INTRODUCTION
Mixed-linkage β (1-3) and β (1-4) β-glucans are soluble fibers composed of D-glucose monomers with several applications in health, food and pharmaceutical fields. β-glucans are mainly known for their health benefits such as maintaining normal blood LDL-cholesterol concentrations and reducing postprandial glycemic responses 1.
The use of β-glucans in food is based on their potential to act as a thickening agent 2, emulsion stabilizer 3 and fat substitute 4, qualities related to their physical and molecular proprieties (molar weight, viscosity, molar rate, among others).
In spite of the nutritional and functional benefits of β-glucans, their extraction and purification involve a complex process that requires special attention to improve their yield and keep up their functional properties 5. Wet extraction allows the enrichment of multiple highly concentrated nutrients and improves yields 6. However, solvents and chemicals used on wet extraction can potentially cause loss of functional properties of β-glucans. Besides, wet extraction methods generally yield fiber contaminated with partially solubilized starch 7. This contamination in β-glucans extracts is responsible for viscosity alterations 8. Thus, it is recommended to use enzymes such as amylases and proteases to eliminate the starch and proteins that could be adhered to the fibers 6.
Differences in the physicochemical characteristics of β-glucans are attributed mainly to genetic and environmental factors, as well as to differences in extraction methodologies, purification, depolymerization, and analytical methodologies used to calculate their molecular mass 9. Thereby, molecular mass and viscosity of β-glucans are affected by their physical state in the grain, betaglucanase activity, processing and storage conditions 10.
The importance of chemical characterization of extracted β-glucans before their application in foods has been widely emphasized. In that sense, 10 mention that the food industry has always shown a keen interest in physicochemical and functional properties of nutraceutical compounds, as this will help in the choice of a specific type of compound with particular characteristics. The aim of the present work was to characterize barley β-glucans extracted (EβG) and to compare it with three commercial β-glucans in order to identify the best soluble fiber for future application in food. Subsequently, the extracts (EβG) were purified (PβG) and molecular and structurally assessed to identified what extent these processes affect the characteristics of β-glucans.
MATERIALS AND METHODS
Materials
Commercial dehulled barley was supplied by SL Alimentos Ltd. (Paraná, Brazil). The kit for the determination and purification of β-glucans was obtained from Megazyme International Ltda (Wicklow, Ireland). The β-Glucan test kit content lichenase enzyme (specific, endo-(1-3)(1-4)-β-D-glucan 4-glucanohydrolase) to breakdown the link endo (1-3) (1-4); beta-glucosidase enzyme para breakdown of β-Gluco-oligosaccharides in D-glucose and GOPOD reagent to assay the D-glucose produced. Two commercial brands of β-glucan (CβG: A and B) were supplied by Polycell Technologies (Minnesota, USA) and one sample (CβG C) was supplied by Cargill Company (Minnesota, USA) Commercial β-glucans were selected in order to identify the best soluble fiber for future application in food. MES/TRIS buffer, and 2,5-dihydroxybenzoic acid (DHB) were bought from Sigma-Aldrich, Brasil Ltda (Sao Paulo, Brazil).
Extraction of β-glucans
Barley β-glucan was extracted in according 11 with some modifications (HCL was not used to avoid the β-glucan degradation during extraction). The barley grains were ground to 0.5 mm, mixed with distilled water (1:10), adjusted to pH 8 with CaCO3 (20% w/v) to precipitate the starch, and heated in a water bath (30 min at 50 ± 2°C). The solution was cooled to room temperature and centrifuged (4850 g/30 min at 4°C). The supernatant was recovered and an equal volume of ethanol (99 %) was added for β-glucan precipitation. After 16 hours at 4°C, the solution was centrifuged (3700 g/10 min at 4°C). The β-glucan precipitate was recovered and washed with 99 % ethanol. Excess moisture was removed at room temperature for 2 hours. The extracted β-glucans (EβG) were kept in a desiccator for 24 hours prior to grinding in an IKA mill (Model A11 Basic) and sifted to 0.5 mm.
