INTRODUCTION
The interest in health-enhancing foods has increased considerably, and the functional foods have managed to stand out and reach a favorable position in new consumption habits. Fruits and vegetables provide many of the nutrients needed for an adequate diet, and they are also a source of bioactive compounds that support various bodily functions intending to maintain the body in the best conditions of health. A significant source of bioactive compounds is berries. These fruits that have been particularly interesting to researchers due to the great diversity of phytochemicals associated with the prevention and/or reduction of several chronic diseases, for example obesity, diabetes, cancer, cardiovascular and neurodegenerative diseases, caused mainly by alterations in the oxidative balance (oxidative stress) produced by an excess of free radicals (1-2).
The Andean berry, Vaccinium meridionale, is a shrub that grows wild in the Andean rainforests. It produces fruits of 5 to 10 mm in diameter, with purple-red to almost black color. It has a slightly astringent and acidic taste. In addition to fresh consumption, the fruits also can be found as processed products such as jams, jellies, syrups, juices, yogurts, bakery products, pastries, and ice cream (3). V. meridionale is a fruit rich in polyphenols, an extensive family of bioactive compounds found mostly in plants as secondary metabolites and which are important for having a high antioxidant, anti-inflammatory and free radical scavenging activity (4). In this context, the presence of flavonoids, tannins, and anthocyanins as the main compounds stands out; also, other compounds such as tocopherols, glucosinolates, organosulphur compounds, sterols, saponins, and stilbenes to a lesser extent (5).
Despite the interesting nutraceutical properties, Andean berry fruits have a low shelf life due to a high post-harvest respiratory rate and microbiological decay caused mainly by fungi (6). It is, therefore, necessary to transform the fruit into higher valueadded products such as wine and vinegar that can be used as raw materials in the food industry and allow these bioactive ingredients present in the fruit to be maintained or increased by making them available to consumers.
According to FDA, the vinegar is “the product made by the alcoholic and subsequent acetic fermentation of the juice of fruits”. The permitted content for vinegar, expressed as acetic acid, must not be less than 40 g/L, except for wine vinegar, which must be at least 60 g/L (7). In vinegar brewing, ethanol is converted to acetic acid by aerobic acetic acid bacteria through reactions catalyzed by two membrane-dependent enzymes, pyrroloquinoline quinone (PQQ), a dependent alcohol dehydrogenase (ADH), and aldehyde dehydrogenase (ALDH). In the first stage ADH, oxidizes ethanol to acetaldehyde, then, ALDH converts acetaldehyde into acetic acid. The process is directly coupled with the electron transport chain, which makes it energetically favorable for the bacteria (8). Gourmet or aged vinegar are very popular in European and Asian food, used as dressings or as sauces ingredients for retail consumer products and even haute cuisine products. Depending on the fruit of origin, vinegar can maintain or yet increase nutraceutical compounds due to the physicochemical and microbiological changes that occur during the double fermentation and aging (9).
Along with the production of acetic acid, other compounds are formed, including organic acids, esters, ketones, and aldehydes that contribute to the organoleptic properties of vinegar according to the characteristics of the fruit (10). It has been reported that regular consumption of vinegar contributes to the diet with polyphenols, micronutrients, and other bioactive compounds, which are responsible for the antimicrobial, antidiabetic, antioxidant, anti-obesity, and antihypertensive effects (11-13). Recent studies with patients, Ali et al. (2019) reported that a daily consumption of 30 mL of date vinegar in mildly hypercholesterolemic adults, improved substantially the blood lipid profile (total cholesterol, high and low density lipoproteins and triglycerides) and inflammatory biomarkers (C-reactive protein, nitric oxide, tumor necrosis factor alpha and fibrinogen) (14).
This research aimed to produce an alcoholic beverage and vinegar from the fermentation of fresh fruits of Andean berry (V. meridionale). Three different processes were used to obtain the fruit juice, macerated fruit machine (MAC), preheating of the fruit at 80 °C (CAL), and a combination of both of them (MIX). The fermentative parameters of performance, the monitoring of the antioxidant capability, and the content of antioxidant compounds were evaluated. Finally, the antiproliferative capacity of vinegar in SW480 colon cancer cells was determined.
