Services on Demand
Journal
Article
Indicators
-
Cited by SciELO
-
Access statistics
Related links
-
Cited by Google
-
Similars in SciELO
-
Similars in Google
Share
CT&F - Ciencia, Tecnología y Futuro
Print version ISSN 0122-5383On-line version ISSN 2382-4581
C.T.F Cienc. Tecnol. Futuro vol.3 no.3 Bucaramanga Jan./Dec. 2007
THERMAL MATURITY HISTORY AND IMPLICATIONS FOR HYDROCARBON EXPLORATION IN THE CATATUMBO BASIN, COLOMBIA
Historia de la madurez térmica e implicaciones para la exploración de hidrocarburos en la cuenca del Catatumbo, Colombia
Antonio Rangel1 and Roberto Hernández2
1Ecopetrol S.A. - Instituto Colombiano del Petróleo, A.A. 4185, Bucaramanga, Santander, Colombia e-mail: rangel.av@gmail.com
2Ecopetrol S.A. -Aex, Bogotá, Colombia. e mail: Antonio.Rangel@ecopetrol.com.co
A thermal model integrated with an oil and gas geochemical study has been constructed for the Catatumbo Basin, Colombia to provide petroleum system data for hydrocarbon exploration.
The calibration of the thermal model with maturity data took into account a changing heat flow scheme which included a thermal increase towards the end of the Jurassic and another one in the Early Eocene, associated with rifting events.
Locally, active/generating source rocks are within the synclines axes. The hydrocarbon expulsion time for Cretaceous source rocks (Capacho and La Luna formations) started in the Upper Paleocene-Eocene, while for the Los Cuervos Formation the generation and expulsion started at 10 my. The petroleum expelled during the Paleocene-Miocene, were likely accumulated in structures formed since the end of the Cretaceous, while the younger structures that resulted from the Andean orogen were charged by remigration from the older structures and additionally with the yougest lately generated hydrocarbons. The accumulations of hydrocarbons are mainly the result of generation and migration locally within the basin.
The Catatumbo basin contains thermogenic wet gases with different degrees of thermal maturity which varies from around 1,0 to 2,5 equivalent Ro. The highest degree of thermal evolution according to maturity indicators and thermal modeling is in the southern area, which is prospective for wet gas. The central and northern area appears more prospective for oil with minor amounts of gas.
Keywords: organic geochemistry, source rock, crude oil, maturity indicators, thermal modelling.
Un modelamiento integrado con un estudio geoquímico de gas y aceite ha sido realizado en la cuenca del Catatumbo, Colombia con el fin de proveer información para la exploración de hidrocarburos.
El ajuste del modelo térmico con los datos de madurez fue posible a partir de un esquema de flujo de calor cambiante, que incluyó un incremento térmico hacia finales del Jurásico y otro en el Eoceno Temprano, asociados a eventos distensivos.
Regionalmente, en los ejes de los sinclinales se identificaron pods de roca fuente activa en el presente. Los tiempos de expulsión de hidrocarburos para las rocas fuente Cretáceas (Formación Capacho y la Luna), inician en el Paleoceno-Eoceno Superior mientras que para la Formación Los Cuervos la generación y expulsión inicia hace 10 ma. Las acumulaciones de hidrocarburos se infiere que son el resultado principalmente de generación y migración dentro de la cuenca. La fracción de petróleo expulsado durante el Paleoceno-Mioceno posiblemente fue acumulada en estructuras que crecieron desde finales del Cretácico, mientras que las estructuras más jovenes resultantes de la orogenia andina se infiere que se han cargado con los productos de la remigración desde las estructuras más antiguas y adicionalmente con las últimas fracciones de hidrocarburos generadas.
Los gases de la cuenca Catatumbo son del tipo termogénico húmedos con diferente grado de madurez termal que varía desde alrededor de 1,0 hasta 2,5 de Ro equivalente. De acuerdo con el grado de evolución termal, la geoquímica y el modelamiento térmico, se infiere que la región sur es prospectiva para gas húmedo y condensado, mientras que el sector central y norte es prospectivo para aceite y cantidades menores de gas asociado.
Palabras clave: estudio geoquímico, roca fuente, petróleo crudo, datos de madurez, modelo termal.
INTRODUCTION
The Catatumbo Subbasin is a prolific Colombian hydrocarbon province, located in the southwestern edge of the Maracaibo Basin (Figure 1). The area of the subbasin is 7350 km2 with sedimentary column nearly 15 000 feet thick (Figure 2). The estimated recovery from eleven oil and gas fields is around of 550 million barrels of oil. The oil comes from reservoirs at different stratigraphic intervals, i.e. Cretaceous fractured carbonates, and Cretaceous and Tertiary clastics, in structural or stratigraphic traps.
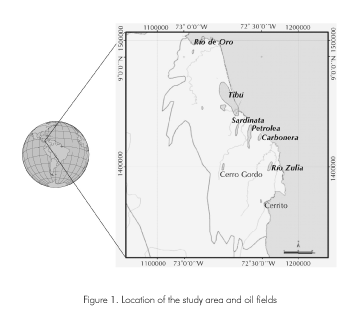
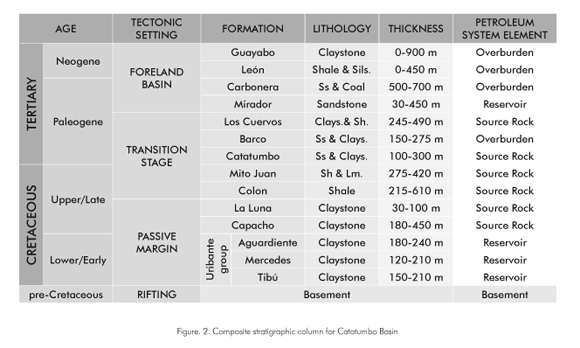
Many geological, geochemical and thermal modeling studies have documented the petroleum potential of the Venezuelan portion of the Maracaibo Basin. (Talukdar, Gallango, & Chin-A.Lien, 1985; Talukdar, Gallango, Vallejos, & Ruggiero, 1987; Sweeney, Talukdar, Burnhan, & Vallejos, 1990; Talukdar, & Marcano, 1994). However, very little has been published about the Catatumbo Subbasin. Yurewickz, Advocated, Lo and Hernández (1986) integrated hydrocarbon Geochemistry and basin modeling, as well as some Ecopetrol Internal reports such as Mondragón (2001), Ecopetrol ICP (2001), and Mora (2000).