Composition analysis
Chemical characterization of all samples was performed according to the American Association of Cereal Chemists 12 methods of analysis. The analysis were: moisture (Method 44.01.01); ash (Method 08.01.01); protein by Kjeldahl, using 6.25 as conversion factor (Method 46.10.01) ); lipids by Soxhlet extraction with petroleum ether (Method 30.10.01); total (FT), insoluble (FI) dietary fibers by enzymatic gravimetric procedure using the kit assay supplied by Megazyme Internacional Ltda. (Wicklow, Ireland) (Method 32.07.01); total starch and β-glucan by enzymatic procedure using assay kit the Megazyme International Ltda. (Wicklow, Ireland) (Methods 76.13.01 and 32.23.01, respectively). Reported values are the means of three replicates. The results were expressed in grams per 100 grams (dry basis).
Mineral content
Mineral content was performed according to the AOAC Method 968.08 13 for calcium (Ca), iron (Fe), magnesium (Mg), and zinc (Zn), by flame atomic absorption spectrometry (F-AAS). The atomic absorption spectrometer (Analyst 200) was equipped with deuterium arc, background corrector, and Echelle optical resolution system. Acetylene (99.7% purity) was used as fuel to heat the atomizer system and compressed air was used as support gas. All samples were previously calcined at 550°C. Ash were diluted with HCl 8 mol/L. Hollow cathode lamps for the analyzed elements were from Lumina.
Phosphorus (P) was determined by molecular spectrometry and the AOAC Method 965.17, using a visible UV spectrophotometer with tungsten lamp, set at 420 nm (Evolution 60S, Thermo Scientific, Madison, USA). After calcination and complexation as molybdovanadate phosphoric acid, phosphorus was determined by interpolation in calibration curves prepared with KH2PO4 in the concentration range from 1 to 20 mg/L. Sodium and potassium were determined by flame atomic emission spectroscopy (F-AES) at 589.0 nm with a flame photometer (Analyser Comércio e Indústria Ltda., São Paulo, Brazil), after calcination and ash dilution with nitric acid (HNO3) 4 mol/L. The results of the samples were interpolated in calibration curves that ranged from 1 to 10 mg/L. (AOAC Method 969.23). The results were expressed in mg per 1 kg.
Water binding capacity (WBC)
The WBC of the samples was measured using the method described by 5 with some modifications. A volume of 20 mL of distilled water was added to a pre-weighed centrifuge tube containing 200 mg of β-glucans. The hydrated samples were placed on a shaker at 25°C for 3 hours and centrifuged (4850 g/30 min at 25°C). The supernatant was discarded, and the tubes with the precipitate were weighed again. The WBC of each sample was expressed as the weight of water held by 1.0 g of β-glucan sample and was quantified by Equation 1.
Analyzes were performed in triplicate for each sample
Determination of pH
The samples were pre-diluted in distilled water at 1.5% (w/v), placed at 80°C for an hour, cooled to room temperature for two hours, all with continuous mixing. The pH was determined, according to method 943.02 14 using a previously calibrated pH meter (Q400A-Quimis, Ltda. São Paulo, Brazil).
Rheological assessment
The rheological behavior of aqueous dispersions (1.5% w/v) of β-glucans was assessed following the methodology used by 11 with modifications. It was performed on a rotational rheometer (Brookfield Engineering Laboratories model RVDV-III Ultra, Stoughton, MA, USA) using concentric cylinders: spindle S21 (for EβG) and ULA (for commercial brands samples). Duplicate analysis was carried out for each sample. Rheological responses were analyzed by the Ostwald Waale model or Power law by Equation 2:
Where: (a = Apparent viscosity (mPa.s-1), K= Consistency index (Pa.sn), ( = Deformation rate (s-1), n = flow behavior index (dimensionless).
