Introduction
Textile industries release considerable volumes of water-soluble waste substances because of fabric dyeing processes. This waste consists of dyes (azoic, disperse, anthraquinone, etc.) and dye-related compounds (surfactants, salts, polymers and solubilizing agents). Usually, these chemicals are discarded in the sewage, ending up in water bodies where they become a serious threat to the environment due to their chemical complexity and low biodegradability indexes (BOD5 /COD < 0.5). This BOD5 /COD is the ratio between the biochemical oxygen demand (BOD5) and the chemical oxygen demand (COD) of a given waste product. Biodegradability indexes, such as BOD5 /COD, depend on dye type, concentration and its mixture with other compounds.
Azoic dyes comprise an important type of industrial dyes extensively used for dyeing cotton-made fabrics. Structurally, azoic dyes consist of three main components, (i) a chromophore formed by three aromatic rings linked together by an azo bond, (ii) an auxochrome which modifies the chromophore's light absorption, and (iii) a solubilizer. Azoic dyes bind covalently to cellulosic fibers and can thus withstand temperature, friction, and pH changes (Kongliang et al., 2014; Satapanajaru et al., 2010). During fabric dyeing processes, a fraction (20% - 30%) of the dye is not attached to the fiber and it is therefore released, producing color effluents with high COD and BOD5, entailing considerable amounts of solids, and having alkaline pH values and high conductivities (Qingxiang et al., 2009; Waghmode etal., 2011). In complying with environmental acts and regulations, textile industries have adopted different strategies to minimize the impact of dye residues. These strategies consist of implementing physical, chemical, and biological wastewater treatment protocols, based on sedimentation, coagulation, activated sludge, anaerobic reactors, and bio-filtration. However, these protocols have proved to be effective only to certain extent (Waghmode etal., 2011), and they are not sufficient to reduce color and toxicity, deriving in documented adverse effects on aquatic ecosystems Jadhav et al., 2010; Punzi et al., 2015).
Complementary or sequential technologies can be used to improve conventional textile effluent wastewater treatments. Ligninolytic fungi and photocatalysis with TiO2 make suitable secondary and tertiary treatment options, respectively (Deveci etal., 2016). Ligninolytic fungi are more efficient than aerobic and anaerobic bacteria at removing and bio-transforming dye waste due to their oxidative enzymes with low substrate specificity (i.e. peroxidases and polyphenol-oxidases) (Morales et al., 2016; Morales et al., 2017; Rivera-Hoyos et al., 2013). This group of fungi is also capable of removing heavy metals and reducing COD and BOD5, thus increasing waste biodegradability ratios to values higher than 0.5 (Maalejet al., 2009; Puentes et al., 2012a; Sathian et al., 2014). However, they have certain limiting factors such as long processing periods, secondary sludge (biomass) production, and progressive fungal biomass inactivation as the system becomes saturated (Castillo et al., 2012).
Heterogeneous photocatalysis with TiO2 represents an attractive tertiary treatment application that can enhance the effectivity of ligninolytic-based protocols (Deveci etal., 2016). This technology uses natural or artificial light to produce two main photo-chemical reactions that take place at the TiO2 surface trough UV-light irradiation: (i) photo-induced redox reactions of adsorbed compounds and (ii) hydrophilic photo-induced conversion of TiO2. Complete or incomplete dye mineralization via electromagnetic radiation (> 3.2 eV) is based on electron hole pair production followed by recombination due to a charge transference (oxidation or reduction) process (Claudinei et al., 2015; Fernandez et al., 2015; Fernandez et al., 2016). Thus, a desirable sequential textile effluent wastewater treatment would use ligninolytic fungi to reduce high organic material and color, suspended solid concentrations, and facilitate photocatalyst action, coupled to a photocatalysis course that abates colored byproducts and decreases wastewater toxicity (Nogueira et al., 2015; Punzi et al., 2015).
The present study aimed at assessing a non-conventional biologic secondary textile wastewater treatment with an active ligninolytic fungal biomass coupled to a TiO2 physical-chemical tertiary treatment. To this end, ad hoc prepared TiO2 films were reused trough four consecutive cycles to study progressive loss of photocatalytic activity. Additionally, acute toxicity tests were performed before and after sequential treatment using the fresh-water polyp, Hydra attenuata, and Lactuca sativa, seeds as toxicity indicators. Finally, sequentially treated wastewater was utilized to carry out dyeing cotton fabric batch experiments in the laboratory.