Regarding the thermal history of the Maracaibo Basin, Sweeney et al. (1990), reconstructed the thermal history of the basin using a constant heat flow during the past, similar to their calculated present-day heat flow values (close to 50 mW/m2).
For the Catatumbo Subbasin, Yurewickz et al. (1986) believed that the source rock maturation depended partially on a contribution from a late Jurassic thermal anomaly associated with back-arc rifting. Their burial history predicts a crustal thickening of 30% to 40%.
According to Marcano and Cassani (1994), the present-day maturity of the southeastern portion of the Maracaibo Basin should be modeled using a heat flow between 43 and 55 mW/m2. However, Llerena and Marcano (1997) argue that the Jurassic rifting event partially affected the level of maturity of the Cretaceous source rocks of the Catatumbo Subbasin. Later, Rodríguez and Navarro (1997) recognized an insignificant thermal effect of the Jurassic rift event on the maturity evolution of the La Luna Formation.
In summary, many authors discuss the option to model the thermal maturity of the Maracaibo Basin, using a constant heat flow during the past similar to the present heat flow. However, the anonomalously high source rock maturity observed in some areas is an indicator that the paleo heat flow was higher at some time in the past.
The purpose of this study is to add to the thermal history discussion of the Catatumbo Subbasin a new thermal modeling approach that takies into account an increased heat flow during the Lower Eocene (54-59 my). This thermal event was associated with an extensional stage related to the Lara Nappes tectonism observed by Bueno and Pinto (1997), Fimlay and Gou (1997) Rodríguez and Navarro (1997). In addition, the basin modeling is corroborated with a maturity assessment of produced oil and gas. This leads to usefull observations for hydrocarbon exploration of the Catatumbo Basin.
METHODS AND SAMPLING
Oil and gas production samples from representative wells in the Catatumbo Subbasin were collected (Figure 1). More than 20 saturated fractions of oil were subjected to Gas Chromatography (GC) and Gas Chromatopraphy Coupled to Mass Spectrometry (GC-MS). Total alkane fractions or branched/cyclic sub-fractions were analyzed for the selected ions on an H.P. 5890 GC-MS system. The GC column was a 30 m HP-5 temperature programmed from 60ºC-320°C (303,15ºK- 593,15ºK) at 4°C/minute and helium carrier gas at 1,5 ml/min. Recorded on the 5890 MSD were m/z 177, 191, 217, 218, 259 for saturated fractions.
Seven gas samples were analyzed. Gas composition was measured using a gas chromatograph equipped with a flame ionization detector for the ethane to i-butane fraction and a thermal conductivity detector for methane and non-hydrocarbons. Carbon isotope analyses were performed at CENPES-Petrobras with IRMS equipment (isotope ratio monitoring system mass-spectrometer). Methane, ethane, propane, and butane were separated by GC, combusted in an oven and the CO2 directed to a MS for isotope measurement. Carbon isotope compositions were reported as d13C (%) relative to the V-PDB standard with an estimated precision of ± 0,2%.
This study also discusses source rock data collected from previous studies, most of them for internal reports in Ecopetrol S.A. The thermal history was constrained by modeling five representative wells in the basin and one pseudowell in one of the deepest areas along the Sardinata syncline. The thermal modeling was done using the Basin Mod-1D of Platte River Associates.
GEOLOGICAL SETTING
The tectonic-sedimentary and thermal history of this subbasin is tied to that of the Maracaibo Basin, and in general to the north of South America, and it can be summarized in four main events: A rift-type extension stage, a passive margin stage, a foreland basin transitional stage and a foreland basin stage.
The tectonism that separated the Pangea developed a mosaic of extensional basins throughout the Atlantic margin (Pindell, George, Kennan, Cristancho, & Higgs, 1998; Villamil, & Pindell, 1998). Towards the early Cretaceous, a period of thermal subsidence started and developed a shallow marine platform which extended from the East of Venezuela to Ecuador and Peru (Macellari, 1988). Towards the end of the Cretaceous, the collision of the Pacific Plate, against the western margin of the South American Plate, transformed the passive margin into an active orogenic belt which started the development of a foreland depositional system (Parnaud, Gou, Pascal, Capella, Truskowski, & Passalacqua, 1995; Pindell et al., 1998). The foreland basin developed at the beginning of the Tertiary with the oblique collision and transcurrent deformation of the Caribbean Plate and the Panama Arc throughout the passive margin of the north of South America, generating several deformation events in the area of the Maracaibo-Catatumbo arc; among them is the progressive movement towards the east and south of the Lara Nappes in the northern part of Maracaibo Basin at the end of the Paleocene. In addition the Andean mountains, Perijá Range, Massif of Santander and the Mérida Andes were uplifted and, Maracaibo-Catatumbo Basin separated from of the Barinas-Apure and the Middle Magdalena Valley Basin (Parnaud et al., 1995).
