Introduction
The potential yield of common bean Phaseolus vulgaris L. (Fabaceae) is considerably reduced by diseases such as angular leaf spot caused by fungus Pseudocercospora griseola (Sacc.) Crous and Braun (Delgado et al., 2013). This disease can decrease production by up to 70% (Mora-Brenes et al., 1983), mainly due to defoliation and photosynthetic reduction of plants (Bergamin Filho et al., 1997; Jesus-Junior et al., 2001). Because of the favorable annual inoculum conditions of the pathogen in crops in southern Brazil, angular leaf spot is one of the most common diseases that affect grain yield in this legume.
The genetic resistance of beans to angular leaf spot has been the most recommended for being inexpensive, efficient, and less risky to the environment. However, the significant variability of the pathogen makes it difficult to obtain cultivars with high resistance levels (Pastor-Corrales et al., 1998). As a result, the disease has been managed mainly using fungicides, particularly some strobilurins that have shown a control higher than 80% (Sartorato & Rava, 2003). In the search for production technologies that are less harmful to humans and the environment, the use of natural products or resistance inducers has taken on an added importance in the area of plant protection (Wordell Filho et al., 2007).
An alternative for the management of plant diseases be macroalgae (Paulert et al., 2009). Despite their potential, macroalgae have been little explored as physiological stimulants or biotic stress-resistance inducers (Stadnik & Freitas, 2014). Within this context, it is known that preventively applied Ulva sp. extracts and their polysaccharides control fungal diseases in different species of agriculturally important plants. For example, aqueous Ulva sp. extracts reduce the severity of anthracnose (Colletotrichum lindemuthianum) in beans by 44% (Abreu et al., 2008), alternariosis (Alternaria porri) in small onion by 70% (Araújo et al., 2012), and powdery mildew in vines, cucumber, and beans by 77, 80 and 90%, respectively (Jaulneau et al., 2011). Apart from protecting bogie plants (Medicago truncatula) from fungus Colletotrichum trifolii, preventive applications of Ulva spp. can activate genes related to the biosynthesis of phytoalexins and proteins associated with pathogenesis and the cell wall (Cluzet et al., 2004).
In addition to resistance induction, the extracts of these algae seem to have a stimulating effect on plant physiology (Stadnik & Freitas, 2014). For instance, U. lactuca-derived liquid fertilizer improves nutrient absorption and increases growth, photosynthesis, and flowering of Tagetes erecta (Sridhar & Rengasamy, 2010). Also, it stimulates nitrogen metabolism in roots and shoots of Vicia faba L. (Fabaceae) (El-Naggar et al., 2005). The foliar application of the methanolic U. fasciata extract in bean seedlings grown in greenhouse conditions stimulates the growth and fresh mass of the aerial part of plants infected with anthracnose (Paulert et al., 2009).
Typically, pathogens can negatively affect leaf pigments by causing leaf lesions (Bassanezi et al., 2002). For example, C. lindemuthianum reduces the levels of chlorophyll and carotenoids in bean leaves (Lobato et al., 2009). These adverse effects can be avoided with the application of fungicides (Zhang et al., 2010) or alga extracts (Blunden et al., 1997).
Several studies with Ulva sp. extracts and its components, such as ulvana, have been conducted under laboratory conditions. However, no studies evaluate the efficacy of these derivatives under field conditions. Therefore, this paper aims to evaluate the effect of alcoholic U. fasciata extract on angular leaf spot severity, leaf pigment content, and grain yield of three cultivars of common bean of the black group.
Materials and methods
The experiments were carried out during 2005 (02/24–05/31) and 2006 (02/13–05/20) at the Experimental Station of the Empresa de Investigación Agropecuaria y Extensión Rural de Santa Catarina (Epagri), Ituporanga, Valle Alto de Itajaí region, SC, Brazil, located at 27 °38′ S and 49°60′ W at an altitude of 475 MSL.