MATERIALS AND METHODS
Raw material
Andean berries (Vaccinium meridionale) were harvested in Santa Elena, in the eastern region of Antioquia, at an average height of 2,600 m.a.s.l. It is a humid tropical climate with an average temperature of 14.5 °C and relative humidity of 89%. This material has a voucher number ILS 14050070. Fruits were transported to the laboratory in plastic bags and then washed correctly.
Pretreatment of must and preparation of Juice and alcoholic beverage
The methods of musts treatment described by Solieri and Giudici (2008) and Ferreira et al. (2009) (7, 15) were modified and adapted. Musts were prepared by adding 1 kg of fruit and 1 L of water. The beverages were obtained using three different methods, macerated fruit machine (MAC), preheating of the fruit at 80 °C for 15 min (CAL), and a combination of both of them (MIX). The yeast was prepared according to the procedure described by Alfenore et al., 2004 (16). Saccharomyces cerevisiae (Pasteur Red Star), 0.4 g of yeast per 100 mL of beverage were used. It was activated in the water at 37 °C for 10 min. Subsequently, alcoholic fermentation was performed for 20 days at room temperature (17). The Juice was prepared in the same way avoiding the fermentation process.
Vinegar preparation
For the production of Andean berry (V. meridionale) vinegar, the alcoholic beverage previously described was taken as the source of the substrate and diluted to 35 g/L (18).
Source of microorganisms and inoculation process
The fermentative process was developed using common microorganisms. Starter culture called “Vinegar Mother for Red Wines” commercially distributed by Beer & Wine Hobby (Vinegar cultures VIN000R, 155 New Boston St. MA). The inoculum preparation protocol consisted of adding in equal proportions 1:1 of the starting substrates and the Andean berry alcoholic beverage, diluted to 40 g/L of ethanol. This fermentation process was carried out until reaching 3 g/L of acidity, expressed as acetic acid. The product obtained was used as inoculum for the acetic fermentation at a concentration of 30 g/L, for a final volume of 150 mL (19).
Acetic fermentation
The experiments were performed in 1.0 L Erlenmeyer flask with 150 mL of culture medium, yeast extract 1.6 g/L at 30 °C and agitation rate at 150 rpm (20).
Titratable acidity (TA)
The acid value was determined by acid-base titration according to the AOAC method 942.15, 1996 (21), 10.0 mL of sample were placed in 250 mL Erlenmeyer flasks, plus an equal volume of distilled water. A standardized NaOH solution (0.1 M) was dropped from the burette until the pH value was stable at 8.2 ± 0.5. The pH was monitored with a pH meter Metrohm model 744. The samples were analyzed in triplicate, and the results were expressed as the percentage of acetic acid, g of acetic acid per 100 mL of solution.
Content of acetic acid
The quantification of acetic acid at the end of the fermentation process was made according to the method described by Bouzas et al. (1991) (22), with some modifications. An LC-20AD liquid chromatograph (Shimadzu, Tokyo, Japan), equipped with a SIL-20A auto injector/HT, a communication module CBM-20A and RID10A refractive index detector, chromatographic column was an Aminex® HPX 87H 300 x 7.8 mm, (Bio-Rad California, USA) was used. The calibration curve was made with acetic acid standard (10-100 g/L) (Sigma-Aldrich). The mobile phase was sulfuric acid (0.005 M) in a flow of 1.0 mL/min, injection volume sample of 20 µL, and column temperature oven of 65 °C. The samples were analyzed in triplicate, and the results were expressed as grams of acetic acid per liter of sample.
