Introduction
Microencapsulation is a technique used to protect active and nutraceutical compounds (Ribeiro et al., 2020) and consists in uniformly coating functional ingredients (vitamins, enzymes, phenols, molecules, and cells) with a polymeric film to obtain micrometric capsules (Dhakal & He, 2020; Ozkan et al., 2019; Ribeiro et al., 2020). The encapsulation process can be carried out with gases, liquid drops, or small solid particles (Ozkan et al., 2019). The film acts as a barrier to protect the active compound from the surroundings, control its release, avoid interaction with other ingredients, and improve its stability (Ribeiro et al., 2020).
Bioactive compounds are mostly secondary metabolites separated from natural products such as animals, plants, fungi, and microorganisms (Banožić et al., 2020; Zhang, Wen, et al., 2020). They are commonly used for treating diseases and manufacturing functional foods (Banožić et al., 2020; Zhang, Wen, et al., 2020). Functional foods are products that incorporate or enhance nutritional ingredients with potential health benefits, such as foods with additional nutrients or components, foods in which a harmful component has been eliminated, replaced, or reduced, and foods whose composition has been naturally improved (Contreras-Rodríguez et al., 2020).
Numerous active ingredients are known to improve human health: dietary fiber, oligosaccharides, sugar alcohols, amino acids, peptides, and proteins; glycosides, alcohols, isoprenes, and vitamins; hills, lactic acid bacteria, minerals, unsaturated fatty acids, phytochemicals, and antioxidants (Ye et al., 2018). This review focuses on essential microencapsulated components such as vitamins, minerals, oily substances, antioxidants, and probiotic microorganisms sensitive to conditions such as heat, light, and low pH (Lucas et al., 2020; Ye et al., 2018).
The direct incorporation of bioactive and nutraceutical compounds in food matrices has difficulties related to low solubility, defects in product quality (negative changes in color, texture, taste, and appearance), loss of functionality caused by food processing and storage operations, and degradation by digestive enzymes and unfavorable environmental conditions (oxygen, light, and temperature) (Mohammadian et al., 2020). Microencapsulation is a technological solution to optimize the preservation of active ingredients in raw materials and food during processing and storage (Favaro-Trindade et al., 2020; Ye et al., 2018). Several microencapsulation strategies have been introduced to reduce its thermal deterioration and achieve its incorporation and controlled release in the body, such as spray-drying (Lucas et al., 2020; Ye et al., 2018).
Spray-drying is the oldest microencapsulation technique used due to its ability to evaporate moisture quickly and maintain a low temperature on particles. In this technique, the formation of microcapsules involves the homogenization of core materials (bioactive compounds) and wall materials (polysaccharides and proteins) to create an emulsion, followed by spraying in the drying chamber (Dhakal & He, 2020). This operation allows the processing of heat-sensitive liquid materials without significantly reducing their quality, and its operation is continuous and economical (Mujumdar, 2014; Ye et al., 2018). Also, the deterioration due to high temperatures in the product is relatively small due to the short times of the procedure (Campelo et al., 2018; Cortés-Rojas et al., 2015).
This review aims to compile up-to-date scientific information on the most relevant technological aspects of microencapsulating bioactive and functional compounds (vitamins, minerals, oily substances, antioxidants, and probiotics) within food matrices using the spray-drying technique, specifically its technological principle, physicochemical properties, the wall materials used, and the operating conditions for the development and innovation of functional foods.
Materials and methods
We searched the ScienceDirect, PubMed, SciELO, and Springer databases using the descriptors: “microencapsulation,” “spray dryer,” “food matrix,” “functional food,” “nutrients,” “wall material,” “physicochemical and functional properties,” “antioxidant,” “vitamins,” and “probiotics.” These keywords were combined differently to obtain search results related to the subject matter.
From the articles obtained, we selected those published between 2010 and 2020, organized according to the topics chosen for each section, and analyzed them to extract information related to the research topic.