From a sedimentary point of view, the mega sequence of the Jurassic-Triassic comprises the Girón, La Quinta and equivalent formations; they are the economic basement (Figure 2). Towards the end of the Lower Cretaceous, during the Aptian, the shales, mudstones, limestone and sandstones of the Tibú, Mercedes, and Aguardiente formations were deposited, (Figure 2), which form the main regional reservoir. Subsequenly, the Capacho and La Luna source rock formations were deposited in an anoxic marine environment. These are the main oil source rocks of the subbasin. The transitional tectonic phase is dominated by progradation of the Colón-Mito Juan sequences on top of the La Luna Formation, from the southeast toward the northeast of the subbasin. The foreland stage contains deltaic and shallow marine sediments of the Barco, Los Cuervos, Mirador and Carbonera formations. Some intervals of Los Cuervos Formation may constitute minor oil source rocks.
DISCUSSION
Source rock formation
The La Luna Formation (Conancian to Late Campanian) and Capacho Formation (Cenomanian-Turonian) are the main source rocks with average values of 3,2% and 2,1% of TOC, respectively and a maturity at the oil window in the north and beginning of the gas window towards the south (Ecopetrol-ICP, 2001). The Hydrogen Index (HI), is now generally low in both formations, below 200 mg HC/g TOC. This can be attributed to the high maturity reached. The kerogen of the La Luna Formation is Type II, while in the Capacho Formation it is Type II / III. The petrographic analyses by Ecopetrol-ICP (1993) indicate high percentages of solid bitumen in both formations, varying between 5% and 60%, with an average of 28%, evidence of hydrocarbon generation and migration.
The Capacho Formation is 180-450 m in thick and shows at the bottom facies characteristic of shallow platform environments, which deepened and became more dysoxic towards the top. Regionally, there is no significant variation in facies.
The La Luna Formation has an average thickness of 60 m and consists of limestone intercaled with distal marine platform shales. This formation varies from a calcareous to a muddy limestone facies with a clastic content of up to 30%. The least calcareous facies are located in the western part of the basin (Ecopetrol-ICP, 2001).
The Paleocene sequence (Catatumbo and Los Cuervos formations) consists of shales and coal and are rich in organic matter, Type II/III with a TOC content that vary from 1% to 69% in the coal units. The thickness of the Cuervos Formation (Late Paleocene –Early Eocene) range from 200 m to the east (Tarra, Los Manueles and Petrolea fields) to 500 m in the Río de Oro Field (Figure 1). Towards the south of the Catatumbo Subbasin, in the Cornejo-Santiago section, it reaches a thickness of 315 m. For the Paleocene sequence the highest risk is the thermal maturity, which is low in most of the basin. Overall, in both the Cretaceous and Tertiary formations, the maturity generally increases towards the south.
The maturity of the basin from the vitrinite reflectance (Figure 3) shows a higher thermal gradient towards the south in the Cerrito 1 well, compared with the wells of the central area. The vitrinite reflectance profiles reveal tectonic effects by faults as well as a period of strong erosion of the younger horizons.
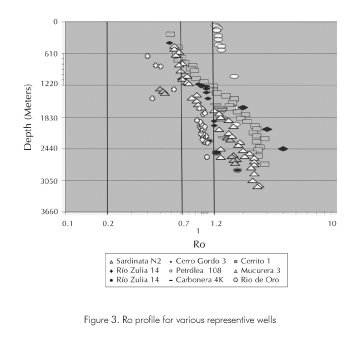
Heat flow and thermal maturity modeling
For the thermal history modeling we adopted a variable heat flow throughout the tectonic history of the basin. Despite the high heat flows associated with the rift stage (200 my - 110 my), this event did not affect the critical times of generation and migration which started at about 57 my in the southern basin and ended 5 million years ago in the center and northern basin. The post-rift stage involves an exponential decrease of the heat flow. According to McKenzie (1978), Allen and Allen (1990) for the active rift phase the heat flow is around 63-110 mW/m2 depending on the stretching factor and in the thermal subsidence phase decays to about 40 mW/m2.
During the passive margin stage (Lower Cretaceous to Upper Paleocene) an exponential decrease of the heat flow is interpreted (Figure 4 and Table 1). This is produced by a tendency towards thermal equilirium. In the starting stage of foreland development in the Lower Eocene (54 - 49 my) an increase in flow rate is used coinciding with the rifting event discussed by other workers (Bueno and Pinto, 1997; Fimlay and Gou, 1997; and Rodríguez and Navarro, 1997). This is a consequence of the extensional phase related to the tectonism of Las Napas de Lara throughout the pre-existing Jurassic and Cretaceous faults. This heat flow spike has a great impact on the maturity of the source rocks and the generation and migration processes, and is fundamental to calibrating the thermal history.
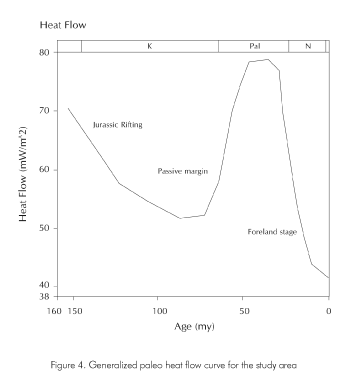
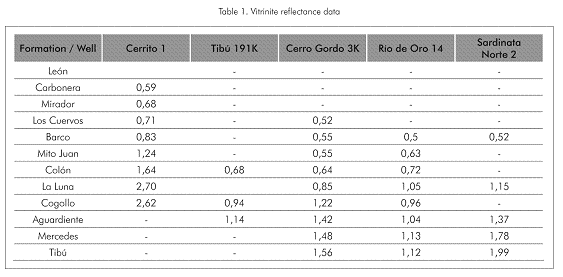
In this exercise the following wells were modeled: Río de Oro-14, Sardinata –3, Cerro Gordo-3, Mucurera-3 and Cerrito-1 representing the north, center and south of the basin.
