Introduction
Cape gooseberry, Physalis peruviana L. is native to the Andes, grows between 1,500 and 3,000 m a.s.l. as a wild plant and as a crop between 1,800 and 2,700 m a.s.l. (Fischer et al., 2005). Colombia is the largest producer in the world, generating revenues of up to 31.7 million dollars per year and concentrating 90% of world production (Agronet, 2016). The extracts of the plant and its fruits are attributed with several anti-cancer and anti-inflammatory properties, due to the high content of antioxidants, vitamins, minerals, and fiber and its low-calorie content (Ramadana, 2015). Recently, in Colombia, two outstanding cultivars were registered for their fruit quality (ICA, 2016). However, cape gooseberry still requires improved cultivars to accurately control diseases. At the national level, the greatest limitation is vascular wilt (Enciso-Rodríguez et al., 2013; Osorio-Guarín et al., 2016), a disease caused by Fusarium oxysporum f. sp. physali (Simbaqueba et al., 2018), causing losses of up to 100% of production and yield (González and Barrero, 2011). In terms of quality, the cracking of the fruit stands out as the greatest limitation (Fischer et al., 2011; Valdenegro et al., 2013).
Several studies of phenotypic characterization of P. peruviana and related species from Colombian germplasm have found variability in the fruit descriptors. Among these, the research of Bonilla (2008) with 24 accessions from the provinces of Nariño, Valle del Cauca, Caldas, and Cundinamarca stands out. Morevoer, Trillos et al. (2008) identified that fruit cracking, calyx weight, and fruit number per plant were useful descriptors for the differentiation of 46 cape gooseberry accessions conserved in the Germplasm Bank for Food and Agriculture, Plant Subsystem, located at the Research Center "La Selva" of AGROSAVIA. Madriñan et al. (2011) determined that the Brix degrees, weight and fruit diameter allowed the differentiation of 29 introductions from the work collection of the Universidad Nacional de Colombia (Palmira campus). On the other hand, Herrera et al. (2012) identified diversity by weight, size, pH and maturity index in 54 accessions of the Center and NorthEast regions of Colombia.
Regarding the resistance response to Fusarium oxysporum f. sp. physali (Foph),González and Barrero (2011) used the highly virulent strain of the pathogen (MAP5) to evaluate 58 accessions of cape gooseberry and 12 related species from the collections of the Universidad Nacional de Colombia (Bogota campus), the Universidad de Nariño and AGROSAVIA. The authors identified accessions with different levels of phenotypic resistance response to the pathogen in plants under controlled conditions (Enciso-Rodríguez et al., 2013). From the accessions that showed different levels of phenotypic response comprising resistant (R), moderately resistant (MR) or susceptible (MS); seed multiplication was performed for further evaluation of field response with a high and no inoculum pressure of the Foph MAP5 strain (Rodríguez, 2013). Moreover, Osorio-Guarín et al. (2016) identified promising accessions with different degrees of resistance, as well as sixteen Single Nucleotide Polymorphisms (SNPs) markers associated with the resistance response.
The main objective of the present research was to evaluate fruit traits of commercial interest, i.e. yield, quality, physicochemical and antioxidant capacity in 14 accessions previously identified by carrying traits of interest, and investigate their relationship to the resistance response to Foph in order to identify promising genotypes for their direct use or as parentals for genetic improvement processes.
Materials and methods
The study was carried out at the Tibaitata Research Center of the Colombian Corporation for Agricultural Research, AGROSAVIA, located in the municipality of Mosquera (Cundinamarca), at 4°42'00" N and 74°12'00" W, at 2,543 m a.s.l., cold thermal floor (Fa). The experiment was carried out in the field between September 2010 and September 2011 under the following climatic conditions: average temperature between 13 and 14°C, relative air humidity between 80 and 85%, precipitation between 34 and 198 mm/month and effective solar brightness between 2.6 and 4 h. The plants used in the experiment were previously germinated or hardened under greenhouse conditions in 1 kg bags with 50% husk: 50% soil substrate. They were grown and maintained in the greenhouse during 45 d. After that, they were moved to the field and planted in solarized soil contained in No.6 plastic bags with a capacity of approximately 25 kg (Rodriguez, 2013). Once planted, the plants were located on the ground and later positioned at a planting distance of 2 m between rows and 2 m between streets. The experiment comprised two lots (500 m2 each), one with high inoculum pressure of the MAP5 strain of Foph (1x105 cfu ml-1) sprayed directly to the soil, and a second one without pathogen inoculation. For each case, a completely randomized experimental design was implemented (Rodríguez, 2013). The inoculum for the experiment was produced according to the methodology proposed for Namiki et al. (1994) with some modifications. MAP5 isolate was grown in 2L Erlenmeyer flasks containing 500 mL of PDB medium during 10 d at 28°C in constant shaking. The culture was filtered using three layers of sterile gauze and conidial suspension was adjusted to the concentration mentioned above. This concentration was tested previously in greenhouse experiments under controlled conditions (Enciso et al., 2013).