Biological material and treatments
The present study used bean genotypes cv. IPR Uirapuru (IAPAR, Londrina-PR), CF 22, and Mouro graúdo (local materials) of indeterminate growth habit (type II). The plants were treated at phenological stages V3, V5, R6, and R7 with the alga extract (50 mg of dry mass/mL) or fungicide azoxystrobin 60 g ai/ha (Amistar., Syngenta), using a 20 L knapsack fumigator at 75 psi pressure as a maximum and 600 mL/min flow rate. Untreated plants were used as controls.
The alga extract was prepared according to the methodology of Abreu et al. (2008) with some modifications. Green macroalgae of the Ulva fasciata species were collected in February 2005 at Armação beach, in Florianópolis-SC (27.4454° S and 48.2956° W), soaked in running water, and quickly dried in an oven at 50 °C for 48 hours. The dry material was finely crushed, and the dry powder was kept in an opaque container at -20 °C. For the extraction of the compounds, ethanol (98%) was added to the dry powder at a ratio of 3.5:100 (p:v). After 24 hours, the extract was filtered on Whatman filter paper (# 4) and evaporated on a rotary evaporator at 40 °C. Finally, the aqueous fraction was collected, quantified, and stored at 4 °C until use.
Experimental conditions
Bean seeds, previously treated with carbendazim (45 g ai per 100 kg of seeds) + thiram (105 g ai per 100 kg of seeds), were sown in a direct sowing system on Secale cereale L. (Poaceae) remains for the agricultural seasons 2005 and 2006. Twelve seeds per linear meter were used, resulting in a population of 25 plants m-2. The experimental units consisted of a functional area of 7.2 m., each plot consisting of four lines that were 4 meters long and 0.45 meters wide. It was fertilized with the composition 5-20-20 (N-P2O5-K2O) in doses of 250 kg/ha. Weeds were controlled by applying the herbicide fluazifop-p-butyl (200 g ai/ha) + fomesafen (250 g ai/ha), and insect pests by spraying lambda-cyhalothrin (10 g ai/ha) at phenological stages V3 and V5.
Evaluation of angular leaf spot
For both years, angular leaf spot severity (%) was estimated weekly from phenological stage V3, totaling six evaluations during each campaign. A scale from 1 to 9 was used, where 1 = absence of symptoms and 9 = 100% affected leaf area (Marques-Júnior et al., 1997). The averages (scores) of each plot were transformed into a percentage and, later, integrated as the area under the disease progress curve (AUDPC), according to the trapezoidal integration equation described by Campbell and Madden (1990).
Determination of foliar pigments
Foliar pigments were determined in 2006 in ten fully expanded young bean leaflets, randomly collected in the two central rows of each plot at phenological stage R5. Necrotic areas (if any) and petiole were removed from each leaflet. For the extraction of foliar pigments, each 2 g sample of leaf tissue (fresh mass) was macerated in a mortar containing liquid nitrogen. 30 mL of acetone (85%) were quickly added, stirring the mixture for 2 min. The resulting extract was filtered using filter paper (Whatman 40, porosity: 8 µm) in a Büchner funnel coupled to a vacuum pump (flow rate of 2.2 m3/hour). The solution obtained was completed to 50 mL with 85% acetone. After homogenization, a 2 mL aliquot was removed, measuring absorbance at wavelengths of 646, 663, and 470 nm (corresponding to the light absorption of chlorophyll a and b, and carotenoids, respectively). All samples were analyzed in duplicate.
For calculating the levels of chlorophyll a and b, and total carotenoids, the equations of Lichtenthaler (1987) were used, with the pigment content of the extracts converted to μg/mL. With these values, total foliar pigments were subsequently calculated as follows:
Chlorophyll a (μg/mL) = (12.21 × A663) - (2.81 × A646) Equation 1
Chlorophyll b (μg/mL) = (20.13 × A646) - (5.03 × A663) Equation 2
Carotenoids (μg/mL) = [(1,000 × A470) - (3.27 × Ca) - (104.0 × Cb)]/229 Equation 3
Evaluation of grain yield and thousand-grain weight
The plants were harvested when they reached physiological maturity. The yield values obtained in 2005 and 2006 were transformed into kilograms per hectare (kg/h) and corrected to 13% humidity. In 2005 the mass of one thousand grains was determined.
