas concentraciones de almidón (11,6 mg/100 g MS), posiblemente se trata de almidón primario de la fotosíntesis de los frutos verdes, mientras que los maduros basales (60 días de edad) acumularon principalmente sacarosa (27,7 mg) y monosacáridos (21,2mg/100 g MS).
Palabras clave: productos fotosintéticos, almidón, sacarosa, glucosa, fructosa.
Introduction
The cape gooseberry (Physalis peruviana L.) originated in the Andean zones and, according to Legge (1974), probably Peru. It belongs to the Solanaceae family, in which the Physalis genus comprises between 75 and 90 species (Whitson and Manos, 2005) that develop their fruit in an inflated calyx (or husk). From Chile to Colombia, it grows wild and half-wild in frost-free zones between 800 and 3,000 m a.s.l. (FAO, 1982), where conditions are favourable for growth all year long. In Colombia,the best commercial growing conditions are found at altitudes between 1,800 and 2,800 m a.s.l. (13-16°C mean temperature, 70-80% relative humidity, and 1,000-1,800 mm annual rain fall) (Fischer et al., 2014).
In Colombia, the cape gooseberry is not only an important source of vitamins (A, C) for highland inhabitants (Fischer et al., 2007), but has also become an important export fruit, occupying second place after bananas (Fischer and Miranda, 2012).
The cape gooseberry is a semi-woody, perennial, shrubby plant (1 to 1.50 m tall) with a sympodial type of ramification, mainly of the dichasial pattern, generally forming four reproductive and fruiting stems (Fischer and Miranda, 2012). It has an indeterminate growth habit where the branches continue to grow; thus, the vegetative and reproductive organs develop simultaneously (fruits grow in each stem node, initiating at the first dichasial bifurcation, after 8 to 12 nodes of the vegetative stem) (Fischer et al., 2011; Ramírez et al., 2013).
The leaves (5-15 cm long and 4-10 wide) are soft, hairy, heart-shaped, peciolated and inserted alternately on the vegetative stem, where, on the reproductive stems, generally two leaves per node emerge; while, the bell-shaped, hermaphrodite yellow flowers, with dark purple-brown spots in the throat, are formed in the leaf axils(Fischer, 2000; Fischer et al., 2011). The leaf-like calyx, which completely encloses the ripening fruit, grows to a bladder-like organ (at fruit maturity becomes papery), measuring about 5 cm, with a round or elongated shape (Fischer et al., 1997).
Cape gooseberry fruits can be characterized as nearly round, glossy yellow berries with many flat seeds (150 to300 corns/fruit) and measure 1.25 to2.50 cm in diameter and weigh about 4 to 10 g, which take 60 to 80 d to mature (Fischer, 2000). The calyx completely encloses the fruit during its development (Fischer et al., 2011). During the first 20 d of fruit development, the calyx plays an important role in both the build-up and translocation of non-structural carbohydrates, mainly sucrose, for the fruit (Fischer and Lüdders, 1997).
Carbohydrates (CH) are produced by photosynthesis and used for growth and energy (Lakso and Flore, 2003); thus, Goldschmidt (1999) stated that CH levels probably are involved in the regulation of plant metabolic and developmental events. Ninety percent of plant dry matter (DM) is the result of photosynthesis (Daie, 1985); consequently, the accumulation of CH during the growing season is essential for the survival of plants (Kozlowski and Pallardy, 1997a). Carbohydrates comprise about three-fourths of the dry weight (DW) of woody plants and are the primary storage compounds and the organic substances from which most other organic compounds are synthesized (Pallardy, 2008).
Carbohydrate partitioning to the plant organs is responsible for the amount and pattern of plant growth and yield (Lakso and Flore, 2003). Thus, Engels et al. (2012) stated that the partitioning of biomass among plant organs and its mechanism of control are of vital importance in plant production. Translocation depends on the developmental stage of the plant (Fischer et al., 2012) and the direction and volume of transported CH are influenced by sink position and attraction strength (Fischer and Friedrich, 2000). During the reproductive phase of the cape gooseberry, Salazar et al. (2008) found partitioning coefficients of 0.09, 0.23 and 0.69 for leaves, stems, and fruit, respectively; that is, 69% of the total daily DM growth was allocated to the mass growth of fruits.
Oliveira and Priestley (1988) stated that, in fruit plants, soluble carbohydrates are composed of monosaccharides(normally glucose and fructose) and oligosaccharides (mainly sucrose); whereas, insoluble carbohydrates are made up of starch and hemicelluloses (DeJong and Ryugo, 1998).
Typically, and in nearly all plant species, sucrose is the most important transport form of CH (Lemoine et al., 2013; Loescher et al., 1990), making up over 95% of the DW of the material translocated in the sieve tubes of the phloem (Pallardy, 2008). In species of the Rosaceae family, as deciduous fruit trees, sugars are mainly transported by the sugar alcohol sorbitol (Kanayama and Kochetov, 2015); but also, in some cases, mannitol and oligosaccharides of the raffinose family are translocated (Lemoine et al., 2013).