Color
The color was determined using a Chroma Meter CR-400 colorimeter (Konica Minolta, Osaka, Japan) with CIE-Lab system (L*, a*, b*) using D65 illuminant and 10° viewing angle. Where L* defines the brightness of the product with L* = 100 as white and L* = 0 as black; a* = red (+) or green (−) direction; b* = yellow (+) or blue (−) direction of a product. The saturation index C*, indicates the intensity of a hue, was calculated by Equation 3: The reported values are the means of five replicates.
Where: C*: Saturation index; a*: Red coloration; b*: Yellow coloration
Purification of extracts
EβG sample was diluted with MES/TRIS buffer 0.05 M (pH 8.2) in the ratio 2.5% (w/v) and stirred for 15 min to guarantee complete hydration and dispersion without forming clumps. Thereafter, 50 μL of thermostable α-amylase (3000 U/mL) was added and incubated (35 min/95°C). Samples were cooled (60ºC), then 100 μL of protease (50 mg/mL) were added and incubated (60ºC/30 min). Samples were cooled, pH adjusted to 4.5 (HCl 5%) and 200 μL amyloglucosidase (140 U/mL) was added and incubated at 60°C/30 min. The extract was added to ethanol 99% (4:1, v/v), rested for 15 min, centrifuged (4850 g/4°C/20 min) and the precipitate was washed with ethanol (99%). The purified fraction of β-glucans (PβG) was freeze-dried and saved for further analysis. Protein, starch, β-glucans, WBC and color analysis were performed on the PβG with the same methodologies previously described.
Determination of molar weight
The determination of average molecular weight (Mw), radius of gyration (Rg) and the second virial coefficient (A2) were performed in NaOH solution (0.5 mol/L) following the method described by 15. Static measurements were conducted using a Brookhaven instruments including a precision goniometer (BI-200SM), and a monochromatic laser light source (Laser He-Ne, λ = 632.8 nm), with power of 35 mW (Brookhaven Instruments, Holtsville, NY, USA). Light scattering measurements were carried out in the angular range of 40 - 150° with intervals of 5°, using decalin as an internal reference. The signal was digitized by a discriminator-amplifier and analyzed by a multiple digital correlator. Purified β-glucans samples (PβG) were diluted in several concentrations (0.1 - to 0.8 mg/mL) to ensure complete hydration and dispersion without forming clumps. The solutions were filtered through 0.45 mm cellulose ether filters and placed into glass cells cylindrical vials with 10 mm in diameter. From the intensity of light scattered at different angles (θ), for different concentrations of polymer, the Zimm Plot diagram was constructed using the software supplied with the equipment. All the light scattering measurements were performed at 25°C. The refractive index increment, dn/dc, was determined as 0.146 mL/g for β-glucan in aqueous solution. Five replicates were performed at each dilution. The result was expressed in kudo.
Structural characterization by MALDI-TOF
β-glucan hydrolysates (Hβ-G) were prepared following the methodology used by 16 with modifications. Solutions (0.2 to 0.5% w/w) of PβG in water HPLC grade were prepared by incubation in a boiling water bath with continuous stirring until the sample was fully solubilized. After cooling to room temperature, the pH was adjusted to 6.5 followed by the addition of 1.35 mL of lichenase (150 U). The mixture was incubated at 50°C/1 h, and stirred every 20 min. The hydrolysate was heated in a boiling water bath to inactivate the enzyme and centrifuged at 4580 g for 20 min. The supernatant was collected and subjected to matrix assisted laser desorption ionization time of flight (MALDI-TOF) analysis. The β-glucan hydrolysates (Hβ-G) were mixed with three different matrices (DHB, Super-DHB, and DHB + Isovanilin) in the ratio of 1:3 and dried for 10 min at room temperature. The nitrogen laser pulse was accelerated under 20 kV before entering the time-of-flight mass spectrometer. The spectra were acquired in m/z range from 400 to 20,000 Da with low mass gate set to m/z = 400, 65% laser intensity, 2000 laser shots accumulated (200 to 200, in linear positive mode, reflector. The MALDI-TOF spectra were recorded on a MALDI-TOF Autoflex III Smartbeam Bruker Daltonics and processed and calculated by PolyTools software from Flex Analysis (Bruker Daltonik).