In each lot, 30-31 genotypes from 14 P. peruviana accessions were evaluated. The accessions were provided by the Colombian Germplasm Bank for Food and Agriculture, Plant System (Tab. 1). Fertilization, cultural practices and pest and disease management were carried out as described in the Technical Manual for the management of the cape gooseberry crop (Zapata et al., 2002).
TABLE 1 Response of accessions from the cape gooseberry collection (P peruviana) evaluated after the infection process by Foph MAP5 strain under greenhouse and field conditions.
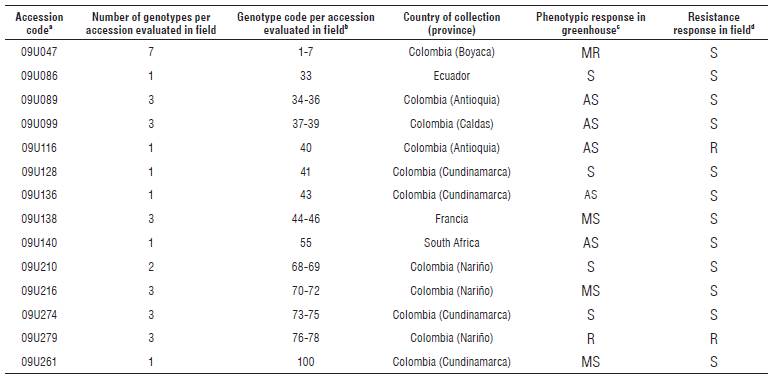
aCode assigned by the Germplasm Bank and plant subsystem to the accessions evaluated under conditions of high and no presence of Foph. bCode assigned to each genotype obtained by in vitro multiplication or self-pollination of accessions evaluated in greenhouse and later in the field.
cPhenotypic response in greenhouse according to Pulido (2010). AS: highly susceptible, S: susceptible, MS: moderately susceptible, MR: moderately resistant, R: resistant. dPhenotypic response in the field according to Rodríguez (2013).
The phenotypic response of the accessions evaluated under field conditions was recorded considering the disease severity variable. The symptoms monitoring was carried out monthly, adapting the scale of evaluation of vascular wilt symptoms in the greenhouse (Enciso et al., 2013). This scale is composed of 10 categories, where 0 groups the plants that show no symptoms of the disease and 9 those that have died from the infection caused by Foph. For this study, plants with values ≤4 on the severity scale were considered resistant and plants with values >4 were considered
Mayorga-Cubillos, Arguelles, Rodriguez, Almario, Arlza, and Barrero: Yield and physicochemical quality of Physalis peruviana L. fruit related to the resistance response against Fusarium oxysporum f. sp. Physali susceptible. Statistical analyzes were carried out using SAS software version 6.1 using a Chi-square test (X2) in order to determine the percentage of resistance of the evaluated accessions (Rodriguez, 2013). At 281 d after the establishment in the field, fruit harvest was started at an optimum degree of maturation, during three biweekly samplings. The fruits were classified according to the Colombian Technical Standard NTC 4580 (Icontec, 1999). Eighteen quantitative descriptors, with 5 fruits per descriptor, related to quality and yield were used (Herrera et al., 2012) (Tab. 2). The physicochemical variables were analyzed on export-type fruits (Icontec, 1999).
TABLE 2 Description of quantitative variables evaluated in the work collection of P. peruviana.