Photosynthate-producing and exporting organs in the plant (mainly fully-expanded leaves) are known as sources and the importing organs are recognized as sinks (developing organs and non-photosynthetic organs such as immature leaves, fruits, roots, flowers, and tubers) (Taiz and Zeiger, 2010). The importance of various sinks may shift during plant development; whereas, the root and shoot apices are usually the major sinks during vegetative growth, fruits generally become the dominant sinks during reproductive development, particularly for the adjacent and other nearby leaves (Taiz and Zeiger, 2010).
Leaf photosynthesis and remobilization of carbohydrate reserves are the source for assimilates supplied to the fruit (Friedrich and Fischer, 2000). During their development, fruits accumulate carbohydrates, generally as starch, sucrose, or hexose sugars (Pallardy, 2008; Sugiyma et al. (1991) found that, in mature cape gooseberry fruits, sugar components are sucrose, glucose and fructose, as seen in the strawberry and other fruits (Macías-Rodríguez et al., 2002).
Contents and types of stored CH vary seasonally and in different organs and tissues, so that Kozlowski and Pallardy (1997a) considered starch as the most important reserve of CH; starch has often been used as the sole indicator of the CH status of plants (Pallardy, 2008). Starch is accumulated when a high level of sugars is built up or, in tissues where the non-structural CH content is low, it is converted to sugars (Pallardy, 2008). The reserve CH are very important for regrowth, e.g. after the cold winter season or following the pruning of shoots (Kozlowski and Pallardy, 1997a), which constitutes a great demand for reserve CH during flowering and fruiting (Monerri et al., 2011). Starch-sugar conversions in both vegetative and reproductive tissues commonly occur (Kozlowski and Pallardy, 1997a).
In recent decades, CH economy has been studied in many other species, using model plants, mainly at the quantitative level; cellular and biochemical CH systems have also been examined (Goldschmidt et al., 1999), but, for the cape gooseberry, less knowledge exists on CH partitioning between plant organs. Therefore, in this study, an attempt was made to clarify the pattern of CH accumulation within the plants; the results can serve as a basis for selection and plant productivity programmes.
Material and methods
This experiment was carried out in the glasshouse of the Division of Fruit Science, Humboldt-Universität zu Berlin. The seed propagated ecotype 'Colombia' was originated from native plants in the mountainous Boyaca region (2,000-2,800 m a.s.l.) of Colombia.
One month after sowing in intermittent mist chambers, vigorous plants were transplanted in 9-10 cm diameter pots and, 6 weeks later, in 2.5 L black plastic containers. The substrate used in the propagation and culture was washed quartz sand (calibre 0.7-1.2 mm). The plants were triple-irrigated daily with a 300-500 mL/pot Wuxal® solution, which had a composition of 8:8:6 (N:P:K; 0.24-0.40 g total N and K2O, and 0.18 g P2O5 per pot), depending on the development and climate conditions. Calcium and magnesium were applied with tap water.
The plants were kept on cement tables and trained in the following way:
- Pruned to one main vegetative stem with two fruiting productive stems (Fig. 1),
- Lateral branches of the fruiting stems cut after the first node,
- Leaders were supported by threads connected to the glasshouse construction.
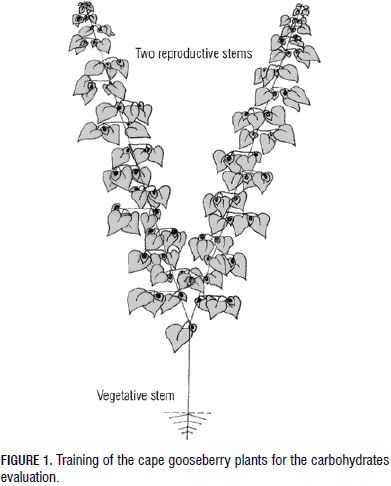
Mean minimum and maximum air temperatures in the glasshouse were 18 and 26°C. Relative humidity oscillated between 60 and 90%.
The treatments were arranged in a completely randomized design with a 3x3 factorial arrangement and six replicates (single plants). The data were analyzed by using the SAS® program.
The fruit setting date was registered and, at the end of the experiment, the fruit weight and carbohydrates were analysed in relation to the fruit age. When five basal fruits per plant reached their maturity (80 d after transplanting), so that the two fruiting stems represented all of the development stages, the plants were harvested.
For the CH analysis, samples were taken from the roots (completely), the first 5-15 cm of the basal vegetative stem, the first 5-15 cm of the two reproductive stems, and 10-20 cm measured from the apical top of the reproductive stems. The plant parts were shock-frozen in liquid nitrogen immediately after excision from the plant (between 10.00 and 11.00 a.m.) in order to completely stop the metabolic processes. After the freeze drying, the samples were finely ground in an agate mill. The samples were submitted to extraction five times with 80% ethanol at 60°C; the extractions were combined and the pellet separately prepared for the starch analysis. The glucose, fructose, sucrose, and starch were assayed enzymatically based on the methodology of Bergmeyer (1977) and Boehringer AG (1989) and determined spectrophotometrically at 340 nm with a Shimadzu UV‐1601spectrophotometer (Schimadzu Scientific Instruments, Columbia, MD).
