Introduction
The adequate manipulation of plant arrangements is a very important management strategy for optimizing maize grain yield because it affects the leaf area index, the leaf insertion angle, and the crop efficiency for intercepting solar radiation at different canopy layers (Argenta et al., 2001; Sangoi et al, 2019).
The right choice of row spacing and plant density are two crucial decisions because they will determine the best use of abiotic factors, such as water, solar radiation, and soil nutrients, so that maize can express its physiological potential to produce grains (Penariol et al., 2003).
At the same plant density, a reduction in row spacing allows a greater distribution of plants per area. Therefore, the use of narrow rows may enhance the interception of solar radiation, improve water and nutrient uptake, and increase maize's ability to suppress weed growth. Furthermore, it can reduce water losses by evaporation and favor the use of the same row spacing for maize and soybean speeds up sowing. Narrow rows also permit better seedling distribution along the sowing row due to the low speed of the seeder (Balbinot Jr. et al., 2011; Boiago et al., 2017).
Another strategy to change maize row arrangement is by using a cropping system with twin rows (TR). The planting concept with TR involves the sowing of two neighboring rows with reduced space (16-18 cm) apart from the next two neighboring rows at a greater distance (50-60 cm). The TR system also aims to improve plant distribution per area to decrease intraspecific competition, increase solar radiation interception, and provide more space for leaf and root development (Balem, 2013).
Several factors increased the grower's interest in reducing maize row spacing from the traditional 0.7-0.9 m to 0.4-0.5 m. Among these factors are the development of hybrids more tolerant to crowding, the offer of a great number of selective herbicides to control weeds after plant emergence, and the high agility of the industry to design agricultural equipment adapted for its use in narrow row maize crops (Sangoi & Silva, 2016).
The use of narrow rows does not always enhance maize yield. It also increases the production costs due to the need for adjusting the machine to harvest the crop. Furthermore, it hampers post emergence management practices and increases mechanical damages to the plants during these operations (Jeschke, 2014).
The benefits of reducing row spacing or using twin rows depend on several factors such as the kind of hybrid, the sowing date, plant density, and grain yield expectation. The advantages are more pronounced when the growers use hybrids with erect leaves, sown at the end of the winter with plant densities higher than 70,000 plants ha-1 and a yield perspective greater than 10,000 kg ha-1 (Strieder et al., 2008; Sangoi et al., 2010).
This work was carried out based on the hypothesis that the reduction of row distance and the use of twin rows are effective strategies for manipulating plant arrangements that enhance maize yield. The experiment had the objective of evaluating the effects of narrow and twin rows on the index leaf nitrogen content, the crop leaf area index, and the maize grain yield at different plant densities.
Materials and methods
This study was conducted in the municipality of Lages, state of Santa Catarina, in the highlands of southern Brazil, during the 2016-2017 and 2017-2018 growing seasons. The experimental site is located at 27°50'35" S, 50°02'45" W, and 849 m a.s.l. The climate of the region is Cfb according to the Koppen classification system (Kottek et. al., 2006) with mild summers. The average temperature of the hottest month is below 22°C. The winters are cold, and there is adequate rainfall throughout the year. The soil at the experimental site was a Rhodic Kandiudox (United States Department of Agriculture, 2010).
A soil analysis performed in August, 2016 showed the following values: 470 g kg-1 of clay, water pH 5.2, 13.5 mg dm-3 of P, 232 mg dm-3 of K, 42 g kg-1 of organic matter, 6.1 cmolc dm-3 of Ca, 2.6 cmolc dm-3 of Mg, and 0.1 cmolc dm-3 of Al. The experimental area was in rotation with soybean (Glycine max L.) during the summer with the presence of black oat (Avena strigosa Schreb) preceding maize during the winter.
A randomized block design was used with treatments arranged in split plots. Two plant densities were tested in the main plots (7 and 9 plants m-2), and five row space distances in the split plots (0.4 m, 0.6 m, 0.8 m, 1.0 m and TR). The distance between the rows inside each TR was 0.18 m and the distance between pairs of TR was 0.6 m. The split plots had five replicates and consisted of four rows, with a length of 7 m. All data were collected from the second and third row, excluding 0.5 m at the end of each row.