Antioxidants assays
Free radical scavenging activity (DPPH)
The Brand-Williams method was used with some modifications (23). Into a test tube, 10 µL of the diluted sample, and 990 µL of a DPPH standardized solution were added. As a reference, the same amount of DPPH and 10 µL of sample solvent (water) were used. The antioxidant capacity was measured employing the decrease in absorbance after 30 minutes. The reaction wavelength was 517 nm. The percent inhibition of the radical was calculated, and the results were expressed as Trolox equivalents: μmol Trolox per liter of the sample (μmol TE/L), by the construction of a standard curve, using Trolox as the antioxidant. The analysis was were performed in triplicate.
Ferric Reducing Antioxidant Power (FRAP)
The reducing power was done according to the method proposed by Benzie and Strain (1996) (24). A solution of TPTZ (2,4,6-Tris(2-pyridyl)-s-triazine, Sigma-Aldrich St. Louis, Missouri, USA) with FeCl3 , sample, and buffer pH 3.6 are used. After 30 min, the absorbance was read at a wavelength of 593 nm. The reference curve was constructed using ascorbic acid (Sigma-Aldrich). The results were expressed as ascorbic acid equivalents: mg ascorbic acid per liter of the sample (mg AAE/L). The FRAP analysis was performed in triplicate.
Oxygen radical Absorbance capacity (ORAC)
The test used Trolox as standard and temperaturecontrolled conditions at 37 °C. The assay was determined by the methodology description of Zapata et al. (2013) (25), by diluting the Trolox in 75 mmol/L in phosphate buffer at pH 7.4. The readings were done at an excitation of 493 nm, and emission of 515 nm. For the development of the technique, 3 mL of the solution was prepared: 21 µL of a 10 mmol/L fluorescein solution, 2,899 µL of phosphate buffer, 50 mL of AAPH 600 mmol/L and 30 µL of sample or 500 mmol/L Trolox. The antioxidant protector effect was calculated using the differences of areas under the decay curve of the fluorescence between control and the sample and the comparison against the Trolox curve. The results were expressed as µmol of Trolox equivalents per liter of the sample (µmol TE/L), according to Equation 1:
Where AUCsample is the area under the curve of the samples, AUCcontrol is the area under the curve of the control (without sample), AUCTrolox is the area under the curve of Trolox and f is the ratio of sample and Trolox concentrations.
Content of antioxidant metabolites
Total polyphenols The determination of polyphenols was done by the colorimetric method of Folin-Ciocalteu (26). Briefly, a volume of 50 µL of each sample was mixed with 125 µL of Folin-Ciocalteu reagent, then 425 µL of sodium carbonate solution (71 g/L) was added, and the final volume was adjusted to 1 mL with distilled water. The reaction was kept in the dark for 60 min, and afterward, the spectrophotometric absorbance was determined at 760 nm. A standard curve was constructed using gallic acid as the standard (Sigma-Aldrich). Analyses were performed in triplicate, and the results were expressed as mg of gallic acid equivalents per liter of the sample, mg GAE/L.
Total anthocyanins
Total anthocyanins were determined by the differential pH method (27). Two aliquots of 100 μL of samples were mixed separately with 900 μL of buffers at pH 1.0 (a potassium chloride 25 mM solution adjust pH to 1.0 with HCl 37%) and pH 4.5 (a sodium acetate 0.4 M solution adjust pH to 4.5 with HCl 37%). Then, the absorbance of test portions was measured on a Multiskan Spectrum spectrophotometer (Thermo-Scientific) at 530 nm and 700 nm. The anthocyanin content was expressed as cyanidin-3-glucoside equivalents per liter of the sample (mg C3G/L), according to the Equation (2):
where A=, MW=449.2 g/mol (molecular weight of cyanidin-3-glucoside), ε=2,6900 L/(mol×cm) (molar extinction coefficient of cyanidin-3- glucoside), L=1.0 cm (pathlength in cm) and DF=is the dilution factor of the samples. Analyses were performed in triplicate.