The results are presented in a descriptive manner, using line (Fig. 2) and bar graphs (Fig. 3, 4 and 5) that include the standard error (SE) of each average. Six replicates were used and the experimental unit was one plant.
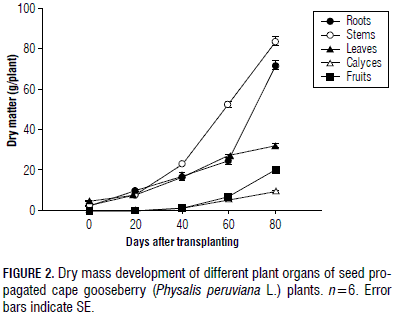
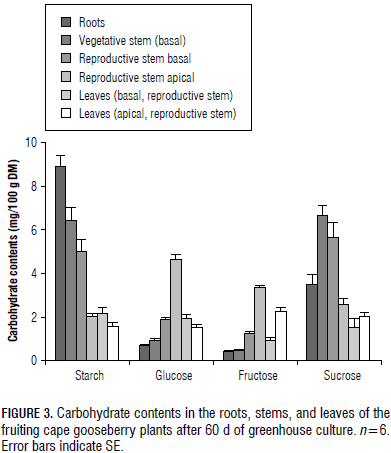
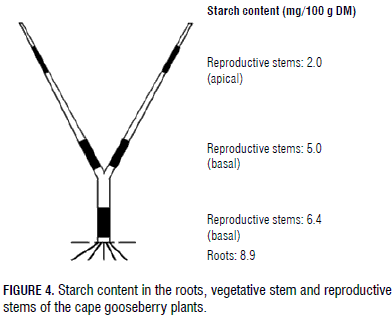
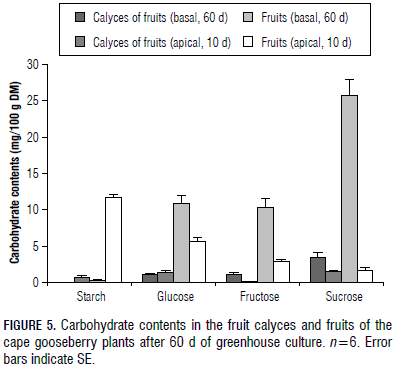
In this study, the CH distribution was expressed as a percent of DW of various tissues (organs), which can be misleading, in some cases, because high concentrations of carbohydrates often occur in tissues that comprise a low proportion of the total DW of a plant.
Results and discussion
Dry mass of plant organs
The development of DM of different plant organs shows the predominant role of the stem growth (34.5% of total plant DM), followed by the root, while the leaf DM increased constantly, but on a lower scale, and the fruit DM accumulation, with 20.46 g, reached only 9.9% of the total plant DM at 80 dat (Fig. 2). The fruit setting began 1 month after transplant.
The higher accumulation of DM in the stems of this semi-lignified plant shows the important formation of the supporting structure of the plant that is required prior to fruiting (Gardner et al., 1985) and, supposedly, for building sufficient tissues for storing CH reserves in this perennial plant. In the cape gooseberry, shoot growth is of special interest because, in each node of the reproductive stems, one fruit develops (Fischer, 2000). The second highest accumulation of plant DM in the root system highlights the importance of this organ, where absorbance of water and nutrients and growth hormone synthesis are crucial for the aerial part of the plant at this initial stage of development (Pallardy, 2008). The pattern of DM partitioning coincided with those mentioned by Lakso and Flore (2003); that is, young tree shoots and roots receive significant quantities of CH, as required by the development and construction of both the top and the subterranean part of the tree. The reduction of the percentage leaf DM from 60 to 80 d (24.6 to 15.5%) indicated the significance of leaf CH for the fruit filling (Schumacher, 1989) because these organs augmented their contribution from 6.3% to 9.9% at this period of time (Fig. 2).
Plant carbohydrate content
In the cape gooseberry, a species with an indeterminate growth habit (Fischer et al., 2011), the plant tries to maintain an equilibrium, attending to the vegetative and reproductive sinks simultaneously, avoiding excessive growth and development in one part (Gardner et al., 1985), which was achieved in our experiment through the removal of the basal lateral shoots and pruning of the plant top to eliminate apical dominancy (Fig. 1).