The experimental area was fertilized with a mixture of nitrogen, phosphorus and potassium on the day of sowing, based on the results of soil analysis and in accordance with the Sociedade Brasileira de Ciência do Solo - Comissão de Química e Fertilidade do Solo (2004) recommendations to achieve a grain yield of 18,000 kg ha-1. The sources used to supply N, P, and K were urea (45% N), triple superphosphate (46% P2O5), and potassium chloride (60% K2O), respectively. The rates applied at sowing were equivalent to 30 kg ha-1 N, 295 kg ha-1 P2O5, and 170 kg ha-1 K2O. Fertilizers were distributed on the soil surface close to the plant rows. Nitrogen was side-dressed at a rate of 250 kg ha-1, and split into three different applications, when the crop was at the V4, V8 and V12 growth stages, according to the scale proposed by Ritchie et al. (1993).
The experiment was hand-sown in a no-tillage system over a dead coverage of black oat on October 20, 2016 and October 21, 2017. The single-cross hybrid P30R50YH was used in both growing seasons. This is a very productive early hybrid recommended for the highlands of Southern Brazil. Three seeds per hill were used. The distance between neighboring hills per line varied according to the plant density and row spacing. Strings previously marked with the proper distance between hills were used to assure the right seed distribution on each treatment. According to Richie et al. (1993), the experiment was thinned when plants were at the V2 stage to adjust plant density to the desired value. Seeds were treated with thiamethoxam (140 g) + fipronil (12 g) and fludioxonil (25 g) + metalaxyl (10 g) per 100 kg of seeds to prevent damages caused by insects and diseases during the crop emergence and early growth stages.
Weeds were controlled with two herbicide applications. The first, immediately after sowing and prior to plant emergence, with a combination of atrazine (1,250 g ha-1) and metolachlor (1,250 g ha-1). The second, after corn emergence when the plants were at V4, with tembotrione (100 g ha-1). Two applications of the fungicides azoxystrobin (60 g ha-1) + cyproconazole, (25 g ha-1) were performed when maize was at V10 and V18 growth stages to prevent leaf diseases.
Soil moisture was monitored with tensiometers. The experiment was irrigated when soil water tension was below -0.4 MPa, to maintain soil moisture close to field capacity. In the first growing season, pluvial precipitation during the crop cycle was 680 mm, and three irrigations were applied. In the second growing season, total rainfall reached 710 mm and the experiment was irrigated twice. Nearly 30 mm of water was provided by a sprinkler system at each irrigation.
Five plants were tagged on each split plot when the crop reached the R1 growth stage (silking), according to Ritchie et al. (1993). These plants were used to determine the nitrogen content of the index leaf (leaf attached to the stem node of the ear), according to procedures described by Vargas et al. (2012).
The same five plants were used to estimate leaf area (LA) and leaf area index (LAI) at the growth stages of R1 (silking) and 30 d after silking, when kernels were at R3 (milk stage). Both measurements followed the procedures used by Leolato et al. (2017). LA was estimated measuring the length (L) and largest width (W) of each photosynthetic active leaf (leaf with >50% green area). Leaf area per plant was calculated adding all the leaf measures and using the following equation:
where 0.75 is a correction coefficient used because leaves do not have a rectangular area. LAI was determined dividing the leaf area per plant by the soil area occupied by each plant, according to the combination of plant density and row spacing.
The number of ears per plant was counted at harvest. The experiment was manually harvested on 04/20/2017 and 04/20/2018, when all leaves were senescent and kernel moisture ranged from 18% to 22%. The ears were shelled, and their grains were oven-dried using a drying oven with circulation/air exchange (MA035/1152, Marconi, Piracicaba, SP, Brazil) at 65°C until the ears were at a constant weight. The number of kernels per ear, 1,000 grain weight and grain yield ha-1 were calculated and expressed at 13% grain moisture.
The data were statistically analyzed by analysis of variance using the SAS statistical software (SAS, 2016). The F test was performed in two ways: individually per growing season and collectively with data from both years. When F values for the main effects and interactions were significant at the 5% significance level (P<0.05), the means were compared by the Tukey's test (P<0.05).
Results and discussion
Significant statistical differences (P<0.05) were observed between the two growing seasons for the number of kernels per area, number of grains per ear, weight of 1,000 grains, and grain yield ha-1. Data from these variables are presented separately for each experimental year. The data related to other variables are described collectively, considering the means of both growing seasons.
The nitrogen content of the index leaf ranged from 3.46% to 4.22%, depending on the plant arrangement (Tab. 1). The mean value for this variable in the experiment was 3.93%. The nitrogen content of the index leaf was affected by the plant density but not by row distance. The density of 7 plants m-2 showed a higher nitrogen percentage at the index leaf than the density of 9 plants m-2. The lower N values observed at the higher density indicate that the competition for nitrogen was more intense in the crowded stand of maize. The same behavior was reported by Fontoura and Bayer (2009), who suggested an increase of 10 kg ha-1 in the nitrogen rate for each 5,000 plants added to plant populations above 65,000 plants ha-1. Plants with adequate nitrogen supply will show a leaf nitrogen content ranging from 3.7% to 4.2% during maize flowering (Cantarella & Marcelino, 2008). Therefore, the results of the experiment show that the amount of nitrogen available in the soil was sufficient to fulfill the needs of the maize regardless of the plant arrangement.