Results and discussion
Principle of spray-drying in microencapsulation
In the spray-drying process, the food enters the drying chamber and is sprayed, increasing the surface/volume ratio of the spray droplet and the mass and heat transfer. In turn, the vapor pressure differences of the drops and the hot air cause the drop solvent to be transferred to the gas stream (Poozesh & Bilgili, 2019; Tontul & Topuz, 2017). Inside the drying chamber, a large volume hot air stream as a drying medium comes into contact with another stream of particles (10–500 μm in diameter) with a large surface area through which moisture evaporation occurs. This process is subject to some design characteristics of the dryer, such as the type of nozzle, operating temperatures, flow rate, and applied pressure (Tan et al., 2015; Wei et al., 2019). The air expands adiabatically from the nozzle with small holes to the drying chamber, and its temperature drops almost instantaneously (Morales-Guzmán et al., 2010).
The particles formed during flash evaporation collide with the walls of the drying chamber and pass to a cyclone separator (Lisboa et al., 2018), given the adjustment of the feeding, spraying, and drying capacity to the hot airflow and the drying column height (Paudel et al., 2013; Poozesh & Bilgili, 2019). The primary objective of spray-drying for the microencapsulation of bioactive compounds is to build a barrier between the encapsulated component and the environment to avoid deterioration chemical reactions and contact of the encapsulation with other ingredients (Martínez et al., 2015).
Operating conditions
In considering the thermal characteristics of the materials, this process can obtain compounds with physicochemical and structural properties that preserve the functional qualities of the encapsulated material at low cost, for short processing times, and with a large number of wall materials. These characteristics confer this drying technique numerous advantages for protecting various bioactive compounds (Rodríguez-Restrepo et al., 2017; Solanki et al., 2013).
Air humidity must be well controlled; this is a critical variable as, if there is a very high humidity content in the air, the drying process will take longer to complete. Besides, the microencapsulation must be carried out at low pressures, with different feeding capacities, and low temperature inside the equipment since this could cause the loss of nutrients in the microencapsulated material (Morales-Medina et al., 2016; Rodríguez-Huezo et al., 2014). The diameter of the holes in the nozzle inside the atomizer will determine the size of the particles entering the drying chamber (Morales-Guzmán et al., 2010). The fluid that enters this chamber must be concentrated and have between 30–50% solids for the process to be efficient and fast (Rajabi et al., 2015). At the end of the procedure, a fine powder is obtained whose particle size can be adjusted by varying the operational conditions of the spraying of the previously concentrated fluid (Poozesh & Bilgili, 2019).
Other operating parameters, such as type of nozzle, the capacity of the dryer, flow rate, position of the atomizer in relation to the dispersing air, the mechanism for heating the air, and type of atomizer, are related to the design of the dryer and can be predicted by computational simulation, considering the physicochemical properties of the mixture under operational response variables that will determine the quality of the final product (Wei et al., 2019). Finally, it is necessary to consider that the simultaneous transfer of heat and mass governs the spray-drying process and directly affects its operating conditions (Islam et al., 2016).
Wall materials
In the process of microencapsulating food ingredients by spray-drying, carbohydrates, gums, biopolymers, colloids, waxes, proteins, and starches have been used as wall materials since they have low viscosities, high solid content, and high solubility (Cano-Chauca et al., 2011). The choice of material to use depends on the physical properties of the core (porosity, solubility), the wall (viscosity, glass transition temperature, film-forming capacity), and the compatibility of the core with the wall. Also, the conditions of encapsulation and the end use of the product must be taken into account (Costa et al., 2015). The microcapsules obtained can be made up of one or more layers that contain one or more cores of spherical or irregular shape (Saifullah et al., 2019) (figure 1).