RESULTS
Chemical analysis, pH and WBC
Extracted (EβG) and commercial CβG (A, B, C) β-glucans presented significant differences (p<0.05) for all nutrients analyzed, except for lipids as showed in the Table 1.
Table 1 Composition analysis of β-glucans samples in dry basis.
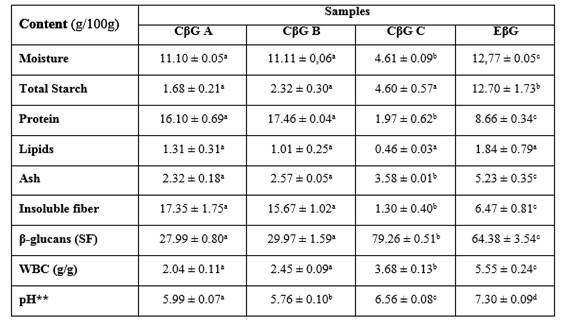
Means ± standard deviation followed by equal letters in the same line does not have significant difference by the Tukey’s test (p > 0.05).
** Dimensionless. WBC = water binding capacity; SF = soluble fiber.
Particularly, CβG A and CβG B presented lower starch, higher protein, higher insoluble fiber (IF), and lower β-glucans (SF) levels. The bigger value of IF in the two commercials samples CβG A and CβG B seemed to indicate a possible dry extraction process of β-G. Dry extraction sequentially separates the pericarp, aleurone and subaleurone layers and embryo by abrasive cleaning to obtain concentrated fractions of βG still attached to high levels of IF 7 and 17. In addition, protein results from these samples (CβG A and CβG B) suggest that purification of the extracts was not performed. Soluble fibers (β-glucans) appeared in a larger proportion in CβG C and EβG (p<0.05).
The WBC was higher in the EβG sample than in the sample CβG C, followed by CβG B and CβG A (p<0.05) (Table 1). As EβG and CβG C have higher β-glucan content, it was expected that the higher WBC due to their ability of β-glucans to bind to water.
Mineral content
The results of mineral content are showed in the Table 2. All samples presented a significant difference. The most abundant mineral in the samples was phosphorus, followed by potassium, calcium and magnesium.
Table 2 Mineral content of β-glucans samples.
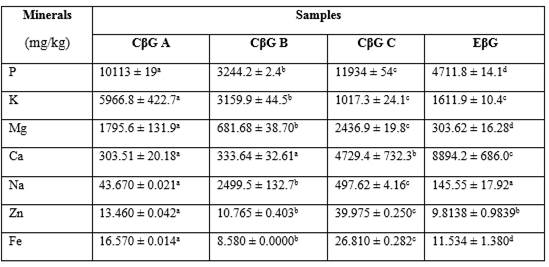
Means ± standard deviation followed by equal letters in the same line does not have significant difference by the Tukey’s test (p>0.05).
On the other hand, sodium concentration was significantly higher in the CβG B samples than in the rest of the samples and the concentration of iron was higher in the CβG C samples (p<0.05).
Rheological assessment
In the commercial samples (CβG-A, B, and C), the shear stress was proportional to the shear rate, being a Newtonian behavior as shown in the Figure 1A. On the other hand, the sample EβG presented a flow behavior similar to a pseudoplastic fluid where the viscosity decreases with the increase of the shear rate and the ratio between shear stress and shear rate is not linear as shown in the Figure 1B.