Materials and methods
Characterization of wastewater
A set of physical, chemical and microbiological textile effluent wastewater parameters were evaluated before and after treatments (Table 1). All fungal biomass was removed from wastewater samples using a Thermo Scientific® Sorvall RC6 Plus centrifuge (10 800 g, 10 min, 4 ◦C), and the following parameters were measured in the supernatant: discoloration percentage, calculated on color units according to Pallerla & Chambers (1997); COD, calculated with the 5222D method (APHA, 2012); and BOD5, calculated with the 523D method (APHA, 2012). Further, conductivity and pH were determined using a digital sensor, and plate count agars were used to culture heterotrophic bacteria (CFU mL-1) (Fernandez et al., 2015).
Secondary treatment with ligninolytic co-culture
Fungal strains of Pleurotus ostreatus (HPB/P3) were obtained from the culture collection at Pontificia Universidad Javeriana (Bogotá, Colombia), and Phanerochaete chrysosporium (CDBB-H-298) were obtained from the culture collection of Department of Biotechnology and Bioengineering, CINVESTAV-IPN (México D.F., México). The two strains were cultured in wheat bran extract agar (WBEA) and wheat bran extract broth (WBEB) according to Morales etal. (2017). The inoculum was incubated for eight days at 120 rpm and 28 ◦ C. Viable Biomass was filtrated using Whatman filters No. 3, washed twice with distilled water and collected (VBLCC). Inactivated or non-living biomass (NLBLCC) was also included in the experiments. To this end, viable biomass was inactivated after autoclaving at 1 atm and 121 ◦ C for 15 min and ascertained by culturing all treated mycelia on WBEA for 7 days at 30 ◦ C. After the incubation period, no mycelial growth was observed.
A 14 L bubble column reactor (pneumatic reactor) was inoculated either with 900 g of VBLCC or 900 g NLBLCC. Then, non-sterilized textile effluent wastewater (10 L/ or 71.5% of effective work volume) was added. Reactor operational conditions were set at 28 ◦C and air flow s et at 1 v .v.m. This experiment was carried out in triplicate, and samples were taken at 0 h, 6 h, 12 h, 18 h, 24 h, and 48 h. Laccase activity was determined on each sample following the methodology described by Tinoco et al. (2001), which measures Laccase activity in terms of 1 μmoL of oxidized ABTS per minute. Manganese peroxidase (MnP) activity was quantified by the methodology described by Santoyo et al. (2008), MnP activity is measured in terms of the production of 1 μmoL of oxidized demetoxiphenol (DMP) per minute. Experiments without ligninolytic co-culture were performed to assess the activity of bacteria already present in wastewater (WLCCbacteria). All samples were subjected to color removal and COD and BOD5 abatement analyses.
Tertiary treatment with TiO2 films/UV
Soda-lime glass cuts (3.5 x 7.0 cm) were used as film substrates. These substrates were washed and degreased according to the methodology by Puentes et al. (2012a). TiO2 films were prepared placing each Soda-lime glass substrate on a 9-cm diameter petri dish. A total of 20 mL of a TiO2/TIP (TiO2 USP/ titanium (IV) tetraisopropoxide solution was poured over each soda-lime glass substrate and allowed to dry during 5 h at 50 ◦C to form the film. Later, TiO2 films were thermally treated at 450 ◦ C for 1 h . TiO2 film surface characteristics were observed using Scanning Electron Microscopy (SEM) (JEOL JSM 6490-LV electron microscope). Crystallographic characteristics of TiO2 films (Anatase and Rutile phases) were determined by X-ray diffraction as in Fernández et al. (2016).
Two cubic reactors of 20 x 40 x 5 cm (width, length, and depth), were utilized. Each one was divided into two equally-sized sections (20 cm wide, 20 cm long, and 5 cm deep) to perform 2 experiments simultaneously under the same conditions (photoreactor 1 and 2). Air glass diffusers of 19 cm2, with 2-mm pores, were set inside each section. Then, UV radiation was administered using two mercury UV lamps (Philips TUV 18, 15 W, wavelength 254 nm) located parallel to each reactor, 10 cm away from the TiO2 films. Both reactors were placed inside a box made of galvanized metal sheets (60 x 50 x 30 cm) with an internal division to separate each reactor (Suppl. 1). Each section contained 8 TiO2 films (1000 mg TiO2) placed in contact with 400 mL of wastewater treated for 6 h with VBLCC of P. ostreaus and P. chrysosporium.