Phenolic acid content
Chlorogenic, caffeic, ferulic, and p-coumaric acids were determined by HPLC, (LC-20AD liquid chromatography, Shimadzu). The samples were filtered through 0.45 µm pore size nylon membranes and diluted with type I water. Chromatographic conditions were mobile phase acetonitrile/acidified water (phosphoric acid pH 2.5), (4:6 v/v). Phenolic components were eluted under the following conditions: flow 1.0 mL/min, 25 °C and isocratic conditions, Colum LiChrospher 100 RP-18, Merck. The UV-visible spectrum ranged from 200 to 600 nm for all peaks, the identification and quantification of the components were made with calibration curves for each of the phenolic acids (28), and the results were expressed as mg of each phenolic acid per liter of the sample, mg/L. Analyses were performed in triplicate.
Antiproliferative activity
Cell culture
Cytotoxic activity was evaluated to verify the functionality of vinegar polyphenolic compounds versus the ability to affect the viability of human colon adenocarcinoma in SW480 cell line due to their effects on the viability and growth of primary tumor cells of colon cancer. SW480 cells obtained from the American Type Culture Collection (ATCC, Manassas Virginia, USA). Some 3,000 viable cells were maintained as described by Maldonado et al. (2009) (29). Briefly, a mix of Dulbecco’s Modified Eagle Medium (DMEM) supplemented with 100 g/L inactivated horse serum, 100 U/mL penicillin, 100 μg/mL streptomycin, and 10 g/L non-essential amino acids were used. Incubations were made at 37 °C in a humid atmosphere with 5% CO2. For all experiments, 24 h after seeding, the medium concentration was decreased to 30 g/L serum, with 10 μg/mL insulin, 5 μg/mL of transferrin, and 5 ng/mL of selenium. The control cells were treated with the extraction vehicle: 1.0 g/L DMSO in the culture medium.
Sulforhodamine B Assay (SRB)
The effect of extracts on growth cells were studied by SRB assay, a colorimetric assay based on staining of total cellular protein from cells with SRB dye. 3,000 viable cells from each cell line were exposed to samples for 24 h after being seeded and incubated for different times. DMEM medium (30 g/L) was replaced every 48 hours with different concentrations (20-200 μg/mL) of vinegar. Cell culture was disrupted with 50 g/L trichloroacetic acid for 1 h at 4 °C, and cell proteins were stained with 4 g/L SRB at 24, 48, and 72 hours. The absorbance of SRB is proportional to the number of adherent and living cells that were determined by optical density at 490 nm using an ELISA plate reader (GloMax®-Multi Promega, Madison Wisconsin, USA) (30). The percent inhibition was calculated by Equation 3:
Where Odt: Optical density of treated samples and Odc: control optical density. The concentration of the extract causing 50% inhibition of cell growth (IC50) was calculated using GraphPad Prism 5.0 (GraphPad Software Inc., San Diego, CA).
Statistical analysis
DPPH, FRAP, ORAC, total polyphenols, total anthocyanins, hydroxycinnamic acid contents, acidity, and growth of SW480 cells were carried out in four replicates. All analysis was performed with the statistical package “R” (Version 15, R-Foundation, New Zealand). The differences between the variables were analyzed to determine the significance through the unidirectional variance analysis (ANOVA) and Fisher’s minimum signif icant difference procedure (LSD) to discriminate between the means.
RESULTS AND DISCUSSION
The fruits of Andean berries (Vaccinium meridionale) grown in the Andean region of Colombia were used as a source of natural antioxidants; from these fruits, juice, alcoholic beverage, and vinegar were prepared. The extraction of the bioactive compounds was carried out using three general procedures: macerated fruit machine (MAC), preheating the fruit at 80 °C (CAL), and a combination of both (MIX). The same numbers of alcoholic beverages were fermented, and subsequently, the various kinds of vinegar were obtained, the fermentation parameters were characterized and presented below.