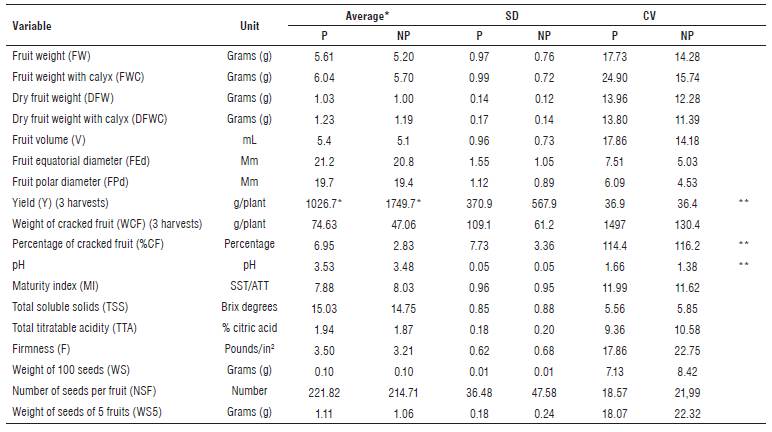
*Average of three harvests. **Statistically significant differences (P<0.05). SD: Standard deviation. CV: Coefficient of variation. P: high pressure of the pathogen. NP: no presence of the pathogen.
The fruit antioxidant capacity was evaluated on the genotypes 09U274-74 and 09U279-76 selected for their differential resistance response to Foph (Tab. 1). This variable was quantified using the methods ABTS (Antioxidant capacity of Trolox equivalents), DPPH (1,1 diphenyl-2-pyridylhydra-zyl) according to Rufino et al. (2007), FRAP (Antioxidant Power of Ferric Reduction) according to Benzie and Strain (1996), and ORAC (Absorbance Capacity of Oxygen Radicals) and total phenols according to Andre et al. (2007).
Data from the 18 descriptors were analyzed using the statistical software SAS version 9.4 (SAS Inst., Inc., Cary, NC). The antioxidant capacity was analyzed with the GLIMMIX procedure of the mentioned software, under a completely randomized design with a 2x2 factorial arrangement (two genotypes x two types of pathogen pressure). The mean comparison was performed using the Tukey test (P<0.05).
Results and discussion
Comparison of means of the variables analyzed
The t-Student test showed statistically significant differences (P<0.05) between the conditions of high pressure of the pathogen and no presence of the same, for the variables Yield (Y), Percentage of cracked fruit (%CF) and pH (Tab. 2). Regarding the comparison of means, genotypes with high pressure of the pathogen showed a decrease in Y of 723 g/plant, an increase in %CF of 4.12% and an increase in pH of 0.05. The above agrees with previous publications related to production losses caused by Foph (Zapata et al., 2002; González and Barrero, 2011).
For the materials without pathogen pressure, it was observed that the values for fruit equatorial diameter (FEd), fruit polar diameter (FPd), total soluble solids (TSS), total titratable acidity (TTA), fruit weight with calyx (FWC) and Y were placed within the standard ranges according to NTC 4580 (Icontec, 1999).
Principal component analysis (PCA)
The PCA for materials under high and no pathogen pressure accumulated 85.45% and 85.27% of the total variance, respectively, at the first five main components. For materials under high pathogen pressure, component one accumulated 40.5% of the variance, in which FEd, FPd, Fruit volume (V), FWC, Dry fruit weight with calyx (DFWC), Fruit weight (FW) and Dry fruit weight (DFW) were the variables of greatest importance to define this component. The component two that participated with 18.13% of the total variance was explained mainly by the variables: Weight of cracked fruit (WCF), %CF, Weight of seeds of 5 fruits (WS5), Number of seeds per fruit (NSF) and Y. The third component accumulated 11.45% of the variance, in which Firmness (F) and TTA were the variables with the highest contribution, while factors 4 and 5 contributed 8.98% and 8.64% of the variance, respectively (Tab. 3).
TABLE 3 Contribution in variance for each of the main components selected in materials under high and no pressure of the pathogen.
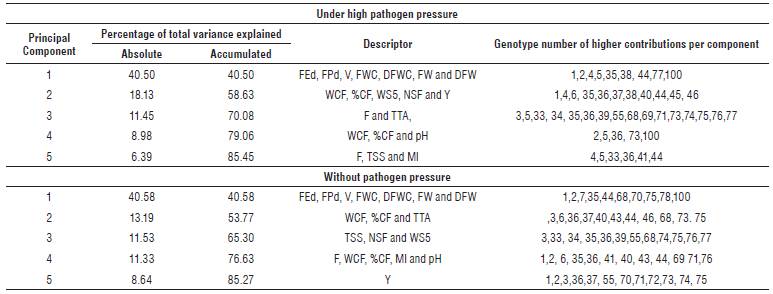
In the materials without the pressure of the pathogen, component number one included variables related to fruit diameter, volume, and weight, accumulating 40.58% of the total variability. Component two, which accumulated 13.19% of variability, was explained by the variables WCF, %CF, and TTA; therefore, it grouped genotypes of high fruit cracking and a high percentage of citric acid. The third and fourth components, with 11.53 and 11.33% respectively, were explained by variables related to fruit seeds, fruit cracking, maturity index (MI) and pH.