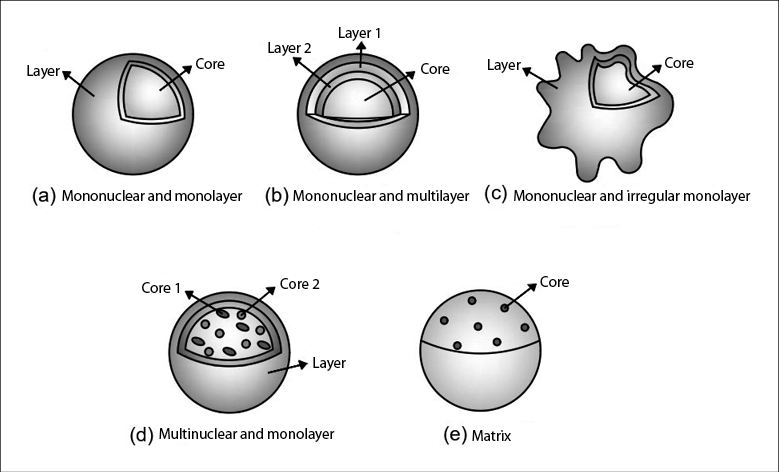
Source: Adapted from Saifullah et al. (2019)
Figure 1 Forms of the microcapsules obtained by spray-drying. a. Mononuclear and monolayer; b. Mononuclear and multilayer; c. Mononuclear and irregular monolayer; d. Multinuclear and monolayer; e. Matrix
Some of the wall materials that have been used in the encapsulation and microencapsulation of bioactive compounds are described in table 1. It also lists some of the results obtained with these materials by different researchers.
Table 1 (cont.) Wall materials used in the encapsulation and microencapsulation of bioactive compounds
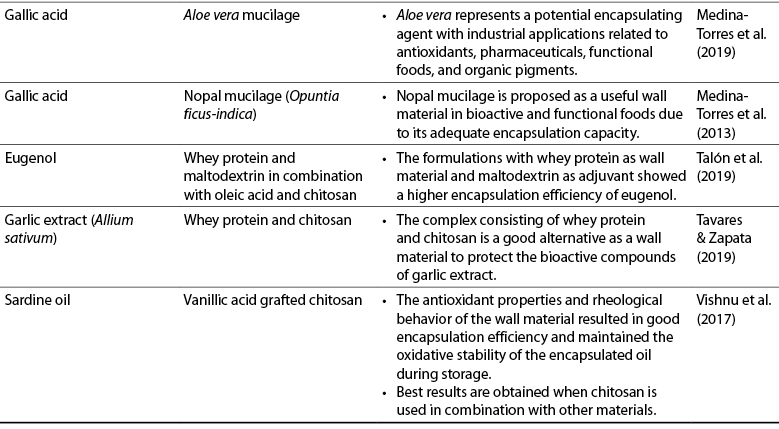
Source: Elaborated by the authors
The requirements of ideal wall material for spray-drying include high solubility, good emulsifying properties, optimal drying qualities, non-hygroscopic character, mild taste, low reactivity, and low cost (Gómez-Aldapa et al., 2019).
Influence of spray-drying on physicochemical and functional properties
These parameters help to understand the structure, distribution, and formation of the particles that make up a food matrix and the changes that can occur in the microencapsulated material. Microencapsulated products using this technique have initial characteristics and properties that depend on the food matrix (Zhang, Khoo et al., 2020).
Particle size
The particle’s size and shape vary in each food and can be affected by the properties of the liquid to be dried and the operating conditions of the process (Chong et al., 2014). The most common methods to determine particle size include the optical ones, based mainly on the scattering of light (Carneiro et al., 2013; Da Silva et al., 2013; Edris et al., 2016; Medina-Torres et al., 2019). In the case of betacyanin pigments extracted from red amaranth (Amaranthus gangeticus), the particle size obtained was 44.44 to 49.44 μm using β-cyclodextrin as encapsulating material and 48.98 to 58.39 μm using maltodextrin (Chong et al., 2014).
For propolis powder with gum arabic in a 1:6 ratio, the average particle size was 24.0 ± 0.6 μm and, in a 1:4 ratio, 23.3 ± 0.4 μm. Using starch with octenyl succinic acid (OSA) as encapsulant in the same proportions (1:6 and 1:4), average particle sizes of 15.0 ± 0.3 μm and 16.0 ± 0.0 μm were obtained, respectively, which were lower than the propolis powder from gum arabic (Da Silva et al., 2013).
Other studies on the preparation of powders from oily substances such as linseed oil and Nigella sativa oleoresin, which had in common the combination of maltodextrin with gum arabic as wall material, showed similar results. For linseed oil, the average particle size was 23.03 ± 0.31 µm, and for Nigella sativa oleoresin, 28.30 µm. The difference between the results was attributed to the drying conditions and the emulsion formulation before the drying process, including the proportions between the encapsulants and the compound to be encapsulated (Carneiro et al., 2013; Edris et al., 2016). According to these results, gum arabic as an encapsulating agent produces a larger particle size. It has also been found that the smaller particles bind to other nearby larger particles and, thus, the particle size obtained becomes even more massive (Islam et al., 2016).