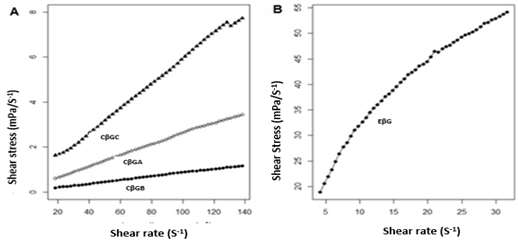
Figure 1 Flow-behavior of β-glucans (1.5%). A: Commercial samples. B: extracted barley β-glucans (EβG).
The rheological behavior of all samples was well explained by the power law model (R2>0.99) as shown in the Table 3.The three commercial samples (CβG-A, B and C) presented low consistency index (k) and flow behavior index (n) approximate to 1, keeping closer to the Newtonian behavior, exhibited proportionality between the shear stress and the shear rate.
Table 3 Power Law parameters (ŋ = k (ɤ) (n-1)) of β-glucan samples.
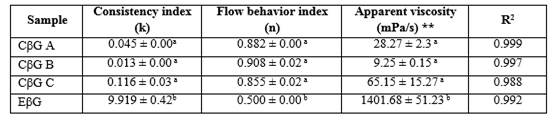
Means ± standard deviation followed by equal letters in the same line does not have significant difference by the Tukey’s test (p>0.05).
** Viscosity available in shear rate = 50 s-1.
On the other hand, EβG had a high consistency index (k) and a mean value of flow behavior index (0.5), indicating pseudoplastic characteristics (Table 3).
Color
In the Table 4 is showed color results. The highest values of luminosity (L*) were presented by the CβG-A followed by the CβG-C, CβG-B, and finally by the EβG, with significant differences.
Table 4 Color results of β-glucan samples.

Means ± standard deviation followed by equal letters in the same line does not have significant difference by the Tukey’s test (p > 0.05).
The EβG presented a darker color (Table 4). All samples presented values of parameter a* lower than 10 suggesting that red tonality is not present in great proportion in the samples analyzed. The highest values of b* were found in EβG and CβG-B, which indicate that those samples were slightly yellowish.
Purified extracts - PβG
Protein, total starch, β-G content, and WBC in purified extracts (PβG)
After purification, total starch decreased to 0.85 ± 0.46% in PβG. Additionally, PβG samples also had lower protein (5.50 ± 0.12) and high βG (69.45 ± 0.81) values. The WBC for PβG was 3.47 ± 0.38 (that is, each gram of PβG can retain up to 3.47 grams of water). The PβG presented color parameters with increased brightness L* (92.60 ± 1.70), decreased saturation index C* (4.81 ± 0.18), and showed a* (0.81 ± 0.08) and b* (4.75 ± 0.19) values which overall resulted in lighter samples with better appearance.
Molar weight of PΒG
Since β-Glucans easily aggregate in aqueous solutions (because of inter-molecular hydrogen bonding), the Zimm Plot was performed in 0.5 mol/L NaOH to remove aggregates. The molar weight of PβG by light scattering was 690 ± 1.6 kDa with a gyration (Rg) radius of 50 ± 10 nm. The second viral coefficient A2 was found to be 2.30 ± 0.37 x 103 cm3 mol/g2.
Structural characteristics
PβG was hydrolyzed with lichenase (Hβ-G) and characterized by MALDI-TOF to identify affinity of Hβ-G in three different matrices of ionization (DHB, Super-DHB, and DHB + Isovanilin) and obtain polysaccharides mass spectral profile. All matrices analyzed presented good MALDI-TOF signal by the clearest fragmentation pattern, however, DHB matrix (20 mg/mL 70% ACN/30% H2O/0.2% TFA) presented a high S/N ratio to fragments with DP>5 as shown in the Figure 2.
In this study, the MALDI-TOF spectrum of hydrolyzed β-glucan (Hβ-G) confirmed the presence of DP3 to DP11 species with DP3 (Mw = 526.976 Da) and DP4 (Mw = 689. 976 Da) being the predominant species.