Criollo et al. (2001) worked with the cape gooseberry collection of the Universidad de Nariño and found that the fruit diameter, weight, and Brix degrees descriptors were the most important. These results also agree with those reported by Bonilla et al. (2008), Trillos et al. (2008), Herrera et al. (2012) and García-Arias et al. (2018). This indicates that national collections from different sources agree on the variables of greatest importance to discriminate the phenotypic variability associated with fruit cape gooseberry traits.
We found significant positive Pearson correlations for MI and TSS (0.54), as well as for V/FW and DFWC/DFW (0.98). Also, a negative correlation (-0.87) between MI and TTA was found. The above agrees with studies published by Herrera et al. (2012). Although these correlations are barely affected by the presence or absence of the pathogen in the plant (Toledo-Souza et al., 2012), they are of great help for future selection processes or morpho-agronomic characterization processes, since the positive relationships between traits may allow reducing the number of study variables such as the case of fruit volume (V) and fresh fruit weight (FP).
Cluster analysis
A cluster analysis was carried out based on the main components selected. Based on the dendrogram corresponding to the genotypes under high pathogen pressure (Fig. 1A), seven homogeneous groups of materials were generated. Group one was comprised by genotypes 1, 3, 34, 77, and 5, with materials 77 with high resistance response and material 1 with the highest Y (1975.40 g/plant) being the ones that stood out. The mean values of DFWC (1.31 g) and pH (3.56) were higher compared to the other groups. In group two, consisting of genotype 4, the variables Y (1543.10 g/ plant), FEd (23.40 mm) and FPd (20.80 mm) presented means above the general average of all materials (Tab. 4). Therefore, it is possible to propose the use of genotypes 1, 4 and 77 in breeding programs focused on yield, fruit size, and fruit quality.
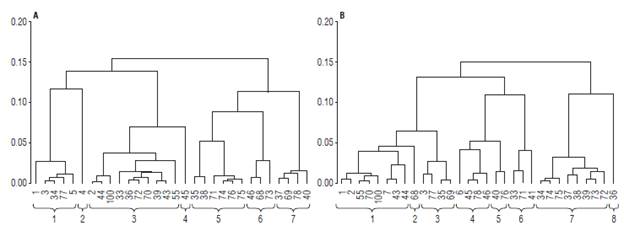
FIGURE 1 Dendrogram for materials under high pressure (A) and without the pressure of the pathogen (B). Coding: Field code (internal numbers of each group) according to Tab. 1. External horizontal numbers of the brackets indicate the number of clusters.
TABLE 4 Mean of the variables for each group conformed by cluster analyses, for genotypes with high pressure of the pathogen.
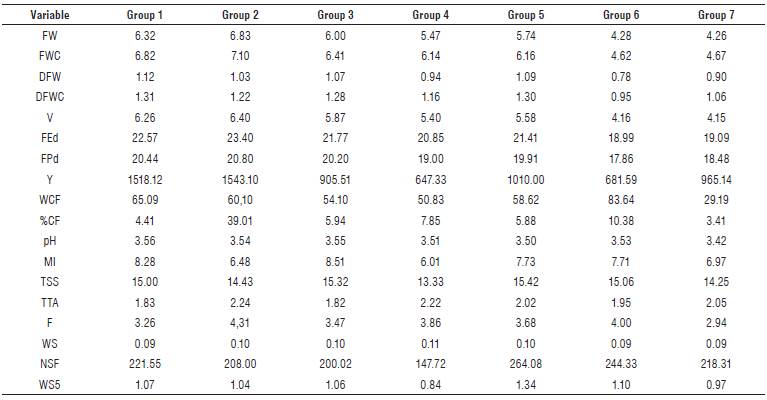
Group three comprised genotypes 2, 44, 100, 33, 36, 72, 70, 39, 43, and 55, with high MI (8.51), which is higher than the general average (7.88), and low TTA (1.82%) (Tab. 4). Group four showed the lowest mean for Y with 647.33 (g/ plant). Group five harbored genotypes 35, 38, 71, 74, 75, and 76, the latter with high resistance response in the field (Tab. 1). These materials also had high TSS (15.42 °Brix), a feature of great interest for industrial processes.