UV lamps were turned on following adsorption/desorption time under dark conditions during 20 min. A preliminary photocatalysis test was carried on samples that were previously subjected to VBLCC secondary treatment for 0 h, 6 h, 24 h, and 48 h. These experiments were performed in triplicate with 4 h UV irradiation. Downstream color removal percentage and COD abatement levels served to establish level analysis results on samples from this photocatalysis test, the conditions for the subsequent photocatalytic experiments.
Photocatalysis (TiO2 /UV), Photolysis (UV) and adsorption removal (TiO2 without UV light) experiments were performed in triplicate for 12 h. Color removal, COD and BOD5 abatement, and microbial inactivation assays were performed on samples taken every hour along the process.
TiO2 film recycling
The number of operational cycles of each TiO2 film w as empirically determined. To this aim, a batch of eight TiO2 (1000 mg TiO2) thin films was prepared per section, as previously described, and used in the treatment of wastewater previously subjected to secondary treatment for 6 hours (1 700 ± 34 CU and 2 345 ± 123 mg L-1 COD). The same TiO2 thin films were then reused for up to four tertiary treatment cycles. At the end of each cycle, wastewater was replaced. Wastewater from each tertiary treatment cycle was tested for discoloration and COD abatement. In addition, SEM was used to document differences between films at the beginning and end of four-cycle runs. Particular attention was payed to looking for microorganisms attached to the film's surface.
Toxicity tests
Acute toxicity tests with (L. sativa) seeds, variety Batavia Gran Lago, were carried out contrasting the toxicity of untreated wastewater, wastewater subjected to secondary treatment with ligninolytic co-culture, and wastewater subjected to secondary treatment and tertiary photocatalysis for 18 h (VBLCC/TiO2UV Sequential treatment). Additionally, TiO2 toxicity was also evaluated. Static acute toxicity tests, exposing L. sativa seeds for 120 h to the three types of wastewater were performed according to the methodology described by McInnis (1989). Toxic effects were recorded as percentage of germination inhibition. Furthermore, an acute toxicity assay was also performed with fresh-water polyps H. attenuata for 96 h following the methodology described by Trottier et al. (1997). Wastewater toxicity in H. attenuata was expressed as polyp mortality percentage at the highest wastewater dilution capable of driving the expected effect.
Cotton fabric dyeing tests with treated wastewater
Distilled water, drinking water, and sequentially VBLCC /TiO2UV treated wastewater were used in dyeing fabric experiments. This dyeing process was performed in the laboratory in quintuplicate. In each dyeing experiment, 35.8 mg of azoic dye (Reactive Black 5) were mixed with 28 mL of the test waters (distilled, drinking, and VBLCC /TiO2UV treated wastewater) in 250 mL flasks at 19 ◦ C. Each solution was heated up to 90 ◦ C and 700 mg of sodium chloride (additive) was added. Dye and additive were mixed during 10 min at 100 rpm, and then a piece (0.7 g) of fabric was immersed in the solution. Each cotton fabric piece (7.0 x 7.0 cm) was degreased and subjected to detergent and impurity elimination prior to immersion in the dyeing solution. Each tested dyeing solution, with one immersed cotton fabric, was then heated up to 90 ◦ C for 45 min and regularly stirred (each 15 min) with a 30-cm glass rod to guarantee uniform staining. Subsequently, all pieces of fabric were cooled down for 1 h at room temperature. The dye concentration (mg L-1) in the remaining solution was used to calculate the q of adsorbed dye by cotton fabric in mg g-1, see Eq. 1.
In Eq. 1, V is the volume of the dyeing solution, C is dye concentration (mg L-1), C o is the initial dye concentration (mg L-1), and x is the cotton piece weight in g. The reported q is the average of the five replicates per treatment.
Statistical Analysis
Normality and variance homogeneity of untreated and treated (secondary, tertiary, and sequentially treated) data were determined with Shapiro-Wilk and Levene tests. Kruskal-Wallis nonparametric tests were performed to establish whether there were differences between treatments. Statistical significance was determined at the 95% (a = 0.05) level. All analyses were conducted with the Statistical Analysis System (SAS 9.0). Data from each independent experiment were presented as the means ± standard deviation of the mean.
Results
Characterization of textile wastewater
All variables analyzed in textile effluent wastewater before and after treatments are shown in Table 1. Remarkably, untreated textile effluent wastewater variables such as color units, COD, BOD5, and pH showed values above levels permitted by local environmental authorities (i.e. Resolución 3957 of Secretaría Distrital de Ambiente, Bogotá - Colombia). Moreover, the effluents had heterotrophic bacteria with morphology associated with Gram-negative and Gram-positive bacilli. Thus, tested effluents were not considered apt for discharging.