The modeling temperature was calculated from a transient heat flow model. The method for calculating temperature takes into account thermal conductivity and the calorific capacity of each lithology data. The used heat flow models are the result of comparison without constraining by the maturity data available in Ecopetrol-ICP, 2001, specifically the Ro and Tmax data.
The present thermal regime was evaluated from a study of the temperature data of the bottom of the wells. Geochemical data such as richness and quality of organic matter was provided by different laboratory studies, however, most of them by the Instituto Colombiano del Petroleo (ICP).
Thermal modeling of the northern area
The well Río de Oro-14 in the Río de Oro field was modeled in this area. The field is located in the extreme north of the basin and the trap is a faulted anticlinal of Cenozoic age.
The crudes oils of this field show API gravity between 31º – 47°. The highest production comes from the Tibú Formation. The producer horizons are at depths between 4338 m in the Río de Oro-24 well, and 2733 m in the Río de Oro-22K.
In this well, the formation temperatures were modeled from a set of five BHT records. Based on the calculated formation temperatures and assuming a surface temperature of 80°F (299,81K) we estimated a current heat flow of 50 mW/m2. Then we determined the maturity profile in the well, using measured Ro values. The results reflect a relatively hot history.
Using the current heat flow projected to the past for the thermal model, it reveals a good agreement between the measured maturity profile and the modeled maturity curve. A good fit to the maturity data was possible due to variable heat flow model (Figure 4), starting with rifting around 170 my, decreasing exponentially, and increaseasing again near the Eocene, related to an extensional event. The modeling included approximately 1500 m of erosion during a major unconformity in the early Tertiary. This modeling shows that expulsion started for the Capacho Formation in the Middle Eocene and ends around 5 my, while for the La Luna Formation the expulsion started in the Upper Eocene and ended also around five million years ago. The peak of generation is in the Middle Miocene (Figures 5, 6, and Table 2).
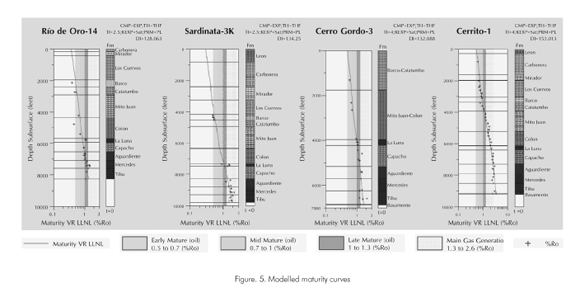
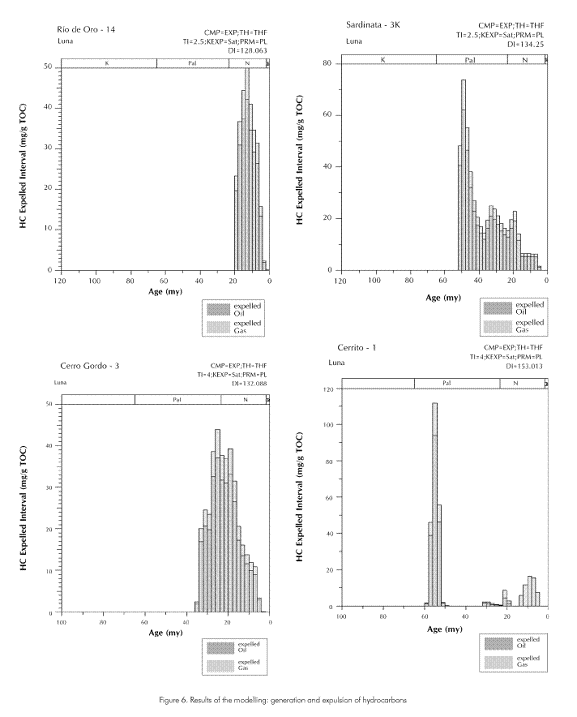
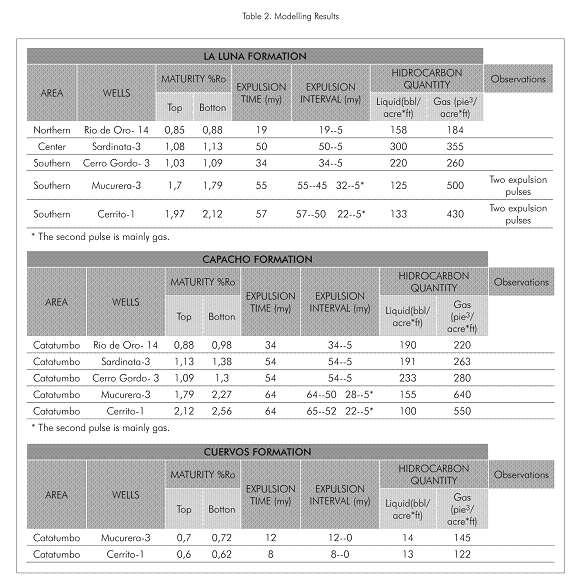
Thermal modeling of the central area
The Sardinata field (Figure 1) is located towards the central area of the basin in a trap type anticlinal. Their crude oils are between 27,5° and 50° API.
The current calculated heat flow in the Sardinata-3 well is close to 40 mW/m2, significantly lower than the heat flow observed north of the basin. The best match between measured maturity data and the modeled maturity curve (Figure 5) was obtained, just as in the north of the basin, from a rift-type model with exponential decrease in heat flow and increase towards the Eocene, associated with the extensional event, with approximately 608 m of erosion during the major unconformity.
In this model hydrocarbon expulsion started for the Capacho formation at 58 my, and in the La Luna Formation at 52 my. However, for both formations the significant expulsion continues to 16 my (Middle Miocene), and ends five million years ago. (Figures 5, 6, and Table 2).