Group six, composed of genotypes 46, 68 and 73, included materials with high CF (83.64 g/plant), compared to the general average (74.63 g/plant). On the other hand, the values for FPd (17.86 mm) and FEd (18.99 mm) were the lowest within the materials under high Foph pressure. Group seven gathered materials 37, 69, 78, and 40, the latter standing out for its resistance to the pathogen in the field.
For materials under no pathogen pressure, eight groups were identified (Fig. 1B). Group 1 comprised eight genotypes (1, 2, 55, 70, 100, 7, 43, and 44). Group two was made up of genotype 68, in which the variables FEd (22.20 mm), FPd (21.00 mm), V (μ=6.6 6 ml), FWC (μ=7.11 g) and FW (μ=6.87 g) presented higher values, representing characteristics of interest for selection of parents, in relation to increasing in fruit size (Tab. 5). In group three, genotypes 3, 77, 35 and 69 characterized by high concentrations of total soluble solids TSS (15.42 °Brix) and high maturity index MI (8.99) were located. Genotypes 6, 45, 78, and 46 formed group 4, in which the percentage of citric acid TTA (2.08) was the most important variable. Group 5 (40 and 76) was characterized by a low number of NSF seeds (159.86), low equatorial diameter and polar fruit FEd (17.63 mm), FPd (18.31 mm) and high firmness F (2.10 kg/in2). Therefore, they can be considered as promising materials to improve tolerance to physical postharvest damage.
TABLE 5 Mean of variables for each group conformed by cluster analyses, for genotypes with no pressure of the pathogen.
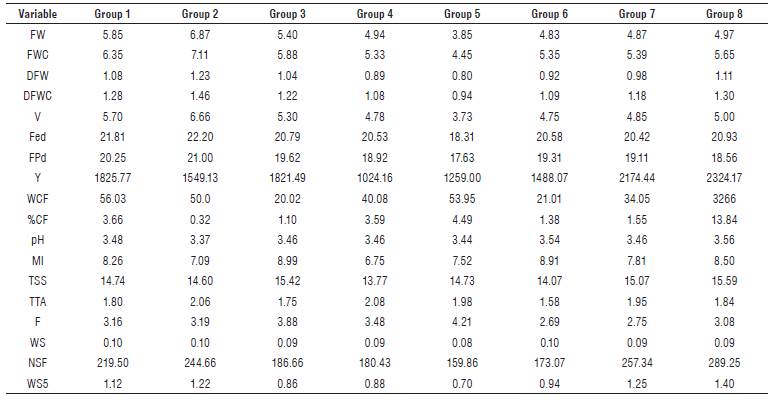
Materials 33, 71 and 41, included in group 6, showed low contents of citric acid (1.58). Group 7 assembled genotypes 34, 74, 75, 37, 38, 39, 73, and 72, harboring materials with high Y on average (2174.44 g/plant), in which genotype 73 had the highest average production (2843.12 g/plant). Genotype 74 showed the highest concentration of TSS solutes (16.14 °Brix). In group 8, there were high Y materials (2324.17 g/plant) and the highest average value for TSS (15.59), highlighting genotype 36. Thus, groups 2 and 8 represent a genetic source of interest to improve fruit quality and production (Tab. 5).
Antioxidant capacity in fruits
The antioxidant capacity analysis was carried out on genotypes 74 (from accession 09U274) and 76 (from accession 09U279), which presented differential resistance responses to Foph (Tab. 1).
The concentration of total phenols did not show statistically significant differences between the evaluated materials 09U279 (resistant) and 09U274 (susceptible) (P<0.05), and it was not affected by the high pathogen pressure (P<0.05) (Tab. 6). On the other hand, the DPPH activity was higher in the susceptible material (309.0 μmoltrolox/100 g BS), compared with the resistant one (213.4 μmoltrolox/100 g BS) (P<0.001), while the high pressure of the fungus did not affect the DPPH antioxidant activity (P<0.05) (Tab. 6). For the oxygen radical absorbance capacity (ORAC), there were differences (P<0.05) between the materials with resistant and susceptible responses, with a greater antioxidant activity in the susceptible material (75.3 μmol trolox/g BS) compared with the resistant one (64.4 μmoltrolox/g BS) (Tab. 6).