TABLE 1 Nitrogen content of the index leaf of maize plants at silking in five row spaces and two plant densities. Mean values of two growing seasons (2016/2017 and 2017/2018).

Means in the column followed by different uppercase letters are significantly different, and means in the row followed by the same letters are not significantly different according to the Tukey test (P<0.05). The distance between rows inside each twin row (TR) was 0.18 m and the distance between pairs of TR was 0.6 m. Coefficient of variance = 7.63% and 3.21% for plant density (main plot) and row space (split plot), respectively.
Leaf area index at silking and 30 d after silking and the senescent leaf area at maize flowering were significantly affected by plant density but not by row distance (Tab. 2). All row distances showed higher values at 9 plants m-2 than at 7 plants m-2. In all treatments, LAI values measured at silking and 30 d after (kernels at milk stage) were greater than 5.0, considered by Andrade et al. (2002) and Robles et al. (2012) as the critical LAI required for the crop to intercept 95% of the incident solar radiation. The high LAI values, associated with the low senescent leaf area at flowering (less than 600 cm2) indicate that maize plants had a favorable photosynthetic surface area for reaching high grain yield in the experiment.
TABLE 2 Leaf area index (LAI) at silking, LAI 30 days after silking and senescent leaf area at silking of maize plants at five row distances and two plant densities. Mean values of two growing seasons (2016/17 and 2017/18).
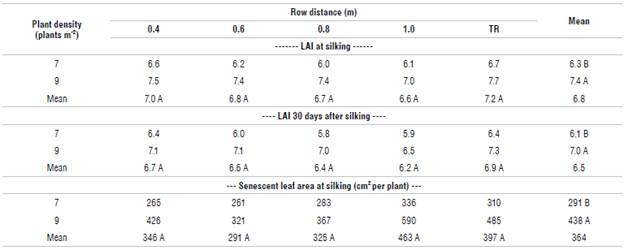
Means in the column followed by different uppercase letters are significantly different, and means in the row followed by the same uppercase letters are not significantly different according to the Tukey test (P<0.05). The distance between rows inside each twin row (TR) was 0.18 m and the distance between pairs of TR was 0.6 m. Coefficient of variance = 8.23%, 9.01% and 6.20% for plant densities (main plot) and 5.34%, 5.89% and 4.80% for row spaces (split plots) of the variables LAI at silking, LAI 30 days after silking, and senescent leaf area at silking, respectively.
Even the plant arrangement that combined high plant density (9 plants m-2) and wide row space (1.0 m) did not speed up leaf senescence. The capacity to keep leaves photosynthetically active for a longer time during grain filling is an important feature in modern maize hybrids. It allows them a superior adaptation to environments with high intraspecific competition (Hammer et al., 2009; Sangoi et al, 2013).
The average grain yields were 15,440 and 16,570 kg ha-1 for the growing seasons of 2016-2017 and 2017-2018, respectively. During the first year grain yield ranged from 14,610 to 16,440 kg ha-1, showing an amplitude of 1,830 kg ha-1 among the different plant arrangements (Tab. 3).
TABLE 3 Grain yield (kg ha-1) of maize plants at five row distances and two plant densities. 2016-2017.
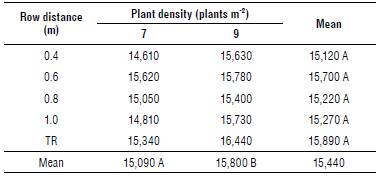
Means in the row followed by different uppercase letters are significantly different, and means in the column followed by the same uppercase letters are not significantly different according to the Tukey test (P<0.05). The distance between rows inside each twin row (TR) was 0.18 m, and the distance between pairs of TR was 0.6 m. Coefficient of variance = 6.40% and 4.86% for plant density (main plot) and row space (split plot), respectively.
In the second year, grain yield varied from 15,650 to 17,570 kg ha-1, having a range of 1,920 kg ha-1 among the different arrangements (Tab. 4). At both growing seasons, the best treatment (twin rows with a plant density of 9 plants m-2) showed a 12% yield increase when compared to the worst treatment (row distance 0.4 m with a plant density of 7 plants m-2). This low percentage difference indicates that there was a small effect of plant arrangement on maize yield. The environment interferes with the grain yield response of maize on the variations of plant density and row space. They can be pronounced according to the soil fertility, climate conditions, and management practices used during the crop growth and development (Schmitt, 2014).