Secondary treatment with ligninolytic co-culture
Ligninolytic co-culture experiments were undertaken to assess how effectively VBLCC, NVBLCC and WLCCbacteria inoculates in textile effluent wastewater reduced its color and its COD and BOD5. Experiments were carried without pH control under non-sterile conditions. In terms of discoloration efficiency at 48 h, treatments VBLCC, NVBLCC and WLCCbacteria resulted in color removal percentages of 80%, 35%, and 11%, respectively (Fig. 1A). Significant abatement of COD, by 92%, and BOD5, by 76%, were observed in the treatment with VBLCC at 48 h, whereas percentages of COD and BOD5 abatement obtained after treatment with NVBLCC at 48 h were moderate, 41% and 25% respectively. The treatment with WLCCbacteria removed as low as 30% and 10% of COD and BOD5 under the same operational conditions (Fig. 1B and C). Statistically significant differences between treatments were observed for color removal, COD and BOD5 abetment, and it was established that treatment VBLCC was more efficient (p < 0.0001) than NVBLCC and WLCCbacteria.
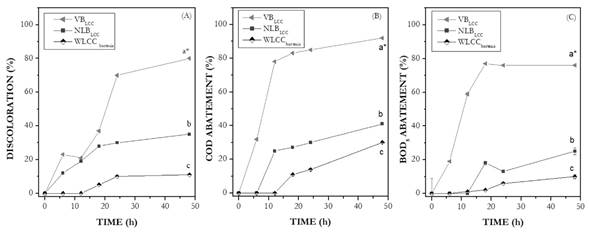
Figure 1 Effect of three ligninolytic secondary treatments on textile effluent wastewater as revealed by A) Wastewater discoloration, B) COD abatement, and C) BOD5 abatement. The three treatments were: Viable Biomass (VBLCC), Inactivated or non-living biomass (NLBLCC), and experiments without ligninolytic co-culture (WLCCbacteria) with operational conditions of: 1 L min-1 and 28 ◦ C. Low case letters in the plot: a* denotes the best treatment (i.e. VBLCC), followed in order b and c.
Ligninolytic enzymes played an important role in color removal and COD and BOD5 abatement. Laccase activity increased progressively reaching values of 452 U L-1 at 18 h of treatment. Later, laccase activity decreased to 410 U L-1 after 48 h of treatment. MnP activity reached a maximum activity value of 1 428 U L-1 at 48 h (Fig. 2A). In the WLCCbacteria treatment, Laccase and MnP activities were not detected. Wastewater alkaline values of 8.0 were observed at the beginning of the experiments; pH gradually decreased to 5.5 ± 0.5, probably due to consumption of simple forms of organic carbon (residual glucose: 0.1 g L-1 at 48 h) and production of citric and oxalic acids by fungi and bacteria (Fig. 2C). Bacterial growth was higher during treatment with VBLCC (10x1013 CFU mL-1) in comparison with the other two treatments, in which bacteria concentrations attained values of 10x108 and 10x109 CFU mL-1 for NVBLCC and WLCCbacteria, respectively (Fig. 2B).
Tertiary Treatment with TiO2/UV Characterization of TiO2 films
Fig. 3A and fig. B show SEM images of TiO2 films prepared at 50 °C and heat treated at 450 ◦C. Under low magnification (5000x), a full oxide semiconductor coating on the substrate and a film's uniform appearance was revealed. At higher resolutions (25000x and 50000x) a rough surface, presence of different aggregate sizes, various morphologies (spherical and ovoid forms), and small cracks were revealed. These cracks are probably caused by heat treatment at 450 ◦ C. The TiO2 films had signals matched with Anatase crystalline planes (101, 103, 004, 112, 200, 105, and 211) (Fig. 3C).