Roots
A starch content of 8.9 mg/100 g DM was measured in the root DW (Fig. 3 and 4), the highest proportion of the vegetative part of the cape gooseberry plant, but sucrose in this organ (3.5 mg/100 g DM) was lower than in the vegetative stem (5.3 mg/100 g DM) and the basal part of the generative stem (4.1 mg/100 g DM) (Fig. 3), possibly the sucrose had been interconverted to starch in this subterranean organ (Macías and Rodríguez, 2002), but, in general, starch and sucrose were the main CH found in the roots, confirming that CH reserves consist of both soluble and insoluble substances (Loescher et al., 1990).
Also, Kozlowski and Pallardy (1997a) stated that high amounts of CH are stored in large perennial roots and fine roots; Loescher et al. (1990) concluded that uppermost CH reserves are stored in root tissues. In general, CH are used during the initiation, elongation, and thickening of roots, as well as in the growth of mycchorrizae and root nodules (Kozlowski, 1992).Stored CH in the roots are of special importance for flowering and fruiting in Valencia orange trees (Dovis et al., 2014). High starch accumulation in roots has been attributed by Wang and Camp (2000) to an excellent adaptation and increased fruit yields in strawberries.With increasing plant heights, from the roots to the apical fruiting stem, the percentage of starch content decreased (Fig. 3 and 4).
The monosaccharide (glucose + fructose) content of the root DW was 1.1 mg/100 g DM, the lowest one of all the measured plant parts (Fig. 3) because this organ is specialized in the storage of CH (Loescher et al., 1990), such as starch and sucrose. In general, the investment of assimilates in root growth is necessary due to leaf water and nutrient requirements (Gardner et al., 1985). Between one-third and two-thirds of all CH translocated to the roots are used in respiration (Lambers et al., 2002).
Dhonta et al. (2006) found that the root concentrations of starch or non-structural CH were not correlated positively with the posterior regrowth of alfalfa shoots but rather the total amounts of these CH. In the case of equally perennial cape gooseberry plants, the root amount of starch used as a reserve for the forthcoming vegetative and reproductive growth was 6.37 g/plant (data not shown) and, thus, was much higher than there serve CH in the basal part of the vegetative and reproductive stems. The root CH reserves are important and play a specific role in supplying substrates for new shoot growth and respiration (Loescher et al., 1990).
Vegetative and two reproductive stems
As in the development of the high DM content of the stems (Fig. 2), this was also confirmed by the elevated starch concentration in these plant organs (Fig. 3 and 4). Even though high, there was a reducing concentration of starch from the plant roots to the apical part of the fruiting stems (Fig. 3 and 4); the starch level was always higher at the stem base than in the middle or upper part of the stem or plant. As in trees (Haddad et al., 1995), this underlined the importance of storage tissues in the lower parts of the cape gooseberry plants, as seen in other perennial species that invest in storage deposits. Wardlaw (1990) affirmed that the stem can act as a temporary storage reservoir of CH in some species. Additionally, Loescher et al. (1990) stated that CH storage is commonly found in the perennial part of the plants, such as the roots and the stems.
On the other hand, as a semi-woody plant, the cape gooseberry has to expend quantities of photosynthates for the production of supporting and transporting shoot tissues. Large amounts of CH are used in the production of xylem and phloem mother cells, their division and differentiation into xylem and phloem cells, and the expansion of the cambial sheet (Pallardy, 2008).
In the vegetative and reproductive stems, nearly the same sucrose concentration as that of starch was found (Fig. 3), with sucrose being the most commonly transported sugar in sieve elements (Taiz and Zeiger, 2010). Its concentration was highest in the basal part of the vegetative stem with a reduction in the upper parts of the plant. This pattern indicates its importance in CH partitioning to the lower plant parts. Additionally, at the base of the reproductive stems, where the fruits are developing and maturing, the highest sucrose and third highest starch concentration were measured, which could also indicate that this stem CH content can support most of the fruit development, as Lapointe (1998) observed in the defoliated herbaceous perennial Trillium erectum.
As described by Scholefeld et al. (1985) for the apical plant part of avocadoes, where flowers, fruits and shoots are developing, the starch content diminished drastically in the apical stem of the cape gooseberry (Fig. 3). The elevated monosaccharide content in the apices (Fig. 3) could indicate a high use of non-reducing sugars in these growth processes.
Shoots compete with fruits for CH, but in the stage of rapid fruit growth, the latter have a much stronger sink capacity for the accumulation of reducing and non-reducing sugars (Fig. 4) through the higher amount of hormonal growth promoters than are contained in the shoots (Kozlowski and Pallardy, 1997b).
Leaves
The young leaves of the cape gooseberry plants had lower starch and monosaccharide contents, but tended to have a higher sucrose concentration than the basal and mature leaves of the generative stems (Fig. 3). Gardner et al. (1985) supposed that the higher sucrose content in the upper leaves is due to their demand to import photoassimilates for energy and carbon skeletons, which are used in growth and development until these leaves produce enough photoassimilates to provide their own requirements.