Thermal modeling of the southern area
The Cerro Gordo-3, Mucurera-3 and Cerrito-1 wells were modeled for this area. Cerro Gordo-3 and Cerrito-1 produce gas from fractured reservoirs in the La Luna Formation. The Ro data support a thermal history with high maturity and the current heat flows were estimated as 57, 52 and 55 mW/m2 respectively.
The thermal history of this well, as well as those of the previous ones, was modeled starting with a rift type scenario, with exponential heat flow decrease from Lower Cretaceous and increase near the Eocene associated with an extensional event, with approximately 2128 m of erosion during the major unconformity.
The expulsion of hydrocarbons (Figures 5 and 6) started for the Cerro Gordo-3 well in the Capacho Formation at 54 my and in the La Luna Formation at 36 my; the major phase of expulsion is between 40 and 16 my, and between 30 and 12 my, respectively.
For the Mucurrera-3 well, the expulsion of hydrocarbons in the Capacho Formation started at 64 my and ended at 5 my. For the La Luna formation it started at 55 my and ended at 5 my. For the Cuervos Formation, it started at 12 my and it is still active. (Figures 5, 6, and Table 2).
For the Cerrito-1 well, expulsion started 60 my ago, with important expulsion in the Miocene. The Los Cuervos Formation shows generation and expulsion since 10 my (Figures 5 and 6).
OIL GEOCHEMISTRY
The geochemical composition indicates three families of crude oils:
Rio Zulia Oil Family (A)
Oil entrapped in the Mirador and Carbonera formations of the Río Zulia Field is characterized by high Pristane Phytane (Pr/Ph) ratios higher than 3, Oleanane/C30 Hopane ratio close to 0,3, and more abundant amounts of C29 than C27 Steranes. Characteristic of these oils is also low Sulfur, Vanadium and Nickel content, as well as a low Gamacerane/C30 Hopane and C35/C34, Hopane ratios (Figure 7 and Table 3).
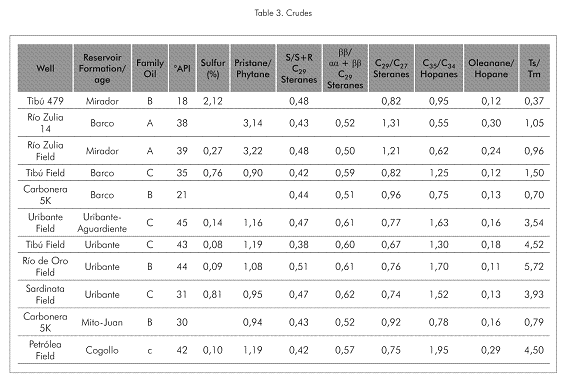
The composition of these crudes according to Didyk, Simoneit, Brassel, and Eglinton (1978) ; Mello, Gaglianone, Brassel, and Maxwell (1988) suggests an origin from an environment with mainly suboxic conditions, possibly deltaic, with a high contribution of organic material derived from land plants.
Peters and Moldowan (1993), indicate that Pr/Ph values higher than 3,0 in crudes and rocks at peak of expulsion indicate oxic conditions and contribution of terrestrial organic matter. In addition, these authors suggest that values above 0,3 for the Oleanane/C30 Hopane ratio indicate organic facies of the Later Cretaceous or Tertiary age, deposited in deltaic marine environment where there is a significant contribution of terrestrial organic matter. Waples and Machihara (1991), thought that a relatively high proportion of C29 Steranes signify crudes derived from deltaic or proximal marine sequences. All these characteristics are consistent with the Río Zulia oils being derived from a source rock deposited in a deltaic marine environment such as the Los Cuervos Formation.
Carbonera crude oil family (B)
This family comprises crude oils of the Barco Formation, Mito Juan and Uribante Group of the Carbonera and Tibú oil fields, Figure 1. Their characteristics are near 1,0 values of Pr/Ph, Oleanane /C30 Hopane values from 0,05 to 0,15, low proportions of C29 Sterane and C35/C34 Hopane ratio lower than 1,0 (Figure 7).
According to these characteristics, these crudes probable derived from a source rock deposited in an anoxic siliciclastic marine environment, with little contribution of terrestrial organic matter and can be correlated with siliciclastic Cretaceous formations rich in organic matter, such as the Capachos Formation.
Petrólea-Tibú crude oil family (C)
This family consists of crudes oils trapped mainly in Cretaceous reservoirs such as the Uribante Group, and the Cogollo-La Luna formations of the Uribante, Petrólea, and Tibú oil fields. This family is characterized by low contents of Sulfur, Pr/Ph ratios close to 1,0 Oleanane/ C30 hopane ratios of 0,1 to 0,3, relatively low proportions of C29 Steranes and C35/C34 Hopane ratios above 1,0 (Figure 7 ).
The major differences of this family with the Rio de Oro family are the increase in the C35/C34 Hopane and Ts/Tm ratios to values above 1,0 and the increase in the proportion of C27 Steranes. These crude oils are probably associated to source rocks deposited in an anoxic marine environment, with relative high carbonate input and a lower contribution of terrestrial organic matter. They may correlatable with the La Luna Formation.
Thermal evolution of crudes
The degree of thermal evolution of the crudes measured through the isomerization ratios of the C29 steranes (Waples & Machihara, 1991; Seifert & Moldowan, 1978) indicate that the crude oils from the Cretaceous reservoirs (Uribante Group Cogollo and La Luna) in the Petrólea, Cerro Gordo and Tibú fields have already reached their thermal equilibrium values. The crude oils located in the Barco Formation of the Rio Zulia Carbonera, Tibú and Río de Oro fields show a lower level of thermal evolution.
The percentage of aromatization, (Mackenzie, 1984), between 0,6 and 0,8 indicates that all of these crudes were generated at a relatively high thermal maturity (>Ro 0,9%). The Methyl-Phenantrene Index (MPI) generally shows values above 0,6, which suggests that most of these crude oils are mature, generated from a parent rock at a level of maturity above Ro 1,2%.