TABLE 4 Grain yield (kg ha-1) of maize plants at five row distances and two plant densities. 2017-2018.
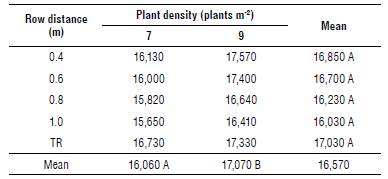
Means in the row followed by different uppercase letters are significantly different, and means in the column followed by the same uppercase letters are not significantly different according to the Tukey test (P<0.05). The distance between rows inside each twin row (TR) was 0.18 m and the distance between pairs of TR was 0.6 m. Coefficient of variance = 5.90% and 5.12% for plant density (main plot) and row space (split plot), respectively.
At both growing seasons, grain yield was affected by the main effect of plant density. The increase of plant population from 7 to 9 plants m-2 produced a yield increment of 4.7% and 6.3% in 2016-2017 and 2017-2018, respectively, on the mean of five row distances (Tabs. 3-4). The greater yield observed at the higher density was probably due to the favorable soil and climate conditions. The experiment was irrigated whenever necessary to keep soil moisture close to field capacity, and the soil fertility was high. The good management practices used during the crop growth and the high yield potential of hybrid P30R50YH also favored the response to crowding. Whenever the environmental conditions are suitable to high kernel yield, maize requires more plants per area to maximize grain yield (Jeschke, 2014; Sangoi et al., 2019).
Row distance did not affect maize grain yield at both growing seasons, regardless of the plant density. This result shows that the reduction of row distance from 1.0 m to 0.4 m or the use of twin rows did not enhance maize yield. Therefore, the more even plant distribution promoted by narrow rows and TR was not an effective management strategy to increase kernel yield. Serpa et al. (2008), Novacek et al. (2013) and Robles et al. (2012) reported similar results in experiments carried out in Brazil and the United States of America, respectively.
The number of grains produced per area increased when plant density was raised from 7 to 9 plants m-2, at both growing seasons (Tabs. 5-6). This yield component was not affected by row distance. On the other hand, the increase of plant density reduced the number of kernels per ear and the 1,000 grain weight, on the average of five row spaces. The number of ears per plant was close to 1 and did not change significantly with plant density and row distance. The yield component behavior proved the observations of Sangoi and Silva (2016) and Boiago et al. (2017), showing that the number of kernels per ear is the variable that has the largest impact on maize yield.
TABLE 5 Yield components of maize plants at five row distances and two plant densities in 2016-17.
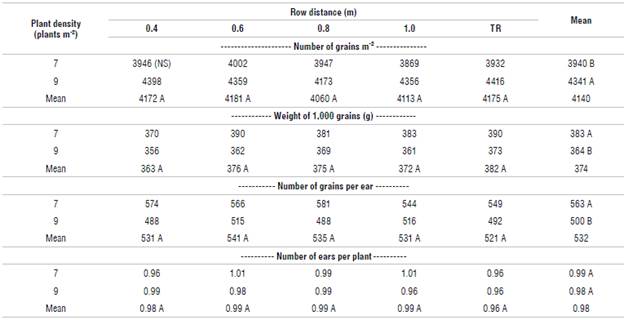
Means in the column followed by different uppercase letters are significantly different, and means in the row followed by the same uppercase letters are not significantly different according to the Tukey test (P<0.05). The distance between rows inside each twin row (TR) was 0.18 m and the distance between pairs of TR was 0.6 m. Coefficient of variance = 10.05%, 4.11%, 9.45% and 7.05% for plant density (main plot) and 8.85%, 3.49%, 8.05% and 6.15% for the number of grains m-2, weight of 1,000 grains, number of grains per ear, and number of ears per plant, respectively.
TABLE 6 Yield components of maize plants at five row distances and two plant densities; Lages, SC, Brazil, 2017-2018.