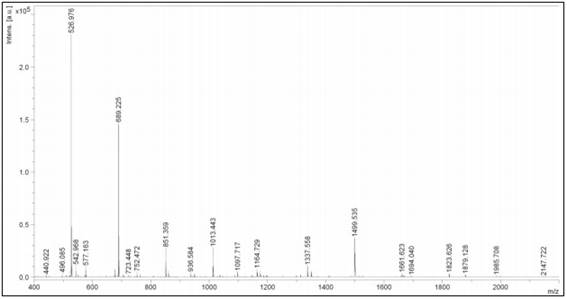
Figure 2 MALDI-TOF mass spectrum of barley β-glucan hydrolysate (Hβ-G) in DHB matrix. Fragments DP3 (Mw: 526,976); Fragments DP4(Mw: 689,225); Fragments DP5 (Mw: 851,359); Fragments DP6(Mw: 1013,443); Fragments DP7(Mw: 1164,729); Fragments DP8 (Mw: 1337,558); Fragments DP9(Mw: 1499,535); Fragments DP10 (Mw: 1661,623); Fragments DP11(Mw: 1823, 626).
DISCUSSION
Chemical analysis, pH and WBC
The EβG sample presented a high starch level, indicative of larger contamination during fiber extraction. This happens in wet extraction, the starch is partially solubilized bonding to the β-glucan molecule 17. Overall, the main contaminants of β-glucans are starch and proteins present in the barley grain 18. β-glucan content is directly affected by extraction and purification processes used, therefore, interaction with other constituents of the barley grain is important in the technological perspectives of this polysaccharide 17. Barley β-glucan extraction under laboratory conditions resulted in similar values of starch 13.8%, protein 4.15% 11. Values of 15% of proteins were found in extracted oat β-glucans 19.
With regard to WBC, 20 presented similar values (3.54 - 4.77) in β-glucans gums. According to 21, the high WBC of the gums resulted in water absorption and intermolecular forces leading to the formation of aggregates or structures in the three-dimensional matrix. These interactions provided functional properties (gelling agents, thickeners, stabilizers, suspending agents) to the gums.
Mineral content
The phosphorus content of commercial samples (CβG-A, B and C) and the extracted (EβG) found in this study are within the ranges reported by 19 and are higher than those reported by 5. The high content of calcium in the EβG samples may be a consequence of the extraction because calcium carbonate was used during starch precipitation. The presence of other compounds such as protein, starch, metals and phosphorus can cause the cleavage and thermal degradation of β-glucans and consequently affect their molar mass and viscosity 19. According to 22, the presence of iron (Fe2+), can promote the formation of OH˙ radicals and consequently generate degradation of the polysaccharide, affecting its viscosity.
Rheological assessment
Similar values of the rheological behavior were reported for extracts of barley β-glucans by 23, indicating values k = 0.06 and n = 0.92. According to 18, pseudoplasticity of β-glucans has been established for high viscosity molecules and usually presents a high coefficient of consistency (k) and low flow behavior index (n). Viscosity values are directly proportional to WBC and pH. That is, when samples have higher pH, they have higher WBC and higher viscosity. Basic pH causes inactivation of the endogenous beta-glucanase preventing degradation of the fiber by the enzyme, keeping the molar mass of β-glucans and consequently their viscosity 24. Additionally, high pH values facilitate the solubility of the polysaccharides, mainly starch. This may facilitate the hydration of the starch and β-glucans present in the EβG increasing its volume and consequently the viscosity of the solution. Nevertheless, the viscosity had no direct relationship with the β-glucan content in the samples, as expected. In the sample CβG-C, the content of fiber soluble (β-glucan) was higher than EβG sample, but the viscosity was lower. The high viscosity found in the EβG sample can be explained by the presence of 12.7% of starch and 5.23% of ash. Additionally, concentration, solubility and molar mass, also affected the viscosity of β-glucan solutions 25. There is a direct relationship between viscosity increase and molar mass, reflected in the pseudo-elasticity characteristics of this compound and 9).