Acetic Fermentation
The acetic fermentation was monitored by titration to evaluate the acidity of the medium. Figure 1 shows the three fermentation processes (MAC, MIX, and CAL) at concentrations of ethanol of 35 g/L, which produced acidity after two days in the lag phase. MIX was the extraction method that presented the highest process of acetification, indicating that the maceration and subsequent heating of the Andean berry fruits, extracted the highest amount of fermentable sugars. De Ory et al. (2004) (31) showed that the lag phase of the fermentation increases proportionally with the concentration of ethanol. It can remain constant up to 30 g/L, but if it reaches 40 g/L, it presents inhibition, affecting the growth of bacteria. Other components can act as activators or inhibitors, depending on the degree of toxicity they have, such as acetic acid (32). Gullo et al. (2008) (33) showed that ethanol tolerance is a species and strain-dependent trait that is conditioned by temperature, pH, and oxygen. In addition, the strains tolerance range is associated with media conditions and substrates.
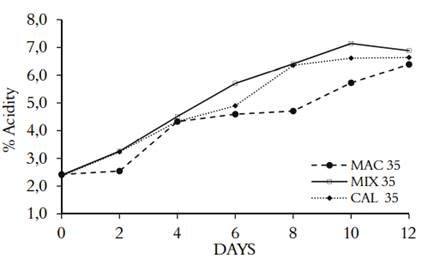
Figure 1 Acidity kinetics in acetic fermentation of three different processing of V. meridionale fruits
Table 1 shows the productivity and yield of acetic acid from the three fermentation processes from each kind of alcoholic beverages. ANOVA analysis indicates that the correlation of the factors (MAC, MIX, and CAL) was not statistically significant (p<0,05). Suggesting that the different methods of fruit processing do not affect the biotransformation capacity of alcohol to vinegar; in previous works, we found the same behavior for the alcoholic fermentation of berries of V. meridionale (17).
Polyphenolic metabolites content and antioxidant capacity of juice, alcoholic beverage and vinegar made from V. meridionale berries.
The results of the antioxidant activity and measurement of secondary metabolites for the samples of juice, alcoholic beverage, and vinegar are shown in Table 2 and 3, respectively. The majority of assays show that the antioxidant capacity decreases in the vinegar compared to the juice. At the same time, the alcoholic beverage remarkably increases the antioxidant power even above the juice. The DPPH assay displayed a significant decrease in all three fruit processing, and corroborated by the ORAC assay, which had a reduction for the three processes (Table 2). FRAP evaluates the reducing power of the sample, evidencing a significant decrease in the MAC process. In contrast, MIX presented an increase, and CAL remained. In agreement with our results, Dávalos et al. (2005), found that antioxidant capacity measured by ORAC of red and white grapes vinegar were lower than the antioxidant capacity of the respective starting juices.
Their values varied from 25,000 µmol TE/L of juice sample to 11,500 µmol TE/L of vinegar sample. The authors explained the decrease in antioxidant capacity as a loss in phenolic and non-phenolic antioxidants in the two-step fermentation process (34). In this research, the antioxidant activity was compared within the three fermentation processes (juice, alcoholic beverages, and vinegar). They presented a fall during the process of acetification to produce the vinegar from alcoholic beverages. In DPPH, the decrease was 45%, 37%, and 45% for MAC, MIX, and CAL respectively, corroborated by the ORAC technique, which showed a more significant drop 78%, 66%, and 71% for the same procedures. In contrast, FRAP technique, the antioxidant activity, only falls in the extraction method MAC with 35%. The antioxidant power in vegetal samples is determined by the expression of some secondary metabolites with antioxidant capability, according to the type of extraction and the intrinsic capacity of the antioxidant molecules, the antioxidant power can be increased (35).