Moisture content and water activity
The inlet humidity of the sample or food dispersion in the drying chamber directly influences the residence time of the sample in the chamber and the final moisture of the product (Daza et al., 2016; Goula & Adamopoulos, 2010). Moisture content can be established using an oven or stove (Goula & Adamopoulos, 2010), moisture analyzers such as conductivity meters, the Karl Fisher method (Daza et al., 2016), or infrared balances (Medina-Torres et al., 2019). It is necessary to reduce their moisture content to preserve the quality of food products, especially those made into powder. If the difference is between the temperatures of the air and the product to be dried is greater, the speed of heat transfer will be higher (Daza et al., 2016; Goula & Adamopoulos, 2010).
The moisture contents of the powdered products obtained by spray-drying vary between 1.50 and 9.55%; a low moisture content prevents agglomeration between particles and improves dust dispersion (Daza et al., 2016; Goula & Adamopoulos, 2010; Medina-Torres et al., 2019). The average moisture content for propolis powder with encapsulating agents of gum arabic and OSA starch in a 1:6 ratio was 9.3 ± 0.4% and 4.9 ± 0.3%, respectively; in a 1: 4 ratio, it was 12.6 ± 0.4% for gum arabic and 7.2 ± 0.6% for OSA starch (Da Silva et al., 2013). In these results, higher moisture content can be observed using gum arabic in different proportions, which can be harmful to the product since the higher the moisture content, the lower the stability.
In another study on drying food-grade solid lipid nanoparticles (SLNs) and nanostructured lipid transporters (NLCs), containing omega-3 fish oil and two different types of maltodextrin (DE 6 and DE 21) as protective materials for lipid particles at different inlet temperatures, Salminen et al. (2019) obtained moisture contents of less than 5.5%. The lowest moisture content range was for NLC particles with maltodextrin DE 21 (2.52-3.20%), and the highest was for SLN with maltodextrin DE 6 (3.89-5.35%) (Salminen et al., 2019).
Another parameter related to moisture content is water activity (aw). For Caliskan and Dirim (2016), the high elimination of water by increasing the drying temperature influences the decrease in aw since a high temperature provides a higher heat transfer rate, generating the driving force for the evaporation of moisture and contributing to the chemical and microbiological stability of powdered food. For their part, Da Silva et al. (2013) reported water activities between 0.25 and 0.39, and Salminen et al. (2019) recorded values in a range of 0.13–0.29. These expected results allow ensuring microbiological stability.
Water activity is closely related to moisture content; this relationship is modeled by sorption isotherms representing the energy required or dissipated during the processes (Da Silva et al., 2013; De Souza et al., 2015). Regarding propolis powder, Da Silva et al. (2013) determined that the gum arabic powders were more hygroscopic than those of OSA starch. Although, when evaluating the stability of the compounds at storage temperatures of 10 and 25 °C, they determined that it was not affected by any of the two encapsulants, and therefore the propolis powder could be stored at room temperature.
Glass transition temperature
This parameter helps to control the adhesiveness, caking, and adverse chemical reactions that may occur in powdered food particles (Medina-Torres et al., 2019) and, therefore, it can predict the stability of dried foods and microcapsules obtained by spraying (Daza et al., 2016; Islam et al., 2016). This property has been used to measure stability in fruit juices and extracts. Goula and Adamopoulos (2010) obtained glass transition temperature (Tg) values within the range of 33–126 °C, which depended on the sample and composition, and observed a positive effect on Tg by decreasing molecular mobility, which improves the stability of powders (Daza et al., 2016; Goula & Adamopoulos, 2010).
In bioactive compounds, it was observed that, with various wall materials, the variation in this thermal property was from 44.98 to 53.35 °C when applying spray-drying, which caused as a positive result a glassy state at temperature environment (Ramakrishnan et al., 2018). According to Caparino et al. (2012), starch can have a Tg of 250 °C, and, in maltodextrin with different degrees of hydrolysis, it can be between 100–188 °C. A high Tg is ideal for powders because it improves stability and promotes more significant conservation (Medina-Torres et al., 2019; Ramakrishnan et al., 2018).