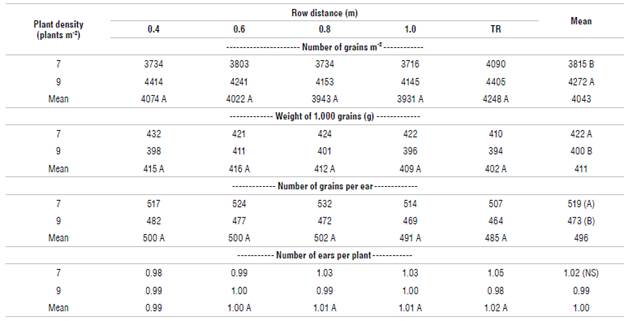
Means in the column followed by different uppercase letters are significantly different, and means in the row followed by the same uppercase letters are not significantly different according to the Tukey test (P<0.05). The distance between rows inside each twin row (TR) was 0.18 m and the distance between pairs of TR was 0.6 m. Coefficient of variance = 10.05%, 4.11%, 9.45% and 7.05% for plant density (main plot) and 8.85%, 3.49%, 8.05% and 6.15% for the number of grains m-2, weight of 1,000 grains, number of grains per ear, and number of ears per plant, respectively.
This study was carried out based on the hypothesis that the reduction of row distance and the use of twin rows are effective strategies to manipulate plant arrangement to enhance maize yield. This hypothesis is based on the premise that both management practices increase the distance between neighboring plants within the sowing row. The improvement in plant distribution may provide a better use of water, solar radiation, and nutrients (Boiago et al, 2017).
The variables measured in the experiment did not confirm the theoretical expectation. The shrinkage of row distances from 1.0 m to 0.4 m or the row arrangement in TR did not significantly change the nitrogen content of the index leaf (Tab. 1) nor the crop LAI at silking and kernel at milk stage (Tab. 2). The absence of significant effects of a more even plant distribution derived from narrow and twin rows on these two physiological parameters was confirmed by the behavior of maize yield that was not affected by the five row distances tested in the trial (Tabs. 3-4).
According to Strieder et al. (2008) and Sangoi et al. (2010), the superior plant distribution derived from using narrow or twin rows has a higher probability of improving the maize grain yield when the crop is grown under conditions that favor high yields. For this reason, the experiments were conducted without water and nutrient restriction. The irrigation and the high soil fertility level provided conditions to attain grain productivity values that were nearly three times greater than the average Brazilian grain yield, which was 5,300 kg ha-1 at the growing season of 2019-2020 (CONAB, n.d.). However, even with yield ceilings above 15,000 kg ha-1, no significant yield advantage of sowing in narrow or twin rows was found, regardless of the plant density (Tabs. 3-4).
The results shown in this research confirmed the observations by Andrade et al. (2002), Lee and Tollenaar (2007), and Jeschke (2014). These authors emphasized that narrow and twin rows have a greater potential for increasing maize grain yield under soil and climatic conditions that prevent the crop from reaching its critical LAI at flowering than when wide row distances are used. Under the environmental conditions of this experiment, the LAI at silking and 30 d after silking was closed to 6.0, even in the wider row spaces of 0.8 and 1.0 m. This shows that solar radiation interception was not compromised, because maize critical LAI ranges from 4.0 to 5.0, according to Andrade et al. (2002) and Robles et al. (2012).
Two other factors that probably contributed to the lack of response to the reduction of row distance were the soil fertility level and the leaf architecture of hybrid P30R50YH. Narrow rows can enhance nitrogen use efficiency (NUE) and increase maize capacity to uptake N from the soil (Barbieri et al., 2008). The higher NUE may increase maize yield in nitrogen deficient environments (Barbieri et al., 2000). The index leaf nitrogen content at silking measured in the trial (Tab. 1) was above the value considered adequate to meet maize requirements by Cantarella and Marcelino (2008). This indicates that there was no restriction in the nitrogen supply for the crop growth and development. The high N rates used in the trial, split over three top-dress fertilizations, contributed to assure an adequate nitrogen supply for the crop during its growth cycle.
The reduction of row distance and the sowing with twin rows have a superior possibility of improving maize yield when hyper-early hybrids, with erect leaves and short plants are grown. This kind of plant architecture allows a greater amount of solar radiation to reach different layers of the canopy. Furthermore, it also prevents the crop from achieving the critical LAI when low plant densities and wide row distances are used (Lauer et al., 2012; Sangoi & Silva, 2016). The hybrid used in the experiment has an early cycle, produces tall plants (plant height ranging from 2.5 to 3.0 m), and has decumbent leaves. These traits allowed P30R50YH to reach the required leaf area index to intercept 95% of the incident solar radiation at all the plant arrangements evaluated in the trial.
Conclusions
The reduction of row distances or the use of twin rows do not change the nitrogen content of the index leaf, the crop leaf area index at silking, and the grain yield of maize hybrid P30R50YH, regardless of the plant density.
The increment of plant density from 7 to 9 plants m-2 is more efficient than the reduction of row distances or the sowing with twin rows to enhance grain yield of maize hybrid P30R50YH.