Table 2 Antioxidant activity of juice, alcoholic beverage and vinegar of three different processing of V. meridionale fruits
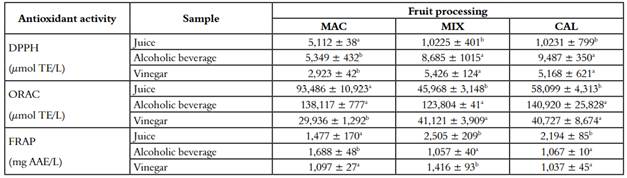
*Different letters in superscripts in the same column indicate that the mean difference is significant at the level of 0.05 per LSD (mean ± SD, n = 4)
Table 3 Antioxidant compounds of juice, alcoholic beverage and vinegar of three different processing of V. meridionale fruits
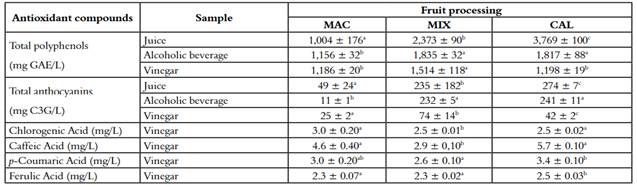
*Different letters in superscripts in the same column indicate that the mean difference is significant at the level of 0.05 per LSD (mean ± SD, n = 4).
In a brewing process, several physicochemical changes may or may not favor the appearance of antioxidant substances derived from more abundant polymeric compounds such as higher molecular weight polyphenols (36). The content of antioxidant metabolites is presented in table 3, where the total polyphenols decreased significantly in MIX and CAL. Still, they remained equal in MAC. Total polyphenolic components also showed a decrease in the MIX and CAL procedure with 17% and 34%, respectively, as well as the anthocyanins that diminished for the last two extraction methods in 68% and 82%, respectively. Likewise, anthocyanins content presented the same trend. However, this decrease, the number of bioactive components present in in the different kinds of vinegar, are a good source of antioxidants in the diet. For example, beverage type juice rich in antioxidants from acai palm fruit (Euterpe oleracea Mart.) as the predominant ingredient, with a total polyphenols and ORAC content similar to Andean berry (1480 mg GAE/L and 22,200 µmol TE/L, respectively), showed protection from oxidative damage in erythrocytes as well as anti-inflammatory properties in vivo due to the decrease of pro-inflammatory markers such as leukotrienes and interleukins (37).
Figure 2 shows the chromatograms and the retention times for the main phenolic acids present in the vinegar samples. It must be noted that the chromatographic profile between the three extraction methods is similar, which indicates that there are no significant changes in the composition of the samples. Table 2 presents the content of hydroxycinnamic acids obtained by HPLC. It is evident that the CAL extraction method achieved the best results, which can be explained since, at temperatures above 75 ºC, enzymes such as polyphenoloxidase (PPO) of fruits are inhibited (38). Several authors have studied the production of fruit vinegar, reporting that the process of the acetification reduces the antioxidant activity, the concentration of polyphenols, and mainly the concentration of anthocyanins (19, 39-40). However, it is essential to stand out that polyphenolic composition represents a useful quality marker, according to its composition in low molecular weight molecules (41).
The fermentations were carried out in a discontinuous way, and the process included a previous fermentation intended to constitute the vinegar mother. Then, it was added to the diluted alcoholic beverage. Those changes in conditions, mainly attributable to ox ygen availability, could explain affectation of the anthocyanins concentration. They can be oxidized, precipitated, or polymerized with other phenolic components that affect the content of polyphenols. This fact is evidenced in the obtained results (19, 39).
Polyphenolic compounds such as phenolic acids and anthocyanins express a high antioxidant activity. A decrease of these bioactive compounds explains the drop in the antioxidant activity. The induction of the acetic fermentation process requires the dilution of the alcohol beverage, to promote the growth of acetic acid bacteria; therefore, all the components present in the sample are diluted. This procedure might explain the decrease in the antioxidant activity. In the production of industrial vinegar, this practice is performed. The results obtained from the vinegar are comparable to Cerezo et al. (2010), which reported a red wine vinegar from Cabernet Sauvignon with antioxidant activity values for ORAC, DPPH and FRAP of 4,630 µmol TE/L, 10,200 µmol TE/L and 1,693 mg AAE/L, respectively (42).