Experimental design and statistical analysis
A randomized complete block design with a 3 × 3 factorial array (genotypes × treatments) was used with four replications. For data analysis, equal variance and normality of the residuals were verified using the Bartlett and Shapiro-Wilks tests, respectively. The AUDPC data were transformed for √x + 1. Upon meeting the assumptions, the data were subjected to two-way ANOVA. When there was a significant difference between the treatments, the Tukey test (. < 0.05) was performed to compare the means. All analyses were carried out with ASSISTAT, version 7.5 beta 2012 (Silva & Azevedo, 2002).
Results and discussion
The three genotypes used were susceptible to angular leaf spots (figures 1 and 2). This phenomenon occurs because none of them had cv. Ouro Negro, frequently used in breeding programs in Brazil, as it is a source of P. griseola-resistant genes (Pastor-Corrales et al., 1998; Sanglard et al., 2013; Sartorato, 2006). So far, only the susceptibility of cv. Uirapuru to angular leaf spot is known (Sartorato, 2006).
The highest final severity of the disease was observed in the Mouro Graúdo and CF 22 genotypes in 2005 and 2006, respectively, with significant interaction between genotypes and years (figure 1, p < 0.05). The pathogenic diversity of P. griseola in Ituporanga may have caused this behavior. Pathotypes 63-23, 63-31, and 63-63 have been reported for this location from a sample of eight isolates of this fungus (Sartorato, 2002). The tremendous genetic (Abadio et al., 2012) and pathogenic (Silva et al., 2008) variability of P. griseola makes it difficult to obtain cultivars with long-lasting genetic resistance. Possibly, the interaction between environmental factors and genotypes have also affected differently the defense responses associated with the basal resistance of genotypes to the pathogen (Walters & Heil, 2007).
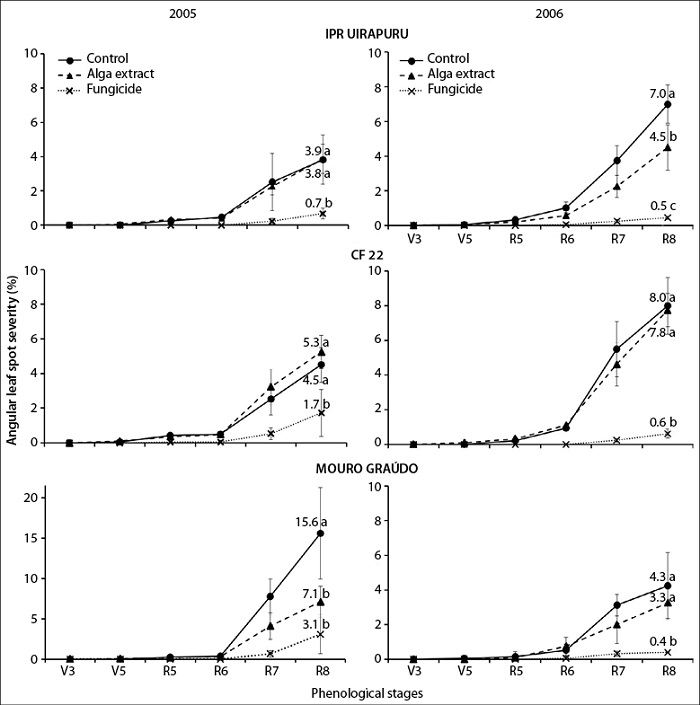
Source: Elaborated by the authors
Figure 1. Temporal progression of angular leaf spot severity in bean genotypes IPR Uirapuru, CF 22, and Mouro graúdo, treated with Ulva fasciata extract and azoxystrobin, in 2005 and 2006. Lowercase letters indicate a significant difference by Tukey’s test (p < 0.05) between treatments for each genotype in the last evaluation. Bars represent the standard deviation of mean severity
The U. fasciata extract decreased final severity (figure 1) and AUDPC (figure 2) of angular leaf spot in the Mouro graúdo (52%) and IPR Uirapuru (37%) genotypes only in 2005 and 2006, respectively. In these genotypes, the protective effect of the extract as reported for other diseases, such as anthracnose in beans (Colletotrichum lindemuthianum) (44%) (Abreu et al., 2008), alternariosis (Alternaria porri) in small onion (70%) (Araújo et al., 2012), and powdery mildew in vines, cucumber, and beans (77, 80, and 90%, respectively) (Jaulneau et al., 2011). However, Delgado et al. (2013) found no differences in the severity of angular leaf spot in beans between the plants treated with the alga ulvana polysaccharide (the main constituent of this alga’s cell walls). This fact denotes the importance of the results of this paper since it would be the first to report the control of this disease in beans under field conditions.