Solubility
It is the speed with which dust particles reach dissolution in water, disperse, and become wholly humidified (Cortés-Rojas et al., 2015; Daza et al., 2016). Better aqueous solubility and bioavailability of compounds of interest have been observed through complex formation with vehicles such as whey protein isolate for curcumin (Liu et al., 2016). Likewise, in the extracts of cagaita fruit (Eugenia dysenterica), gum arabic, and inulin in any concentration increased solubility, gum arabic (94.4-97.8%) more than inulin (86.8-95.9%) (Daza et al., 2016). The solubility of a powder is very important for its reconstitution and the availability of encapsulated compounds; generally, commercial powdered products have a solubility greater than 90% (Cortés-Rojas et al., 2015; Daza et al., 2016).
Viscosity
The viscosity of spray-dried foods varies with changes in temperature, concentration, and shear rate (Badui, 2013). In a study on synthetic strawberry flavor microencapsulation, when the concentration of the encapsulating agent increased, the viscosity increased as follows: from 0.04 to 0.29 Pa·s with modified starch, from 0.04 to 0.92 Pa·s with gum arabic, and from 0.04 to 0.64 Pa·s with xanthan gum (Pellicer et al., 2018).
In Nigella sativa oleoresin encapsulated using gum arabic and maltodextrin, the viscosity of the emulsion was 0.76 Pa·s before the drying process. The high viscosity of the solution created large droplets upon drying when using a two-fluid nozzle composed of an inner tip with a 0.7 mm diameter opening and an outer ring with a 1.5 mm diameter opening (Edris et al., 2016).
Moreover, in the encapsulation of caffeine with egg albumin as an encapsulating vehicle, Tan et al. (2020) found that from 56 °C there was an exponential increase in viscosity attributed to the denaturation of the protein as an effect of temperature. The inlet air temperature for spray-drying in those studies ranged between 60 and 200 °C and the outlet temperature between 23 and 101 °C (Edris et al., 2016; Pellicer et al., 2018; Tan et al., 2020).
Color
This parameter is a physical attribute affected by high drying temperatures (Caparino et al., 2012). Color has been measured in most studies with the help of the CIELAB color system, also known as L∗a∗b∗. L* indicates luminosity, a* indicates negative green and positive red, and b* represents negative blue and positive yellow. This system also allows calculating other coordinates such as chroma or color purity (C*), hue or hue angle (H°), and color change (∆E) (Caparino et al., 2012). From now on, luminosity will be considered as a variable to establish whether the spray-dried samples lose or gain luminosity due to the effect of heat treatment with inlet temperatures ranging between 120 and 180 °C and outlet temperatures between 70 and 90 °C (Caparino et al., 2012; Daza et al., 2016; Ramakrishnan et al., 2018; Rezende et al., 2018).
In a study on bioactive compounds obtained from acerola pulp and residues, Rezende et al. (2018) showed a color difference (darker) in the luminosity values (L*) as a result of the spray-drying process. In bioactive compounds of tamarind juice (Tamarindus indica) analyzed by Ramakrishnan et al. (2018), the increase in the L* value after adding the wall material decreased the intensity of the color in the final powder product. Similarly, in a study carried out on extracts of cagaita fruit, Daza et al. (2016) found that the L* value increased due to the influence of the encapsulating agent and the air inlet temperature on the powder product obtained at the end of the process.
Increased luminosity is a positive consequence of the spray-drying process, as it affects the perception of the product quality to the naked eye. Therefore, this property is relevant when choosing operating conditions and encapsulating agents during microencapsulation (Caparino et al., 2012).
Quality problems in microcapsules
The microcapsules obtained may experience an unwanted physical phenomenon known as agglomeration during the processing, storage, and transport of powdered products, which must be fine, fluid, and easy to handle (Haider et al., 2018). Excessive agglomeration and melting of dust particles result in the formation of lumps that dirty and block equipment, which, in turn, increases production steps, can force the use of anti-caking agents, and generate additional costs. For reducing product waste, these drawbacks can be avoided by controlling individual powder interactions and the conditions governing them (Haider et al., 2018).