Color
High L* values (greater than 83) were related in β-glucans extracted by enzymatic methods, with low levels of starch and protein 5. However, 20 reported L* values between 60.52 and 64.78 for β-glucans treated with high pressures at different dilutions. High degree of luminosity has an industrially appeal to add ingredients in other food products colors 5. Differences in the drying processes in Cβ-G and EβG can also influence the final hue. Thus, while CβG is usually freeze-dried or spray-dried 17, the EβG samples were air-dried and left in a desiccator, allowing the aggregation of the particles, oxidation reactions and probable enzymatic reactions leading to darkening.
Protein, total starch, β-G content, and WBC in purified extracts (PβG)
The results of PβG samples confirmed that WBC of β-glucans is maintained even after purification. WBC is an important physicochemical property of dietary fiber associated with viscosity and their physiological effects 26. The WBC is attributed to the ability of the fiber to form networks that allow the retention of water through inter and intra molecular interactions.
Molar weight of PβG
The high value of Mw in the PβG can be explained as consequence of the extraction and purification procedures that sought to maintain the integrity of the molecular structure. In this research, no was used protein precipitation procedure with HCl during the extraction. According to 24, pH values close to 4 can cause acid hydrolysis, decreasing the molar mass. The European Food Safety Authority (EFSA) indicated that the molar mass of barley β-glucans can range from 50 to 2000 kDa 1. In this sense, the molar mass of nine cultivars of Irish spring barley β-glucans were found in a range between 250.0 to 375.0 kDa using size exclusion chromatography (HPLC-SEC) 24 and the other hand, molar mass were found in a range between 150 to 581 kDa using dynamic light scattering 15. Differences in the molar mass of β-glucans have been attributed to extraction methodologies, purification, depolymerization events, and the analytical methodologies used to calculate molar mass 9.
Structural characteristics of PβG
DP3 and DP4 units are the main building blocks of barley β-glucans 16. According to 9, the major hydrolysates for cereal β-glucans are cellobiosyl-D-glucose (DP3) and cellotriosyl-D-glucose (DP4). However, others oligosaccharides are also released in smaller amounts from the polymer regions containing more than three consecutive linked glucose residues.
Our results indicated that starch and protein contaminations associated with extraction, as well as the drying process, affect the final quality of the EβG. High viscosity in EβG could difficult to use this fiber in concentrations greater than 1.5%. The use of enzymes is a highly effective method for purifying β-glucan samples improving their appearance without modifying their functional and structural characteristics
CONCLUSIONS
The extraction conditions used in this study yielded extracts (EβG) with high levels of fiber, highly viscous, showed high WBC and dark color when compared to commercial samples, which makes it difficult to use this extract in concentrations of 1.5% to be included in foods.
The enzymatic purification process applied in the extract was effective removing contaminating starch and proteins, and improving the color while maintaining the WBC. Besides, the purified extract (PβG) presented high molar mass and kept the main molecular structures of the fiber (DP3 and DP4 species). Preserve these characteristics in the soluble fiber (β-glucan) is important to its functional effect in the intestine when consumed. Therefore, the enzymatic purification of the EβG can be recommended before its application in others foods.
On the other hand, two commercial β-glucans brands presented low fiber content (CβG-A and CβG-B). Consequently, the use of high amounts of these brands will be necessary to achieve values suggested for the consumption of soluble cereal fiber.
Finally, CBG-C presented higher purity, higher CRA, higher viscosity when compared to the other commercial samples. These samples at concentrations of 1.5% presented rheological properties that allow to be recommended for application in foods such as beverages, raw meat by injection or even incorporation in some foods, such as meat emulsions.