In the apical shoot part, only very small fruits (Fig. 1) that could demand photosynthates and encourage photosynthesis grew (Schumacher, 1989). Young (sink) leaves import CH for constructing their own photosynthetic system; whereas, mature (source) leaves, due to their high photosynthetic activity, distribute photosynthates to sink organs (Araya et al., 2006). Additionally, the leaf CH can play an important role in the stomata aperture (Silber et al., 2013).
Bresinski et al. (2008) estimated that 30% of leaf phototosynthetic products will be used in the chloroplasts for starch synthesis, this assimilatory starch is broken down in the dark to glucose and maltose; the latter is exported to the cytoplasm, where it is used for sucrose synthesis. In consequence, the lower starch content of the younger leaves (Fig. 3) could indicate that they had relatively lower photosynthesis and carbohydrate storage rates than the mature basal leaves, where the ripening fruits demand higher transport rates of CH and, therefore, the photosynthetic activity increased and originated higher amounts of primary starches, stored in these leaves (Barceló et al., 2001). Also, Moreira et al. (2013) reported for the 'Ponkan' mandarin that, to maintain high levels of CH in the mature leaves, itis of special importance to improve the quality of fruits. However, it has to be taken into account that high accumulation rates of non-structural CH in mature leaves can suppress photosynthesis, but not so much in young leaves (Araya et al., 2006).
In general, leaves are also important CH reservoirs as Klopotek and Kläring (2014) found in young tomato plants that accumulated and stored CH, such as soluble sugars and starch during low temperature conditions for 1 week and remobilised them later.
The partitioning of assimilates generally occurs to the sinks closest to the source; for example, upper leaves export principally to the shoot apex, as lower leaves do to the roots, and middle leaves do to both organs (Wardlaw, 1990). In various cases, it was found that the CH requirements for fruits were supplied by the nearest leaves, such as two to three leaves in kiwi, four leaves in the tree tomato, and the nearest seven leaves in pineapple and guava (Kozlowski and Pallardy, 1997b; Fischer et al., 2012). In the case of the cape gooseberry, each fruit grows with two adjacent leaves, inserted in the same node (Fischer, 2000), both are involved in the CH export to this fruit (Fischer and Lüdders, 1997).
Calyces
The sepals of cape gooseberry plants form a bladder-like enclosure, the calyx, a leaf-like photosynthetic organ of the fruit during its entire development (Fischer, 2000). We found that its behaviour was closely linked to the fruit CH content and, therefore, nearly no starch was found in the calyces (0.1%), enclosing ripe fruits, but some starch (0.7%) was measured in the husks of young fruits (Fig. 5). Likewise, as in the case of sucrose concentration in ripe fruits, it was also high in the adjacent calyx (3.5%) (Fig. 5) and the same close relationship was found between the monosaccharide content of these two organs (Fig. 5). This behaviour underlines the fact that, although the calyx appears morphologically and anatomically similar to a green leaf (Bresinski et al., 2008), its physiology and CH metabolism are more related to the fruits than to the leaves (Fischer and Lüdders, 1997).
Fruits
Fruits are reproductive structures with a major sink strength, as was observed by Kozlowski and Pallardy (1997b) for the rapid transport of large quantities of reserve CH, and provide photosynthates to growing fruits; consequently, when a plant develops a heavy fruit load, the fruit seems to have priority for the photosynthates export from most leaves (Hansen, 1977), which was confirmed, among others, in persimmon by Park (2011) and in 'Salustiana' orange by Monerri et al. (2011), when most of the tree CH was translocated to the maturing fruits. The principal use of carbohydrates in fruits is for DM accumulation, as was observed by Pavel and DeJong (1993) who calculated that 78% of the total CH requirement is used for DM accumulation and 22% is used for respiration in 'Cal Red'-peach fruits.
Considering that, in each node of fruiting cape gooseberry stems, one fruit develops (Ramírez et al., 2013), the apical fruits had a much higher starch concentration than basal (and ripe) fruits (Fig. 5). Probably, these immature and still-green fruits started photosynthesis, using their own C3 sun-type chloroplasts for photosynthesis (Blanke, 1990), producing assimilatory starch (Taiz and Zeiger, 2010). Although the photosynthetic rate per unit area of fruit surface is generally less than one-tenth per unit of leaf surface (Bollard, 1970), this process can supply enough energy to replace that used in respiration in this early fruit growth stage. Also, it has to be taken into account that ripe fruits convert the initial high amounts of starch into sugars (Pallardy, 2008; Schaffer et al., 1999; Fischer and Lüdders, 1997).
In the early stage of development, the fruits contained relatively low amounts of monosaccharides and sucrose than the ripe fruits (Fig. 5), which coincided with Schumacher (1989), who also stated that fruits, during their full development, consume more than 80% of the synthesized leaf assimilates. Whereas, in the immature and apical fruits, the starch content constituted the highest CH proportion (Fig. 5); in the ripe and basal fruits, this was quite the opposite, taking into account that, in most ripening fruits, starches are converted to sugars (Pallardy, 2008).