Release of microcapsules during consumption
The release of the compounds can occur through the following mechanisms: diffusion, in which the compounds move from the interior of the matrix towards the surface until they are free (Parra, 2010); dissolution, when the wall material dissolves because of the influence of external factors (Parra, 2010; Saifullah et al., 2019); fracture, in which the encapsulant breaks due to external or internal forces (Parra, 2010); swelling, owing to the absorption of the surrounding fluid, and erosion, in which the encapsulating material becomes thin and gradually degrades (Saifullah et al., 2019). Controlled release is a method that defines the availability and the times in which the encapsulated compound will be released at a specific rate (Carlan et al., 2017).
In studies on the microencapsulation of vitamin C, release rates of 95% in 30 minutes have been found using a cross-linking agent such as chitosan (Carlan et al., 2017). In the case of vitamin B12, 100% was released in 120 minutes with chitosan; however, when using alginate, the release time of this vitamin was 15 minutes and with modified chitosan 10 minutes. Reportedly, the 100% release rate of vitamin C was similar to that of vitamin B12 (Estevinho et al., 2016; Murugesan & Orsat, 2011).
Microencapsulation of vitamins
Vitamins are considered sensitive to different types of processing in the industry, which dramatically affects their stability and is mainly reflected in properties such as color and taste (Hategekimana et al., 2015). Studies on the variables that influence the behavior, degradation, and stability of vitamins have shown that encapsulation provides the best conditions to maintain their stability (Murugesan & Orsat, 2011; Parra, 2010).
Hategekimana et al. (2015) have studied the microencapsulation by spray-drying of vitamins C, A, D, E, and some of the B complex. For vitamin B12, with an inlet temperature of 120 ºC, an outlet temperature between 53 and 58 ºC, and a standard 0.5 mm nozzle as drying conditions, Carlan et al. (2017) reported good stability results using modified chitosan as wall material. Microencapsulation considerably improves the stability of vitamins and protects them from oxidation, light, temperature, humidity, and other factors during storage time so that their quality can be optimal for several months with a slight decrease in concentration (Carlan et al., 2017).
Microencapsulation of minerals
Minerals can react to oxygen through rapid release, chemical degradation, protein aggregation, and unpleasant taste sensations (magnesium and iron). Besides, they may experience reactions during storage that compromise their bioavailability (antiminerals or chelators) and the stability of the foods containing them (Salminen et al., 2019; Ye et al., 2018). Wang et al. (2016) applied encapsulation by spray-drying to develop functional instant beverage powder formulations. The pectin-casein mixture as wall material and the addition of peppermint oil as a potential antimicrobial agent allowed the simultaneous encapsulation of hydrophilic and hydrophobic vitamins with a pH of 4.5, prolonged the useful life of mineral salts during refrigerated storage at room temperature for 15 days, and preserved the antioxidant activity of the encapsulated nutrients.
Spray-drying in oily substances
One of the main problems associated with oils rich in polyunsaturated fatty acids is their high tendency to oxidative deterioration and its consequent production of undesirable flavors (Carneiro et al., 2013). Given the need to protect these oils to make them more stable during handling, processing, and storage, spray-drying has been shown to protect oil droplets in an emulsion from lipid oxidation, help control the release of encapsulated active ingredients, and improves its stability in storage (Hashib et al., 2015).
The selection of the wall material influences the stability of the emulsion during its formation and after the drying process; besides, its concentration can affect the characteristics of the resulting microcapsules (Martínez et al., 2015). When added in excess, polysaccharides or high molecular weight surfactants can remain free in the aqueous phase and give rise to long-term destabilization mechanisms such as flocculation and coalescence of oil droplets (Carneiro et al., 2013; Hernández et al., 2015).
The wall material used in the microencapsulation of fatty acids by spray-drying must be highly soluble in water, have good emulsifying properties (Campelo et al., 2018), a neutral aroma, and low viscosity at high solid concentrations. Also, it must provide adequate protection from oxidation, be inexpensive and non-toxic, and have good microcapsule production performance (Fernandes et al., 2016).