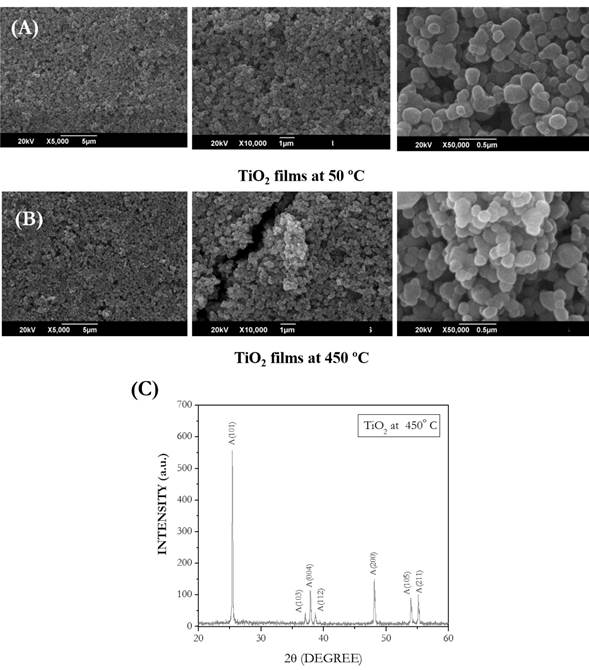
Figure 3 A) Scanning Electron Microscopy images at three amplification levels (5 000X, 10 000X, and 50 000X) of TiO2 films prepared by evaporation at 50 ◦ C. B) Scanning Electron Microscopy views at three amplification levels (5 000X, 10 000X, and 50 000X) of TiO2 films calcined at 450 ◦C. C) X-ray diffraction spectrum for TiO2 films deposited at a substrate and annealed at 450◦C.
Photocatalytic discoloration and COD and BOD5 abatement
Wastewater treated after 6 h, 24 h, and 48 h by VBLCC was used for preliminary photocatalysis tests. Photocatalytic treatment for 4 h resulted in a 25% discoloration and in COD and DOD5 abatement in wastewater (Fig. 4). Based on these results, photocatalytic experiments were performed for 6 hours on wastewater previously treated by VBLCC .
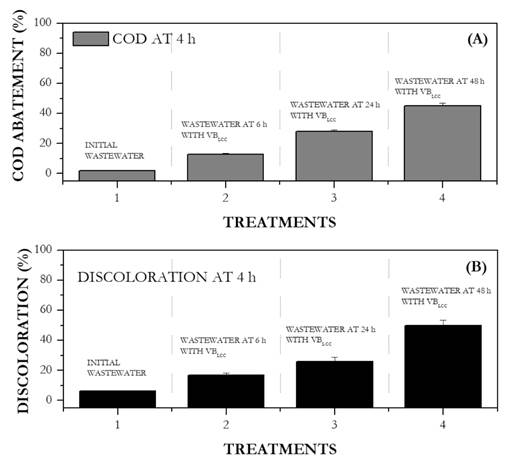
Figure 4 Effect of four secondary ligninolytic treatments on photocatalytic activity at 4 h, as evidenced on A) COD abatement percentage and B) discoloration percentage. Ligninolytic treatments: 1. Wastewater without any secondary treatment; 2. Wastewater at 6 h of secondary treatment or VBLCC; 3. water at 24 h of secondary treatment; and 4. water at 48 h of secondary treatment or VBLCC.
A maximum discoloration of 86% was obtained after 12 h of photocatalytic treatment of wastewater treated with VBLCC. The percentages of discoloration due to photolysis and adsorption were 45% and 33%, respectively (Fig. 5A). The largest COD abatement percentage (73%) was obtained by photocatalysis followed by photolysis with UV (25%) and adsorption (11%) (Fig. 5B). With respect to BOD5 abatement, the percentages obtained were 86%, 41%, and 16% for TiO2/UV, UV and TiO2/in dark, respectively (Fig. 5C). Under the evaluated conditions, statistical differences were found between treatments (p < 0.0001), establishing that discoloration, COD and BOD5 abatement observed after photocatalysis were higher than these obtained via photolysis and adsorption for 12 h. Moreover, the microbial population present in wastewater (9.0 x 109 CFU mL-1) was also reduced by photocatalysis. After 12 h of treatment 4.0 x 104, 4.0 x 104, and 9.0 x 109 CFU mL-1 were found for TiO2/UV, UV and adsorption respectively (data not shown).
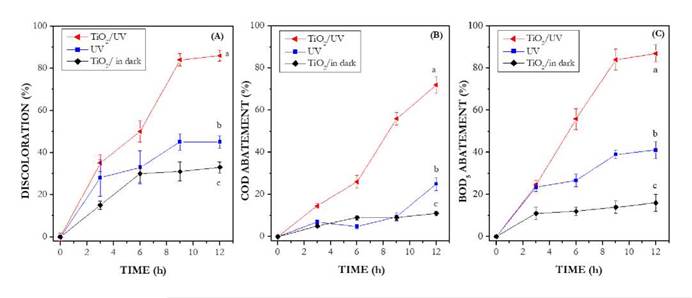
Figure 5 Discoloration a BOD5 and COD abatement experiments. Air 0.5 L min-1, pH 7.0, temperature 25 ◦ C.