In ripe cape gooseberry fruits, the highest concentrations of sucrose (25.7%), glucose (19.8%), and fructose (18.8%) were accumulated (Fig. 5), considering that the ability of fruits to preserve so much CH in a soluble form is characteristic of mature fruit tissues (Fischer and Lüdders, 1997). The high amount of sucrose accumulation in cape gooseberry fruits is favourable from the point of view that this CH, as a disaccharide, and because of osmotic considerations, more efficiently stores CH than monosaccharides (Schaffer et al., 1999).
The pattern of non-structural CH in these greenhouse-ripened cape gooseberry fruits was similar to that of field-ripened ones in the Boyaca region of Colombia, where Fischer et al. (2007) measured, in the Colombian ecotype, 1,183.0 μg glucose, 1,195.6 μg fructose, and 2,895.3 μg/100 g of fresh weight sucrose content. Comparing the CH pattern of the cape gooseberry with that of another Solanacea fruit, the tomato, shows that the latter has a content that is quite the opposite because the sucrose content is generally lower than 5% (Schaffer et al., 1999).
Conclusions
This study clearly showed that the CH, which is accumulated in the greatest portion as starch, concentrations decreased gradually from the roots to the stem apex. In contrast, the sucrose content was highest in the vegetative stem and the base of the reproductive stems, indicating its importance as a transport sugar. An elevated monosaccharide content was measured in the apical stem part, where the meristem and initial leaf and fruit growth take place, but also within the mature fruits, developing at the base of the reproductive stems; high sucrose and monosaccharide contents were found. In general, in the semi-lignified and perennial cape gooseberry plant, a similar distribution pattern to that of woody plants was seen.
Literature cited
Araya, T., K. Noguchi, and I. Terashima. 2006. Effects of carbohydrate accumulation on photosynthesis differ between sink and source leaves of Phaseolus vulgaris L. Plant Cell Physiol. 47, 644-652. Doi: 10.1093/pcp/pcj033 [ Links ]
Barceló, J., G. Nicolás, B. Sabater, and R. Sánchez. 2001. Fisiología vegetal. Editorial Pirámide, Madrid. [ Links ]
Bergmeyer, H.U. 1977. Methoden der enzymatischen Analyse. Verlag Chemie, Weinheim, Germany. [ Links ]
Blanke, M.M. 1990. Photosynthesis in subtropical fruits - review paper. Acta Hort. 275, 435-439. [ Links ]
Boehringer AG. 1989. Methods of biochemical analysis and food analysis using test-combinations. Boehringer, Mannheim, Germany. [ Links ]
Bresinski, A., C. Körner, J.W. Ladereit, G. Neuhaus, and U. Sonnewald. 2008. Strasburger lehrbuch der botanik. Spektrum Adademischer Verlag, Heidelberg, Germany. [ Links ]
Bollard, E.G. 1970.The biochemistry of fruit and their products.Vol 1. Academic Press, London. [ Links ]
Daie, J. 1985. Carbohydrate partitioning and metabolism in crops. Hort. Rev. 7, 69-108. [ Links ]
Engels, C., E. Kirkby, and P. White. 2012. Mineral nutrition, yield and source-sink relationships. pp. 85-133. In: Marschner, P. (ed.). Marschner's mineral nutrition of higher plants. 3rd ed. Elsevier, Amsterdam, The Netherlands. Doi: 10.1016/B978-0-12-384905-2.00005-4 [ Links ]
Dhonta, C., Y. Castonguay, P. Nadeau, G. Bélanger, and F.-P.Chalifour. 2002. Alfalfa root carbohydrates and regrowth potential in response to fall harvests. Crop Sci. 42,754-765. Doi: 10.2135/cropsci2002.7540 [ Links ]
Dovis, V.L., E.C. Machado, R.V. Ribeiro, J.R. Magalhães Filho, P.E.R. Marchiori, and C.R.G. Sales. 2014. Roots are important sources of carbohydrates during flowering and fruiting in 'Valencia' sweet orange trees with varying fruit load. Sci. Hortic. 174, 87-95. Doi: 10.1016/j.scienta.2014.05.011 [ Links ]
FAO. 1982. Fruit bearing forest trees: technical notes. FAO Forestry Paper 34, 140-143. [ Links ]
Faust, M. 1989. Physiology of temperate zone fruit trees. John Wiley & Sons, New York, NY. [ Links ]