In addition to preserving the nutritional properties of oils and improving their stability to physicochemical changes (Luna-Guevara et al., 2017; Yingngam et al., 2019), microencapsulation by spray-drying allows obtaining functional foods from the addition of encapsulated oils to any type of food to improve its nutritional quality and increase the content of essential fatty acids and antioxidants (Martínez et al., 2015).
Microencapsulation of antioxidants
Fruits provide significant amounts of water, fiber, and a wide variety of vitamins and minerals, have a low-fat content and are an almost exclusive source of antioxidants (Santos et al., 2019). Although the drying of fruits increases the stability of the product and improves its handling, it can produce nutraceutical losses due to the temperatures used in the process, which can range between 140 and 180 °C in the inlet air and between 60 and 110 °C in the outlet air (Fang & Bhandari, 2011; Rezende et al., 2018; Santos et al., 2019).
Different studies have reported the successful microencapsulation of bioactive compounds, such as those obtained from pulp and acerola (Malpighia emarginata) residues, in which spray-drying showed better retention of concentrations of bioactive compounds compared to freeze-drying (Rezende et al., 2018). Likewise, in anthocyanins, phenolic compounds, and antioxidant activity in blackberries (Rubus ulmifolius), encapsulation reduced the degradation of antioxidants due to changes in pH (Santos et al., 2019).
Microencapsulation of probiotics
Most of the probiotic foods on the market today are refrigerated dairy products. However, the sale of non-dairy probiotics such as baked goods, meat products, fruit- and legume-based products, mayonnaise, and chocolate-based products has recently increased as an alternative to reduce the risks related to lactose intolerance, allergies produced by dairy proteins, and the consumption of saturated fat and cholesterol (Rouf et al., 2018).
It has been ascertained that probiotics should survive in a significant number of 106 to 108 CFU/g, although the number varies from one probiotic strain to another (Martín et al., 2015). The growth and survival of probiotics are determined by the properties of food (water availability, pH, buffer capacity, among others) and storage conditions (temperature, relative humidity, and atmosphere) (Tripathi & Giri, 2014). Microencapsulation is the ideal mechanism to improve the viability of probiotics in some food matrices; in recent years, commercial food products containing encapsulated probiotic cells have been introduced (Tripathi & Giri, 2014).
Some researchers have reported viability values of encapsulated microorganisms by spray-drying within the range of 107 to 1010 CFU/g, including Lactobacillus paracasei (Cassanego et al., 2015), Lactobacillus acidophilus (Yonekura et al., 2014), and a probiotic microbial triple of Bacillus polymyxa, Bacillus megaterium, and Lactobacillus delbrueckii subsp. bulgaricus (De Araújo-Uribe et al., 2018).
In a recent study, Huang et al. (2017) carried out spray-drying on a pilot scale for microencapsulating L. casei and P. freudenreichii and obtained 100% survival, regardless of the probiotic strain used, operating at lower temperatures with a hyper-concentrated serum of inorganic calcium salts (Ca2+) as wall material. These conditions caused increased tolerance to heat, acid, and the bile salts of the microcapsules obtained.
Conclusions
Numerous studies have been conducted on the application of spray-drying for microencapsulating bioactive compounds. This unit operation is very versatile and has a variety of applications, including the prolongation of the useful life of food raw materials by analyzing absorption isotherms, which relate equilibrium moisture content and water activity.
The wall material significantly impacts different encapsulation characteristics such as stability, solubility, particle size, sensory properties (color and flavor), and antioxidant power.
The microencapsulation by spray-drying contributes significantly to fortifying and enriching food by incorporating vitamins and minerals of different chemical nature. Moreover, this technology allows the controlled release of the bioactive component and the organism to increase its bioavailability. The encapsulation of probiotics by spray-drying is being applied successfully to non-dairy foods, thus developing new functional feeding options.
There is a growing trend to replace traditional encapsulating materials with new, widely available, low-cost compounds of natural origin. This trend can boost the variety and supply of raw materials in the encapsulant market by including agribusiness byproducts. The byproducts can also be used as biofunctional ingredients capable of counteracting chronic and highly prevalent diseases in the global population.