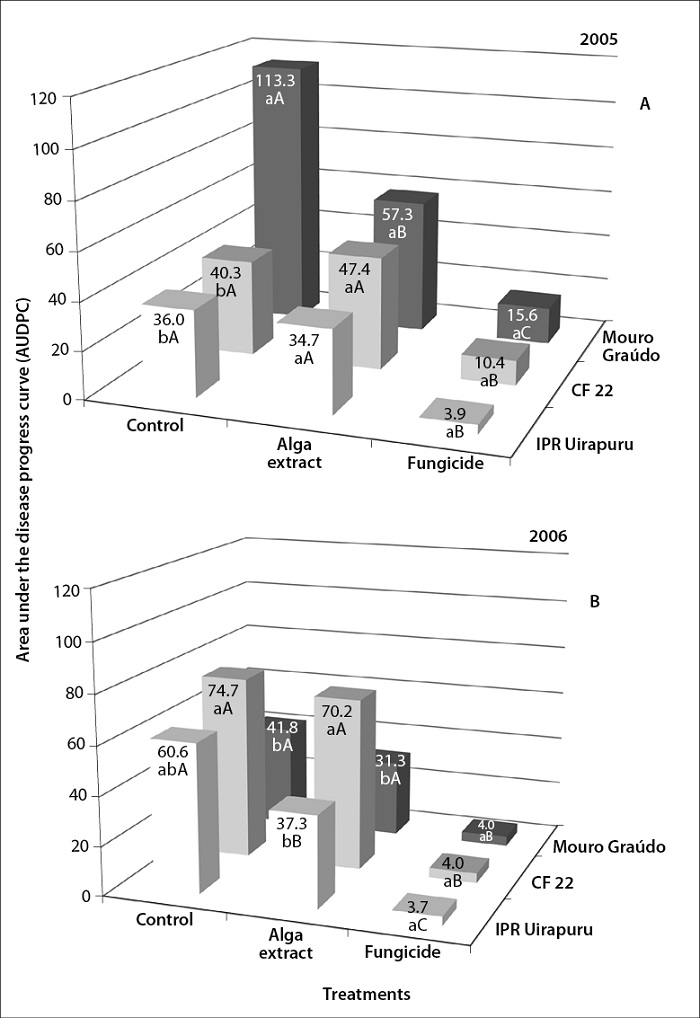
Source: Elaborated by the authors
Figure 2. AUDPC of angular leaf spot in the genotypes Mouro graúdo, CF 22, and IPR Uirapuru treated with Ulva fasciata and azoxystrobin in 2005 (A) and 2006 (B). The mean AUDPC values followed by the same lowercase letter in the treatments and the same uppercase letter in the genotypes did not differ statistically between them per Tukey’s test (p < 0.05)
For the years concerned, the fungicide significantly decreased (62-93%) the severity of the disease in all genotypes when compared with untreated controls. Besides, the alga extract reduced the severity of angular leaf spot in the Mouro graúdo (54%) and IPR Uirapuru (36%) genotypes only in 2005 and 2006, respectively (figure 1). Perhaps, the application of the U. fasciata extract may have induced resistance in both genotypes. It is known that in addition to protecting alfalfa plants from the fungus C. trifolii, preventive applications of Ulva spp. can activate genes related to the biosynthesis of phytoalexins and proteins associated with pathogenesis and the cell wall (Cluzet et al., 2004). Likewise, besides protecting bean plants against C. lindemuthianum, the ulvana polysaccharide (one of the components of the extract) increases the activity of peroxidase (POX) and glucanase (GLU) enzymes in resistant plants inoculated with water (Freitas & Stadnik, 2012). This polysaccharide also induces an initial oxidative explosion, activating the signaling pathways of salicylic acid, jasmonic acid, or ethylene in plants against pathogens (El Modafar et al., 2012: Vera et al., 2011).