Thermal evolution of natural gases
Seven natural gases from Catatumbo Subbasin were analyzed for isotopic and molecular composition (Table 4), to assess their level of maturity and generation history.
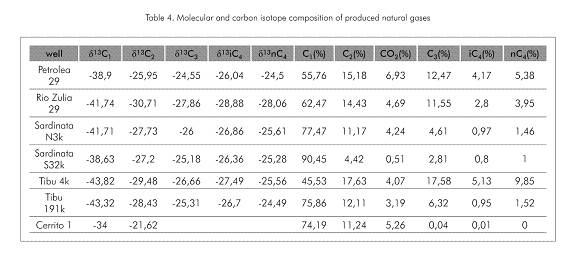
The hydrocarbon composition of the samples shows a relatively high concentration of C2+ components with (C1/C2+C3) ranging from 1,29 to 12,51, indicating that the gases should be classified as wet. Using the scheme of Shoell (1983) the gases can be classified as thermogenic (Figure 8).
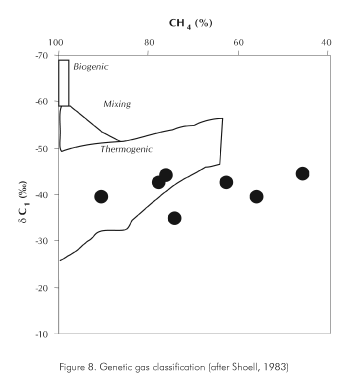
Mild biodegradation can be inferred from the stable carbon isotope compositions of propane and n-butane, which appear in some samples slightly enriched. This enrichment is suggested by nonlinearity when the carbon isotope composition is plotted against the reciprocal of the carbon number (Figure 9, Chung, Gormly, & Squires, 1988). However, applying the interpretative approach proposed by Prinzhofer and Huc (1995), Prinzhofer and Pernaton (1997), Prinzhofer, Mello, da Sila Freitas, and Takaki (2000) (Figure 10), it is possible to conclude that bulk composition and isotopic signature of hydrocarbon gases was not substantially altered by secondary processes such as segregational migration or biodegradation.
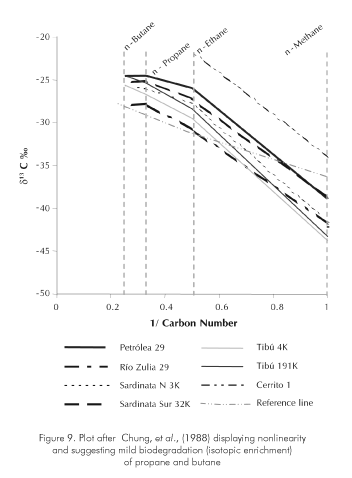
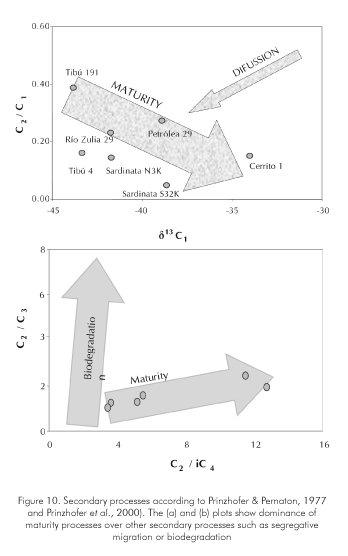
Based in the above it was assumed that isotopic signature was primary controlled by the degree of thermal evolution. The Whiticar (1994) model was then used to assess the degree of thermal evolution of the analyzed gases (Figure 11). This approach describes the isotopic signature of methane, ethane and propane generated from type II kerogen as a function of maturity. Using this scheme, the studied gases show a wide range of thermal evolution: the samples from Río Zulia 29, Tibú 4, Tibú 191 contain isotopic ratios indicating a moderate degree of thermal evolution, within the oil window. The gases from Sardinata and Petrólea wells have isotopically heavy methane which indicates a higher degree of maturity, within the beginning of the gas window. The Cerrito 1 well shows the highest degree of maturity, around 2,5 of equivalent vitrinite reflectance compatible with the end of the gas window.
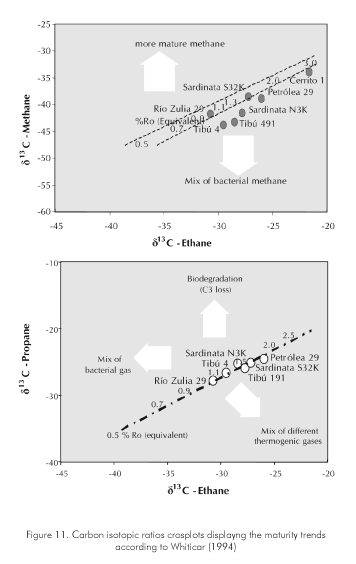
According to these results, the source rocks of the northern of Catatumbo sub- basin have not reached high thermal maturity, while, towards the southern end of Catatumbo subbasin the source rocks are more thermally evolved and are in the gas window.
EXPLORATORY IMPLICATIONS
The generation of oils in the Cretaceous intervals started at the end of the Eocene and beginning of the Oligocene and the maximum expulsion phase was reached in the Middle Miocene (Figures 5, 6 and 12). At the end of the Miocene the generation and expulsion processes ceased in the uplifted areas while in the axes of synclines these processes continued without interruption until now, generating light oil and gas in the more thermally mature areas (Figure 13).