Fischer, G. 2000. Crecimiento y desarrollo. pp. 9-26. In: Flórez, V.J., G. Fischer, and A.D. Sora (eds.). Producción, poscosecha y exportación de la uchuva (Physalis peruviana L.). Unibiblos, Universidad Nacional de Colombia, Bogota. [ Links ]
Fischer, G., P.J. Almanza-Merchán, and D. Miranda. 2014. Importancia y cultivo de la uchuva (Physalis peruviana L.). Rev. Bras. Frutic. 36, 1-15. Doi: 10.1590/0100-2945-441/13 [ Links ]
Fischer, G., P.J. Almanza-Merchán, and F. Ramírez. 2012. Source-sink relationships in fruit species. A review. Rev. Colomb. Cienc. Hortic. 6, 238-253. Doi: 10.17584/rcch.2012v6i2.1980 [ Links ]
Fischer, G., G. Ebert, and P. Lüdders. 2007. Production, seeds and carbohydrate contents of cape gooseberry (Physalis peruviana L.) fruits grown at two contrasting Colombian altitudes. J. Appl. Bot. Food Qual. 81(1), 29-35. [ Links ]
Fischer, G., A. Herrera, and P.J. Almanza. 2011. Cape gooseberry (Physalis peruviana L.). pp. 374-396. In: Yahia, E.M. (ed.) Postharvest biology and technology of tropical and subtropical fruits. Vol. 2. Acai to citrus. Woodhead Publishing, Cambridge, UK. [ Links ]
Fischer, G. and P. Lüdders. 1997. Developmental changes of carbohydrates in cape gooseberry (Physalis peruviana L.) fruits in relation to the calyx and the leaves. Agron. Colomb. 14, 95-107. [ Links ]
Fischer, G., P. Lüdders, and F. Torres. 1997. Influencia de la separación del cáliz de la uchuva (Physalis peruviana L.) sobre el desarrollo del fruto. Rev. Comalfi 24, 3-16. [ Links ]
Fischer, G. and D. Miranda. 2012. Uchuva (Physalis peruviana L.). pp. 851-873. In: Fischer, G. (ed.). Manual para el cultivo de frutales en el trópico. Produmedios, Bogota. [ Links ]
Friedrich, G. and M. Fischer. 2000. Physiologische Grundlagen des Obstbaues. Ulmer Verlag, Stuttgart, Germany. [ Links ]
Gardner, F.P., R.B. Pearce, and R.L. Mitchell. 1985. Physiology of crop plants. Iowa State University Press, Ames, IA. [ Links ]
Goldschmidt, E.E. 1999. Carbohydrate supply as a critical factor for citrus fruit development and productivity. HortScience 34, 1020-1024. [ Links ]
Haddad, Y., D. Clair-Maczulajtys, and D. Bory. 1995. Effects of curtain-like pruning on distribution and seasonal patterns of carbohydrate reserves in plane (Platanus acerifolia Wild) trees. Tree Physiol. 15, 135-140. Doi: 10.1093/treephys/15.2.135 [ Links ]
Hansen, P. 1977. Carbohydrate allocation. pp. 247-255. In: Landsberg, J.J. and C.V. Cutting (eds.). Environmental effects on crop physiology. Academic Press, London. [ Links ]
Kanayama, Y. and A. Kochetov. 2015. Abiotic stress biology in horticultural plants. Springer, Tokyo. Doi: 10.1007/978-4-431-55251-2 [ Links ]
Klopotek, Y. and H.-P. Kläring. 2014. Accumulation and remobilization of sugar and starch in the lwaves of young tomato plants in response to temperature. Sci. Hortic. 180, 262-267. Doi: 10.1016/j.scienta.2014.10.036 [ Links ]
Kozlowski, T.T. 1992. Carbohydrate sources and sinks in woody plants. Bot. Rev. 58, 107-122. [ Links ]
Kozlowski, T.T. and S.G. Pallardy. 1997a. Physiology of woody plants. 2nd ed. Academic Press, San Diego, CA. [ Links ]
Kozlowski, T.T. and S.G. Pallardy. 1997b. Growth control in woody plants. Academic Press, San Diego, CA. [ Links ]
Lakso, A.N. and J.A. Flore. 2003. Carbohydrate partitioning and plant growth. pp. 21-30. In: Baugher, T.A. and S. Singh (eds.). Concise encyclopedia of temperate tree fruit. Food Products Press, New York, NY. [ Links ]
Lambers, H., O.K. Atkin, and F.F. Millenar. 2002. Respiratory patterns in roots in relation to their functioning. pp. 521-552. In: Waisel Y., A. Eshel, and U. Kafkafi (eds.). Plant roots the hidden half. Marcel Dekker, New York, NY. [ Links ]
Lapointe, L. 1998. Fruit development in Trillium. Dependence on stem carbohydrate reserves. Plant Physiol. 117, 183-188. Doi: 10.1104/pp.117.1.183 [ Links ]
Legge, A.P. 1974. Notes on the history, cultivation and uses of Physalis peruviana L. J. Royal Hort. Sci. 99, 310-314. [ Links ]