As to the final severity (figure 1) and AUDPC (figure 2), there was a significant interaction between genotypes and treatments (p < 0.01). In biological terms, years and genotypes interacted since the Mouro Graúdo genotype exhibited the highest final severity of the disease and AUDPC in 2005, but a lower severity in 2006, when compared to the other genotypes.
For these two years, the fungicide significantly decreased (74-95%) the AUDPC in all genotypes, compared with the control (figure 2). Plants of the Mouro graúdo and IPR Uirapuru genotypes treated with alga extract during 2005 (figure 2A) and 2006 (figure 2B) showed a reduction of AUDPC of 49 and 38%, respectively, compared with the control plants. This result suggests that, while the extract affects the disease, this effect is intermediate and dependent on interactions with the genotype and the environment. Interactions between genotypes and components of the U. fasciata extract have been recorded in other pathosystems (Stadnik & Freitas, 2014). For example, the preventive treatment of bean plants with ulvana increased the activity of the GLU enzyme in cv. Pérola, moderately susceptible to U. appendiculatus, but not in cv. IPR Juriti, which is highly susceptible (Borsato et al., 2010). Previously, Delgado et al. (2013), in three consecutive experimental periods, reported that ulvana did not affect the development of the natural infection of angular leaf spot in two bean cultivars. Moreover, resistance induction can be affected by environmental conditions (Walters & Fountaine, 2009), which may influence the physiology of plants, the response to an attack by pathogens, and even the stability of the active components of the U. fasciata extract, such as ulvana (Delgado et al., 2013).
Although the levels of all foliar pigments were similar between genotypes and between treatments (fungicide and U. fasciata extract ), they showed general means of 6.2, 4.8, 11.0, and 0.7 μg/mL for chlorophyll a, b, total, and carotenoids, respectively (table 1); the content of these pigments in some plants can increase with the application of fungicides (Zhang et al., 2010) or alga extracts (Blunden et al., 1997). Even though the chemical group of the fungicide causes the so-called greening effect, observed mainly in grasses (Venancio et al., 2003), it did not increase pigments, which can be explained by the fact that foliar pigments were only determined at phenological stage R5, and not over time. For example, in applying various fungicides to the wheat crop, including azoxystrobin, Zhang et al. (2010) did not observe differences in the chlorophyll content when this fungicide was compared to the control (water) in initial stages (pre-flowering and full flowering). However, they did in advanced stages (milky and pasty grain).
Table 1. Levels of chlorophyll a, b and total, and carotenoids quantified 30 days after the first application (phenological stage R5) of the Ulva fasciata extract and fungicide in bean genotypes IPR Uirapuru, CF 22, and Mouro graúdo in 2006
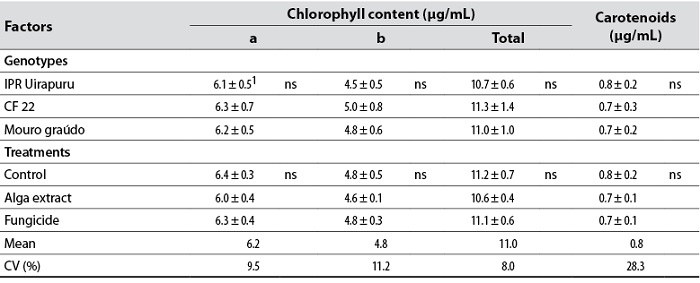
Note:1 Values represented by the mean ± the standard deviation of the mean of ten replicates; ns: The difference between genotypes or treatments is not significant.