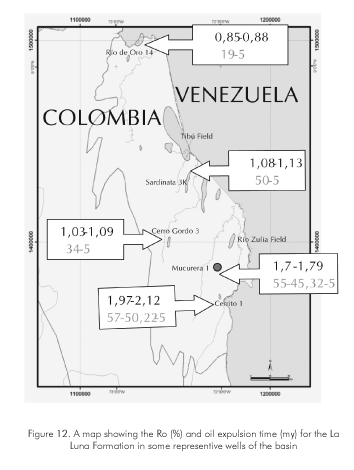
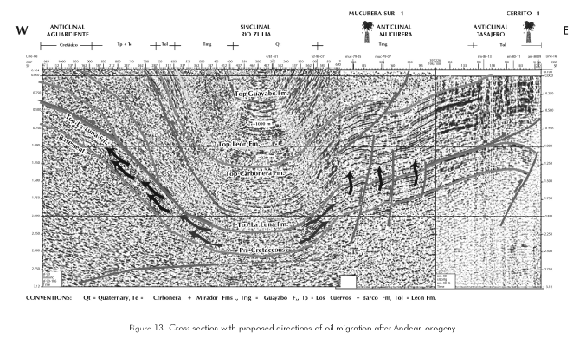
Concerning the migration of hydrocarbons, the presence of a potentially prolific source rock such as the La Luna Formation throughout the basin, and the ubiquitous presence of solid bitumen, indicates that local generation and migration within the subbasin are the predominant processes rather than long distance migration from the Venezuelan area of the basin. However, it is likely that a contribution from source rocks in Venezuela could have contributed to charge the more eastern structures, after the last orogenic event.
On the other hand, the thermal modeling of the Cuervos Formation indicate the possibility of present day generation and migration, particularly in the deeper areas with a thick overburden of sediments (i.e. the Rio Zulia aea)
With relation to trap formation and oil generation time, the data suggest that there is a good confluence of events for the structures existing at the end of the Cretaceous-Tertiary, in particular favouring the accumulation of hydrocarbons generated during the Eocene-Oligocene. There is also good correspondence for the structures of the Andean Orogen and the last migration of the youngest generated oil.
CONCLUSIONS
• The La Luna and Capacho formations are the main regional source rocks, with a marine carbonate origin and siliciclastic marine origin respectively. The Los Cuervos Formation is a presently generating source rock with high fraction of terrestrial organic matter.
• In the north and centre of the basin, the hydrocarbon expulsion from the Capacho Formation, starts in the Paleocene-Middle Eocene, while for the La Luna Formation expulsion started in the Lower Eocene-Upper Eocene. The peak of generation is in the Middle Miocene. In the southern sector expulsion started in the Paleocene with an important expulsion phase in the Miocene. The Los Cuervos Formation shows generation and expulsion since 10 My. In the synclines axes, and generation continues until the present.
• The gases in the Catatumbo basin are of the wet thermogenic type and thermal maturity varies from around 1,0 to 2,5 Ro equivalent. The higher maturity is in the gases of the southern area; therefore the prospectivity in this sector is basically for wet gas, while towards the center and north of the basin, crude oil can be expected.
• The accumulation of hydrocarbons mainly be explained mostly by generation within the basin and short-distance migration. The oil fraction expelled during the Paleocene-Miocene probably accumulated in the structures that formed since the end of the Cretaceous. A smaller long distance migration component from outside the subbasin, from the Venezuelan sector, towards the younger structures at the east of the Catatumbo sub basin is likely.
ACKNOWLEDGEMENTS
We are grateful to anonymous reviewers for helpful suggestions and Luis Fernando Peña for kerogen preparation for the front page microphotography.
BIBLIOGRAPHY
Allen, P. A., & Allen, J.R. (1990). Basin analysis - principles and applications. (2nd ed.). Black Well Publishing, 2005, 549p. [ Links ]
Bueno, E., & Pinto, J. (1997). Geología estructural del alto de Icotea, Lago de Maracaibo. Memorias VIII Congreso Geológico Venezolano, 1: 133-140. [ Links ]
Chung, H. M., Gormly, J. R., & Squires, R. M. (1988). Origin of gaseous hydrocarbons in subsurface environments: theoretical considerations of carbon isotope distribution. Chemistry and Geology, 71: 97-103. [ Links ]
Didyk, B.M., Simoneit, B.R.T., Brassel, S.C. & Eglinton G. (1978). Organic geochemical indicator of paleoenvironmental conditions of sedimentation. Nature, 272: 216-222. [ Links ]
Ecopetrol-ICP (2001). Evaluación de la Cuenca del Catatumbo. Reporte interno División de Exploración y Explotación, Ecopetrol-ICP. 9 Volúmenes. [ Links ]
Ecopetrol-ICP (1998). Evaluación de la Cuenca del Catatumbo. Report interno División de Exploración y Explotación, Ecopetrol-ICP. [ Links ]
Ecopetrol-ICP (1993). Evaluación de la Cuenca del Catatumbo. Report interno División de Exploración y Explotación, Ecopetrol-ICP. [ Links ]
Fimlay, C., & Gou Y. (1997), Inversion tectónica de la falla de Pueblo Viejo. Memorias VIII congreso Geológico Venezolano, 1: 267-277. [ Links ]
Llerena, J. & Marcano, F. (1997). El sistema petrolífero en la subcuenca de Catatumbo, Cuenca de Maracaibo, Venezuela. VI Simposio Bolivariano, Caracas, 540-551. [ Links ]
Macellari, C. (1988). Cretaceous paleogeography and depositional cycles of western South America. Journal of South American Earth Sciences, 1: 373-418. [ Links ]
Marcano, F., & Cassani, F. (1994). Modelado de cuencas y biomarcadores en la reconstrucción de procesos geoquímicos. V Simposio Bolivariano, 414-431. [ Links ]
McKenzie, D. (1978). Some remarks on the development of sedimentary basins. Earth and Planetary Science Letter, 40: 25-32. [ Links ]