Effect on growth and cytotoxicity of Andean berry vinegar in human colon adenocarcinoma cells SW480.
The CAL extraction method presented the highest results in the antioxidant activity. Therefore, it was decided to measure the antiproliferative activity. Andean berry vinegar was evaluated with the SRB assay after the procedure with the cells for 72 hours with vinegar at different concentrations. The effect of vinegar on the growth of SW480 cells is shown in figure 3A, where the optical density (OD) at 490 nm corresponds to the proteins of cells treated or not treated with Andean berry vinegar. The OD of SW480 cell proteins indicates a 93.9% growth that reached 77.4% after treatment for 72 hours with vinegar between 20 μg/mL and 200 μg/mL compared to untreated cells. The Andean berry vinegar showed inhibition of cell growth at all concentrations evaluated in a dose-dependent manner. Figure 3B shows that vinegar suppression of proliferation of SW480 cells from 6.2% (20 μg/mL) to 22.5% (200 μg/mL). The IC50 value extrapolated for vinegar in SW480 cells was 536 μg/mL, in previous works with an Andean berry fermented beverage, we found IC50 values of 139.1 µg/mL (95). However they are lower than those found by us, and they represent a greater antitumor capacity on human colon cancer cells. The decrease in antioxidant metabolites during acetic fermentation, especially anthocyanins (Table 3), could explain the differences found between previous reports and the findings in this research. Considering that these types of substances are mainly responsible for the anti-carcinogenic activity due to their proapoptotic effects. The proposed mechanism is very complex and includes the formation of apoptotic bodies, loss of mitochondrial membrane potential, activation of Bax: Bcl-2 genes, activation of caspase 3, and proteolytic activation of PARP (poly (adenosine 5′-diphosphate-ribose) polymerase (43). The antioxidant effect in vitro of anthocyanins has been demonstrated in different cultures of colon cells, exhibiting antitoxic and anticarcinogenic effects, increasing the radical oxygen absorption capacity of the cells, reducing the formation of oxidative adducts in the DNA, decreasing of the lipid peroxidation, inhibiting mutagenesis by environmental toxins, and carcinogens (44, 45). Although not only anthocyanins function, hydroxycinnamic acids have also been associated with antiproliferative effects (46). Other authors have reported antiproliferative activity from dealcoholized wine, within 24 hours of growth and with concentrations of 100 and 200 μg/ mL gallic acid equivalents, reaching inhibitions of 61% and 78% respectively (47). On the other hand, it has been reported that rice vinegar have succeeded in inhibiting the proliferation of cancer cells by up to 62% (48). In comparison to these values, it can be concluded that Andean berry vinegar has a high antioxidant activity, but its antiproliferative activity is low.
CONCLUSIONS
Fruits of Andean berry (V. meridionale) were transformed in alcoholic beverage and vinegar by brewing using three extraction processes. The acetic fermentation parameters such as yield and productivity were independent of the extraction methodology used, indicating that processing the fruit does not affect the biotransformation capacity of alcohol to vinegar.
The berries and derived products such as wine and vinegar showed a high content of antioxidant bioactive substances, despite this, the fermentation process affects the concentration of polyphenols, especially anthocyanins since in the CAL procedure they decreased notoriously, although this fact can be contrasted with a higher extraction of other polyphenols by the same method. The antioxidant capacity decreases in the vinegar compared to the juice. At the same time, the alcoholic beverage remarkably increases the antioxidant power even above the juice for the majority of extraction assays; the CAL procedure presented the highest results in the antioxidant activity. Andean berry vinegar had an antiproliferative effect on colon cancer cells that was dose-dependent across the range of concentrations, evaluated after 72 hours of contacting the cells with the vinegar. The estimated IC50 value was 536 μg/mL, which, according to the National Cancer Institute of the USA criteria, is considered low antiproliferative activity. Finally, this research suggests that the consumption of fermented berry beverages may contribute to cancer chemoprevention in secondary cancer prevention due to the content of bioactive compounds and their antioxidant power.