The efficiency of the VBLCC-TiO2UV sequential treatment (secondary treatment with VBLCC for 6 h and tertiary treatment with TiO2UV for 12 h) was established based on the initial values of color, COD, and BOD5 levels of untreated textile effluent wastewater. Accordingly, total removal/abatement percentages were as high as 89%, 81% and 89% for color, COD and BOD, respectively. Additionally, a microbial inactivation of 53% was attained (Table 1).
Recycling of TiO2 films without thermal reactivation
After 12 h of tertiary treatment during test cycle 1 (C1) of the TiO2 films, a discoloration value of 81%. was observed. Reusing the same films in subsequent cycles led to decreeing discoloration percentage of 79%, 33%, and 32% for C2, C3 and C4, respectively (Fig. 6). Under the evaluated conditions, TiO2 films can be reused without thermal reactivation during two consecutive cycles. Concerning COD abatement and heterotroph bacteria inactivation, no statistical differences were determined between C1 (57% COD and 54% heterotroph inactivation) and C2 (56% COD and 51% heterotroph inactivation). In C3 and C4, COD abatement and heterotroph bacteria inactivation were less than 40%. Moreover, SEM images showed that bacterial biofilm formation increased from new (Fig. 6A) to C4 (Fig. 6B and Fig. 6C) TiO2 films. The bacterial morphology corresponds to Bacillus with terminal spores. Biofilm formation can be associated to gradual loss of photocatalytic activity, especially after C3 and C4.
Acute toxicity test
Wastewater without any treatment was toxic to both fresh-water polyps and letucce seeds. In the polyp, 100% at 50% lethality was observed. Moreover, acute toxicity tests with L. sativa showed 54% at 50% of inhibition. After comparing the toxicity of untreated wastewater with samples of those treated by sequential treatment (VBLCC/TiO2UV) during 18 h, toxicity on H. attenuata increase to (13% at 6.25%) and decreased on L. sativa (12% at 75%). Additional tests were performed in order to verify TiO2 toxicity at 1% (w/v). According to data, no adverse effects of TiO2 were noticed on L. sativa (0% at 100% by triplicated). On the other hand, acute toxicity tests on H. attenuata revealed that TiO2 lethality (56% at 12.5%). Most of the toxicity found on H. attenuata may be related to by-products formed during sequential treatment. Minor toxicity effects could be associated to TiO2.
Dyeing tests using treated effluent water
Regarding the amount (mg g-1) of adsorbed dye per gram of cotton fabric, no statistical differences were observed between wastewater treated with VBLCC /TiO2UV at 18 h, drinking water and distilled water (0.86 ± 0.02, 0.85 ± 0.04, and 0.88 ± 0.03 mg g-1; Fig. 7A). According to the visual assessment, the brightness and final aspect of the fabrics after dyeing were similar in all treatments. By the end of the process, pH values ranged between 8.0-8.5 and average conductivity was of 5.27 mS cm-1 (Fig. 7B and Fig. 7C).
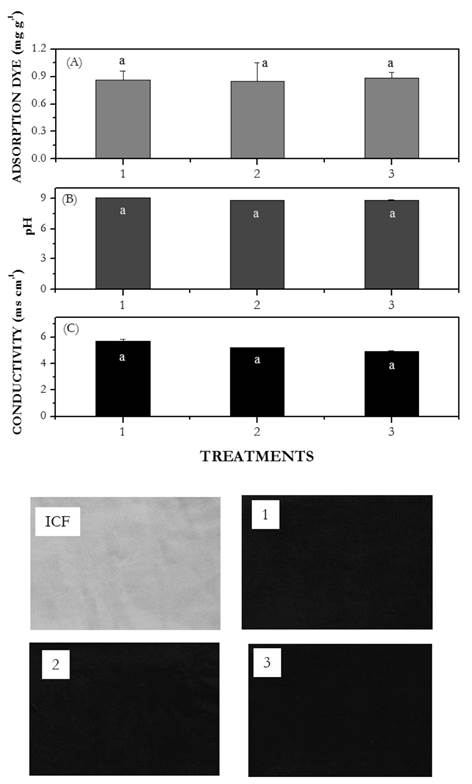
Figure 7 Reuse of sequentially treated wastewater in cotton fabric dyeing test. (ICF) Initial cotton fabric color. 1. Dyeing solution with treated wastewater with ligninolytic co-culture/TiO2 /UV at 18 h. 2. Dyeing solution with drinking water. 3. Dyeing solution with distilled water. Letters represent Tukey homogeneous subsets. a corresponds to best dyeing treatments.