Lemoine, R., S. LaCamera, R. Atanassova, F. Dédaldéchamp, T. Allario, N. Pourtau, J.L. Bonnemain, M. Laloi, P. Coutos-Thévenot, L. Maurousset, M. Faucher, C. Girousse, P. Lemonnier, J. Parrilla, and M. Durand. 2013. Source-to-sink transport of sugar and regulation by environmental factors. Front. Plant Sci. 4, 272. Doi: 10.3389/fpls.2013.00272 [ Links ]
Loescher, W.H., F. McCamant, and J.D. Keller. 1990. Carbohydrate reserves, translocation, and storage in woody plant roots. HortScience 25, 274-281. [ Links ]
Macías-Rodríguez, L., E. Quero, and M.G. López. 2002. Carbohydrate differences in strawberry crowns and fruit (Fragaria x ananassa) during plant development. J. Agric. Food Chem. 50, 3317-3321. [ Links ]
Monerri, C., A. Fortunato-Almeida, R.V. Molina, S.G. Nebauer, A. García-Luis, and J.L. Guardiola. 2011. Relation of carbohydrate reserves with the forthcoming crop, flower formation and photosynthetic rate, in the alternate bearing 'Salustiana' sweet orange (Citrus sinensis L.). Sci. Hortic. 129, 71-78. Doi: 10.1016/j.scienta.2011.03.009 [ Links ]
Moreira, R.A., J.D. Ramos, M.d.C. Monteiro da Cruz, L. Araújo Pantoja, and A. Soares dos Santos. 2013. Leaf carbohydrates in 'Ponkan' mandarin fruit quality under chemical thinning. Acta Sci. Agron. 35, 349-356. Doi: 10.4025/actasciagron.v35i3.16219 [ Links ]
Pallardy, S.G. 2008. Physiology of woody plants. 3rd ed. Academic Press, San Diego, CA. [ Links ]
Park, S.J. 2011. Dry weight and carbohydrate distribution in different tree parts as affected by various fruit-loads of young persimmon and their effect on new growth in the next season. Sci. Hortic. 130, 732-736. Doi: 10.1016/j.scienta.2011.08.029 [ Links ]
Pavel, E.W. and T.M. DeJong. 1993. Relative growth rate and its relationship to compositional changes of nonstructural carbohydrates in the mesocarp of developing peach fruits. J. Amer. Soc. Hort. Sci. 118, 503-508. [ Links ]
Ramírez, F., G. Fischer, T.L. Davenport, J.C.A. Pinzón, and C. Ulrichs. 2013. Cape gooseberry (Physalis peruviana L.) phenology according to the BBCH phenological scale. Sci. Hortic. 162, 39-42. Doi: 10.1016/j.scienta.2013.07.033 [ Links ]
Salazar, M.R., J.W. Jones, B. Chaves, and A. Cooman. 2008. A model for the potential production and dry matter distribution of Cape gooseberry (Physalis peruviana L.). Sci. Hortic. 115, 142-148. Doi: 10.1016/j.scienta.2007.08.015 [ Links ]
Schaffer, A.A., M. Petreikov, D. Miron, M. Fogelman, M. Spiegelman, Z. Bnei-Moshe, S. Shen, D. Granot, R. Hadas, N. Dai, I. Levin, M. Bar, M. Friedman, M. Pilowsky, N. Gilboa, and L. Chen. 1999. Modification of carbohydrate content in developing tomato fruit. HortScience 34, 1024-1027. [ Links ]
Scholefeld, P.B., M. Sedgley, and D.McE. Alexander. 1985. Carbohydrate cycling in relation to shoot growth, floral initiation and development and yield in the avocado. Sci. Hortic. 25, 99-110. Doi: 10.1016/0304-4238(85)90081-0 [ Links ]
Schumacher, R. 1989. Die Fruchtbarkeit der Obstgehölze. Eugen Ulmer, Stuttgart, Germany. [ Links ]
Silber, A., Y. Israeli, M. Levib, A. Keinanb, G. Chudib, A. Golanb, M. Noyc, I. Levkovitcha, K. Narkisa, A. Naorb, and S. Assouline. 2013. The roles of fruit sink in the regulation of gas exchange and water uptake: a case study for avocado. Agr. Water Manage. 116, 21-28. Doi: 10.1016/j.agwat.2012.10.006 [ Links ]
Sugiyama, N., K. Roemer, and G. Bünemann. 1991. Sugar pattern of exotic fruits from the Hannover market, Germany. Gartenbauwiss. 56, 126-129. [ Links ]
Taiz, L. and E. Zeiger. 2010. Plant physiology. 5th ed. Sinauer Associates Publishers, Sunderland, MA. [ Links ]
Wang, S. and M. Camp. 2000. Temperatures after full bloom affect plant growth and fruit quality of strawberry. Sci. Hortic. 85, 183-199. Doi: 10.1016/S0304-4238(99)00143-0 [ Links ]
Wardlaw, I.A. 1990. The control of carbon partitioning in plants. New Phytol. 116, 341-381. Doi: 10.1111/j.1469-8137.1990.tb00524.x [ Links ]
Whitson, M. and P.S. Manos. 2005. Untangling Physalis (Solanaceae) from the Physaloids: A two-gene phylogeny of the Physalinae. Syst. Bot. 30, 216-230. Doi: 10.1600/0363644053661841 [ Links ]