TABLE 6. Combined analysis of variance under high and no pathogen pressure for genotypes 76 (09U279) and 74 (09U274) with differential resistance response.
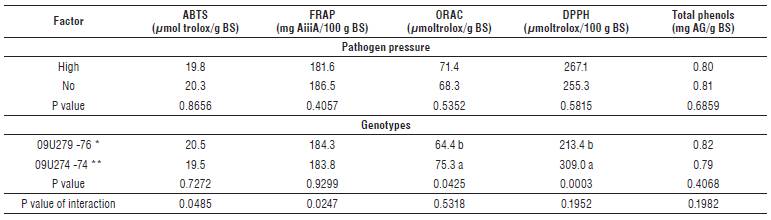
*Genotypes with resistance * and susceptible response **. Averages with different letters indicate significant differences between means (P<0.05).
The antioxidant activity measured by ABTS and FRAP showed significant differences in the interaction between the resistance response (susceptible and resistant) and the pathogen pressure (high and no pathogen (P<0.05, Tab. 6). Therefore, the material with higher resistance response (09U279) showed an increase for these two activities when it was challenged to a high pressure of the pathogen, while for the susceptible material the pathogen pressure was related to the decrease of the antioxidant capacity (Fig. 2).
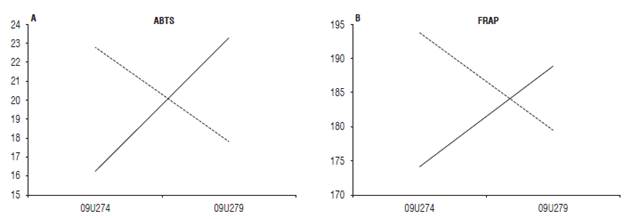
FIGURE 2 Interaction response of resistance x inoculum pressure on A) the antioxidant capacity of Trolox equivalents (ABTS) and B) the antioxidant power of iron reduction (FRAP) according to Pearson's correlation. Material with resistant response (09U279 -76). Susceptible material (09U274-74). Solid line: High pathogen pressure. Dashed line: No pathogen pressure.
The results of antioxidant activity are higher compared to those reported by Lopez et al. (2013), with values in FRAP of 99.70 mg AA/100 g of sample and DPPH of 53.97 μmol trolox/100 g BS. Likewise, they are superior when compared with studies by Botero (2008) with results for FRAP of 54.98 mg AA/100 g sample, total phenols with 39.15 mg AG/100 g of sample and DPPH of 192.51 μmoltrolox/100 g BS for the Colombia ecotype (La Union, Antioquia). This is possibly due to differences in the genotypes and environments studied.
The antioxidant activity could be related to the differential response of the cape gooseberry genotypes against the high inoculum pressure of the Foph pathogen, possibly associated with the expression of an important group of enzymes related to antioxidant activities, signaling, and plant defense. It has been reported that enzymes such as lipoxygenases (LOX), catalases, superoxide dismutases, peroxidases, glutathione reductases, polyphenol oxidases, phenylalanine ammonia lyases, chitinases, and β-1,3-glucanases are induced in response to attack (Peteira and León, 2011). Antioxidant activity measure by ORAC and DPPH in susceptible genotypes could be a defense mechanism of the plant.
Conclusions
The study allowed the identification of materials of interest for the genetic improvement of the cape gooseberry crop focused on fruit quality and yield, in relation to the resistance response to the most limiting pathogen of its production in Colombia, Foph. Privileging the resistance response to Foph and the statistical analyzes of this study, genotypes 1, 36, 68, 73, 74, and 77 are recommended as promising materials for subsequent genetic improvement schemes. In this context, material 36 has been selected for evaluation in different environments for the generation of direct cultivars or as a future parent.
The study, in turn, allowed identifying differential expression in the antioxidant activity of resistant and susceptible genotypes, possibly associated with a mechanism of defense of cape gooseberry against the Foph attack. Future studies will require identifying the defense genes associated with these mechanisms to open possibilities for new approaches in the protection of cape gooseberry against the pathogen.