This can be related to the presence ofsodium chloride acting as dyeing additive (Fig. 7B and Fig 7C). Moreover, the liquid residue obtained after dyeing tests may be treated by the sequential system and re-used in a new dyeing cycle. This will represent a zero-discharge system at the laboratory level.
Discussion
Secondary treatment with ligninolytic co-culture
Treating wastewater material with microorganisms can increase the efectivity of sequential or integrated oxidation (i.e. photocatalysis) procedures. This is possible because biological treatments can remove different charges of organic matter (Henao et al., 2011 and Punzi et al., 2015).
In the current work, the observed COD and BOD5 values were associated to the presence of five d ifferent azoic dye types i n textile effluent wastewater. Hence mechanisms of textile effluent w a s tewater d i s coloration a l s o led to abatement of COD and BOD5 values. Two mechanisms can relate to discoloration via VBLCC. First, dyes can be absorbed into fungal walls, which possess functional groups acting as ligands, (i.e. carboxyl, amine, hydroxyl, phosphate, thiol, etc.) (Morales et al., 2017; Puentes et al., 2012b). Second, following dye adsorption, ligninolytic enzymes can generate modifications in the chemical structure of the dyes. These enzymes can cleavage azo bonds (N=N) modifying chromophore groups, and therefore changing visible spectrum adsorption. Later, byproducts may be oxidized, reduced, demethylated, and hydroxylated producing benzene or substituted quinones (Maljaei et al., 2009; Osma et al., 2010).
The fact that NVBLCC showed some adsorption capacity can be a consequence of the heat treatment (autoclaving) itself. The heat treatment to which NVBLCC inoculates were subjected can generate a considerable number of functional groups that enhance color removal and led to the observed moderate COD and BOD5 abatement levels (Russo et al., 2010). Further, in the experiments without ligninolytic co-culture (WLCCbacteria), the observed levels of COD, BOD5 abatement and color removal were related to bacterial activity, probably as a result of enzyme activities such as peroxidases, azoreductases, riboflavin r e ductases, NADH-DCIP reductase and aminopyrine N-demethylase (Singh et al., 2015).
Positive interactions between fungi and bacteria have been previously described by Pedroza et al. (2018). These authors found that gram-positive and gram-negative bacteria, along with two white rot fungi (Pleurotus ostreatus and Trametes versicolor), can efficiently remove dyes from wastewater. Similar results were found in the present study, indicating that ligninolytic co-cultures can be used in bio reactors without specific s terile control conditions and that fungi are not inhibited by bacteria. Moreover, the presence of fungi may favor bacterial growth as evidenced by a bacterial biomass increase from 6.0 x 106 to 10 x 1010 CFU mL-1. Fungal ligninolytic enzymes are likely to attack complex molecules producing simpler ones that can be transformed by bacteria (Pedroza et al., 2018; Singh et al., 2015; Fig. 2A and 2B).
Concerning enzymatic activities, Laccase and MnP activity increased gradually. This may be related to an inducer effect caused by the dyes. In fact, the chemical structure of azoic dyes, which is made of aromatic rings, resembles that of phenyl propane units present in lignin. This has an enzyme enhancement effect. A possible scenario can be proposed in which the chromophore group is modified by the action of Laccase and then aromatic rings are transformed by MnP. This is drawn from findings by Morales et al. (2016) in which P. ostreatus laccase modified chromophore groups of Malachite Green, and then peroxidases were able attack aromatic rings.
Tertiary treatment with TiO2/UV
In the present study, preliminary tests showed that high concentrations of color and elevated COD values (as seen in wastewater without treatment) affected photocatalytic degradation. This can be explained by color and organic matter loads acting as a screen blocking the passage of UV light; thus affecting electron hole pair formation and leading to deficient rates of reactive oxygen species production. The effect of pollutant concentration on photocatalytic degradation has been previously reported by Puentes et al. (2012a). These authors evidenced that at high concentrations (80 mg L-1 and 100 mg L-1) of the azoic dye Reactive Black 5 (RB5), discoloration and COD abatement percents were as low as 15% and 10% , respectively, at 10 h of treatment. Conversely, at low pollutant concentrations (10 mg L-1, 50 mg L-1 and 70 mg L-1), discoloration was as high as 80% during the sames period of time.
After analyzing tertiary treatment results, it was noted that photocatalysis (TiO2/UV) was more efficient than ultraviolet light and a dsorption. The process was initiated by adsorption of residual dyes thanks to the pH conditions of the wastewater that facilitated residual dye transformation. This transformation is likey due to UV light interactions with the catalyst that lead to reactive oxygen species pruduction ending in dye structural modifications, namely at N=N and C-N b onds. Aditionally, this process would produce by-products like aromatic (phenols, amines, quinones etc), aliphatic compounds, and dissolved ions, such as NO- 3, N2 and SO4 2 (Henao et al., 2011; Puentes et al., 2012a).