Source: Elaborated by the authors
Angular leaf spot did not affect foliar pigment content in bean plants, although the negative relationship between the disease and the photosynthetic rate (Bassanezi et al., 2001) and the emission of fluorescent chlorophyll (Bassanezi et al. ., 2002) is known. Two hypotheses can be put forward to explain the discrepancies between this paper and those in the literature. The first is the percentage of the disease in leaflets. At the time of collection (R5) of leaflets for determining leaf pigments, angular leaf spot severity was less than 1%. According to Lopes and Berger (2001), in one of the experiments, the chlorophyll content in non-necrotic areas did not significantly decrease in bean leaflets with up to 20% anthracnose severity. Likewise, chlorophyll levels decreased markedly only in leaflets inoculated with rust whose severity was greater than 30%. The second hypothesis is the location of the pathogen in the leaflet. Chlorophyll is not significantly affected in the green area of bean leaflets with low levels of anthracnose severity (Lopes & Berger, 2001). Reduced photosynthesis in the host can be limited to the affected parts and adjacent regions (Moriondo et al., 2005).
The genotypes Mouro graúdo (433 g) and IPR Uirapuru (4,319 kg/ha) had the highest thousand-grain weight and grain yield in 2006, respectively. The application of fungicide increased thousand-grain weight by 13% and grain yield by 21% in 2005 and 12% in 2006, compared to controls (table 2). The alga extract did not increase these production variables, possibly due to the angular leaf spot control only in two genotypes and in different years. This hypothesis would be confirmed by the fact that the fungicide reduced the disease in the three genotypes for the two years, thus explaining the higher grain yield obtained in this article. This fungicide increases the emergence and vigor of plants, the number of total pods, and grain yield (Pynenburg et al., 2011), and controls anthracnose in beans. At the same time, there was no significant interaction between genotypes and treatments in these two variables (table 2).
Table 2. Thousand-grain weight (g) and grain yield (kg/ha) in bean genotypes IPR Uirapuru, CF 22, and Mouro graúdo treated with the Ulva fasciata extract and fungicide in 2005 and 2006
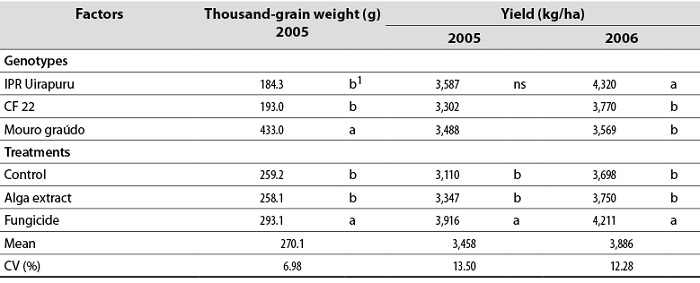
Note:ns: Not significant in the column; 1Means followed by the same letter do not differ statistically per Tukey’s test (p < 0.05).
Source: Elaborated by the authors
The U. fasciata extract seems to produce an effect on angular leaf spot, but unstable, being dependent on interactions with the genotype and the environment. In the future, it will be necessary to study how to increase the efficiency of the alga extract (formulation) by evaluating its impact on the physiology of other cultures and their diseases (foliar or root pathogens) under field conditions.
Conclusions
In the experimental conditions of this paper, it was found that the control of angular leaf spot by the Ulva fasciata extract depends on the biological interaction between the bean genotype and the environment. Furthermore, the U. fasciata extract did not affect leaf pigment content, thousand-grain weight, or grain yield.