McKenzie, A., S. (1984). Aplication of biological markers in Petroleum Geoquemistry. In J. Brooks and D. Welte (eds.) Advance in Petroleum Geochemistry, 1: 115-214. [ Links ]
Mello, M.R., Gaglianone, P.C., Brassel, S.C., & Maxwell, J.R. (1988). Geochemical and biological marker assessment of depositional environmets using Brasilian offshore oils. Marine and Petroleum Geology, 5: 205-233. [ Links ]
Mondragón, J. C., 2001. Proyecto regional de la cuenca del Catatumbo. Gerencia de Occidente, vicepresidencia adjunta de exploración. Reporte Interno, Ecopetrol S.A. – Instituto Colombiano del Petróleo (ICP). Informe técnico anual 2000, 9645. [ Links ]
Mora, C. A. (2000). Evaluación del potencial de los sistemas petrolíferos en las cuencas cretácicas con producción comercial en Colombia. Tesis de Maestría,Universidad Federal do Rio de Janeiro, Brasil, 250pp. [ Links ]
Parnaud, F., Gou, Y., Pascal, J. C., Capella, M., Truskowski, I., & Passalacqua, H. (1995). Stratigraphic Synthesis of Western Venezuela. In: A.J. Tankard, R. Suárez and H. J. Welsink (Eds.), Petroleum Basins of South America, American Association of Petroleum Geologists Bulletin, Memoir, 62: 681-698. [ Links ]
Pindell, J., George, R., Kennan, L., Cristancho, J., & Higgs, R. (1998). The colombian hydrocarbon habitat: Integrated Sedimentology, Geochemistry, Pelogeographic Evolution, Geodynamics, Petroleum Geology, and Basin Analysis. Tectonic analysis para Ecopetrol. Ecopetrol- Instituto Colombiano del Petróleo (ICP). Reporte interno, 416. [ Links ]
Peters K., & Moldowan M. (1993). The biomarker guide. interpreting molecular fossils in petroleum and ancient sediments. Prentice Hall, Inc., 363pp. [ Links ]
Prinzhofer A., & Huc A.Y. (1995). Genetic and post-genetic molecular and isotopic fractions in natural gases. In Rice, D.D. and Schoell, M. (Eds), Sources of Natural Gas, American Association of Petroleum Geologists Bulletin Memoir. [ Links ]
Prinzhofer, A., Mello, M. R., da Sila Freitas, L. C. & Takaki, T. (2000). A new geochemical characterization of natural gas and its use in oil and gas evaluation. In Mello M. R. and Katz, B. J. (Eds.), Petroleum Systems and South Atlantic Margins. American Association of Petroleum Geologists Bulletin, Memoir 70:107-119. [ Links ]
Prinzhofer, A., & Pernaton , E. (1997). Isotopically light in natural gas: bacterial imprint or diffusive fractionation. Chemical Geology, 33 (4), 193-200. [ Links ]
Rodríguez I., & Navarro, A. (1997). Nueva frontera exploratoria en la cuenca petrolífera del lago de Maracaibo: Zulia oriental, Venezuela occidental. Sexto Simposio Bolivariano Exploración Petrolera en Cuencas Subandinas, Colombia, 565-581. [ Links ]
Schoell M. (1983). Genetic characterization of natural gases. American Association of Petroleum Geologists Bulletin, 67 (12), 2225-2238. [ Links ]
Seifert,. W. K., & Moldowan, J. M. (1978). Applications of steranes, terpanes and monoaromatics to the maturation, migration and source of crude oils. Geoch. Cosmoch. Acta, 42: 77 95. [ Links ]
Sweeney, J.S., Talukdar S., Burnhan A., & Vallejos C. (1990). Pyrolisis kinetics applied to prediction of oil generation in the Maracaibo basin, Venezuela. Organic Geochemistry, 16:189-196. [ Links ]
Talukdar, S., Gallango, O., Vallejos, C., & Ruggiero, A. (1987). Observations on the primary migration of oil in the La Luna source rocks of the Maracaibo basin, Venezuela. In: Migration of hydrocarbons in sedimentary basins. Technip, Paris, 59-78. [ Links ]
Talukdar, S. C. & Marcano, F. (1994). Petroleum systems of the Maracaibo basin, Venezuela. In Magoon, L. B. And Dow, W. G. (Eds.) The Petroleum System – From Source to Trap. American Association of Petroleum Geologists Bulletin, Memoir 60:463-481. [ Links ]
Talukdar, S., O. Gallango, & Chin-A-Lien (1985). Generation and migration of hydrocarbon in the Maracaibo basin, Venezuela; Segundo Simposio Bolivariano Exploraión Petrolera en Cuencas Subandinas, Colombia, 2: 44. [ Links ]
Villamil, T., & Pindell J. (1998). Mesozoic paleogeographic evolution of northern south america: foundations for sequence stratigraphic studies in passive margin strata deposited during non-glacial times. In: Paleogeographic evolution and non-glacial eustasy, northern South America. SEPM Special Publication, 58: 283-318. [ Links ]
Waples, D.W. & Machihara, T. (1991). Biomarkers for geologists. AAPG Methods in Exploration Series, 9: 91. [ Links ]
Whiticar, M.J. (1994). Correlation of natural gases with their sources. In: Magoon, L. B. and Dow, W. G. (Eds.), The Petroleum System - From Source to Trap, AAPG Memoir 60: 261-283. [ Links ]
Yurewickz, D.A., D.M. Advocate, N.B. Lo, & Hernandez, E.A. (1986). Source rocksa and oil families, southwest Maracaibo basin (Catatumbo subbasin), Colombia. AAPG Bulletin, 82:1329-1352. [ Links ]
(Received, June 15, 2005; Accepted Aug. 24, 2007)