The observed limited re-usability of TiO2 films c ould b e r elated to progressive inactivation of TiO2 excreted by adsorbed by-products onto the semiconductor's oxide surface. These by-products could block available active sites and prevent photonic excitation. Another aspect that could affect TiO2 performance at each usage cycle can be associated to bacterial population size (9.0 x 109 CFU mL-1). Bacteria can progressively cover TiO2 film surface creating biofilms. In addition, bacteria could behave like suspended solids at high concentrations blocking UV light. This assumption was confirmed by SEM of TiO2 films obtained prior and after TiO2 film usage cycle C4 (Fig. 7), in which a bacterial biofilm was observed. Previous studies have tested film reusability. Yue e et al. (2010) created a novel composite film made of Cds/TiO2/NTFs for methyl orange discoloration. This novel material removed 90% of color and endured five continuous usage cycles of 10 h each. However, in Yue e etal. (2010), a single dye at low concentration was evaluated, in contrast with the dye mixture used in our study.
Some toxic by-products could be produced after industrial wastewater treatment by combined technologies. These can sometimes be even more toxic to different organisms than the original untransformed compounds. Hence, it is important to verify water quality in terms of toxicity. Based on the current results, wastewater without any treatment was slightly toxic to H. attenuata and L. sativa, being H. attenuata more sensitive than L. sativa. This may be associated with the presence of azoic dyes, additive salts, and solubilizers which have been previously reported as toxic and potentially carcinogenic (Almeida & Corso 2014).
Wastewater toxicity on H. attenuata increased by the end of the sequential treatment, probably due to the presence of by-products formed during the process and the TiO2 washed off from the films. This compounds and particulate material would be absorbed by the target organisms, causing alterations in their digestive tract and producing morphological changes (Castillo et al., 2013, Allouni 2009, Rizzo 2011). The current results agree with the observation by Henao-Jaramillo et al. (2011) that the toxicity of an RB5 (300 mg L-1) solution increased after being treated with Trametes versicolor and photocatalysis with TiO2.
Lettuce (L. sativa) was less sensitive than H. attenuara, and responded more favorably to sequentially treated wastewater. L. sativa seeds were likely to be less sensitive to toxic effects due to a strong external layer made of hemicellulose and cellulose. These natural polymers tend to be hydrophobic and protect the seeds. Similar results are recorded by Puentes et al., 2012a who evaluated RB5 toxicity pre and post photocatalytic treatment. They showed that a solution of RB5 without treatment causes an inhibition percentage of 10% at 100%. This result was slightly different in comparison to the one obtained in the present study (56% at 50%). A possible reason for this difference couldbe the synergic toxic effect of the mixture of dyes and additives present in textile wastewater as opposed to that of a single dye.
Adsorption of RB5 takes place in 5 stages. First, the dye is adsorbed by cellulose fibers and then it diffuses in the matrix. Second, the dye is fixed to the fabric under favorable conditions of alkaline pH, additives and temperature.
Next, the dye attaches to the active sites of the fiber through covalent bonds (nucleophilic substitutions). The last stage is the final wash and d rying; in which unabsorbed dye is washed out from the fabric, and it acquires brightness and a uniform color (Sufian et al., 2 016). In the present work, just 20% of the dye was lost in the final wash o ut. On average, 30% dye losses could be expected in textile dye procedures, thus the results are promising and show that textile effluent wastewater recycling after treatment is possible. However, large scale studies should be performed to determine the optimal number of treated wastewater re-usage cycles. The process can help to reduce the volume of wastewater produced and is a good approach to zero discharge in the textile industry.
Conclusions
A laboratory-scale sequential system to treat textile wastewater was developed and evaluated. The present work showed that final textile effluent wastewater can be treated by subjecting it to a secondary treatment with a fungal co-culture for 6 h, coupled to a tertiary treatment consisting of photocatalysis with TiO2 for 12 h.
Thanks to this proposed sequential treatment, wastewater color was removed and COD and BOD5 abated. However, treated wastewater remained ostensibly toxic to fresh-water polyp, H. attenuata, and lettuce, L. sativa, seeds.
The reutilization of TiO2 films and treated wastewater was e valuated. TiO2 films can b e r eused f or t wo c ontinuous o perational c ycles a nd treated wastewater can be recycled and used in cotton fabric dyeing.