Introduction
The onset of lactation represents a transition period with physiological and metabolic changes that disturb homeostatic balance and reproduction . This process begins in the final stage of gestation and continues during the initial period of lactation, where the growth of the pregnant uterus negatively influences rumen size and dry matter intake 1. This change continues after calving, coinciding with an increase in nutritional requirements for milk synthesis, and leading to a negative energy balance (EB) of the matrices 2.
As reproduction is a characteristic of low heritability, reproductive performance is influenced by non-genetic factors such as environment and nutrition 3, the latter being an important relation with the prepartum metabolic state and postpartum fertility 4. Thus, EB becomes a determinant condition for the resumption of ovulation and involution of the uterus. EB have been estimate by the plasma concentration of blood metabolites such as NEFA, βHBA and glucose and even by the body condition score.
NEFA are free fatty acids products of the degradation of lipids stored as fat, βHBA are ketone bodies produced mainly in the liver, from the free volatile fatty acids (aceto acetate), butyrate and aceto acetate present in the portal circulation. Ruminal epithelium also produces small amounts of βHBA from acetate and butyrate. Thus, these metabolites represent negative states of energy balance. The glucose, on the other hand, is a metabolite of synthesis, mainly produced in the liver from propionate, but also produced by the intestinal epithelium normally when animals were have been fed grain without heat treatment, where part of the starch escapes ruminal degradation 5.
Hypothalamic nuclei is responsible for the ovulation, responding with release of GnRH pulses when the animal is in positive energy balance (BE), because GnRH stimulates the release of FSH and LH by the pituitary hormones responsible for growth and ovulation of the follicle 6.
During the postpartum period, the involution of the uterus, with the resumption of ovulation, become essential to achieve intervals between ideal deliveries, so the earlier matrix starts the postpartum uterine cyclicity and involution, the greater will be the fertility achieved during the breeding period 7.
The pregnancy cows, which graze in the dry season during the last gestation period, have been related to weight loss, loss of body condition score and high concentration of NEFA and βHBA, leading to long periods of negative energy balance, this, adequate prepartum nutrition through supplementation, improved nutrient intake, and positive energy balance return 8. Feeding and other care with the matrices in the three weeks before and three weeks after childbirth become the success of the reproductive process; however, nutrition in the early stages of gestation is the most important phase for fetal development and offspring performance 9-13.
Therefore, this experiment could be determine the effect of supplementation in the early gestation periods on the prepartum energy balance and its relation to the ovulation period uterine involution and fertility.
Materials and methods
Location and weather conditions
This experiment was approved by the Brazilian Ethics Committee on Animal Use (CEUAP/UFV - Process nº 26/2014), according to ethical principles of animal experimentation established by the National Council of Animal Experimentation Control (CONCEA). The experiment was performed at the Beef Cattle Farm of Animal Science Department of University Federal de Viçosa, Viçosa-MG, Brazil. Weather conditions during the experiment were: 284 mm of rain and an average temperature of 22.6 °C (first trimester of pregnancy); 94 mm of rain and average temperature 18.6 °C (second trimester of pregnancy); 61 mm of rain and average temperature 18.7 °C (last trimester of pregnancy).
Animals, experimental design and supplements
In the experiment, twenty-seven pregnant Nellore cows were using with a 490 ± 12.82 kg average initial weights. Cows were artificially inseminated at a fixed time using semen from the same bull. At sixty days of gestation, fetal sexing it was perform to obtain homogeneous treatments. Animals were allocated to six-hectare paddocks per treatment, evenly covered with Urochloa decumbens grass, equipped with a drinkers and feeders. Experimental design was a completely randomized design, with three treatments and nine replications as following: UNS, unsupplemented during gestation; MID, supplemented from the 30 to 180-day of gestation; LATE, supplemented from the 181 to 281-day of gestation. Composition of supplements and pastures are shown in Table 1. Percentage composition of supplements was the: ground corn grain (26.25%), wheat meal (26.25%) and soybean meal (47.5%). A total of 150 kg of supplement per animal were provided during the total experiment period for each treatment (1 and 1.5 kg/day to cows in the MID and LATE groups respectively), accompanied by a mineral mixture offered ad libitum (Table 1). The cows in the UNS group, received only a mineral mixture ad libitum during gestation.
Table 1 Nutrient content of dietary supplements and pastures offered to cows during gestation. NDFap, neutral detergent fibre correct for ash and protein; i-NDF, indigestible neutral detergent fibre; Mineral mix: CaHPO4, 50.00%; NaCl, 47.15%; ZnSO4, 1.50%; Cu2SO4, 0.75%; CoSO4, 0.05%; KIO3, 0.05% and MnSO4, 0.05%.
Item | Supplement | PastureA | PastureB |
---|---|---|---|
Dry matterC | 879.85 | 877.20 | 886.74 |
Matter organicD | 958.72 | 907.36 | 906.95 |
Crude proteinD | 282.70 | 92.46 | 74.84 |
Ether extractD | 34.64 | 49.51 | 41.75 |
NDFapD | 196.26 | 565.70 | 587.00 |
Non-fibrous carbohydratesD | 445.10 | 199.68 | 203.35 |
i-NDFD | 35.50 | 207.48 | 191.81 |
A Manual grazing simulation, early-mid gestation.
B Manual grazing simulation, late gestation.
C g/kg fresh matter
D g/kg dry matter
Experimental procedures and sampling
Cows were weighed after 14 hours of fasting at the beginning (30 days of gestation) and end of their portion of the experiment. In order to minimize possible paddock effects on the treatments, all animals were rotated every seven days; all groups stayed the same period in each paddock. Pastures samples were collected via manual grazing simulation, every 15 days. Samples were dried in an forced-air circulation oven (SOLAB®, Ar SL - 102) at 55-60ºC for 72 hours and were crushed in an 1 and 2 mm knife mill (Willye® TE-680).
To evaluate the nutritional characteristics of diet, a digestibility trial was performed for nine days at both 100 and 230 days of gestation. The three-marker method was used as follows. Chromic oxide (Cr2O3) was used estimate the faecal excretion of animals, which was wrapped in paper cartridges in an amount of 20 g per animal/ day and administered with a metal probe via the oesophagus at 10 hours 14. Titanium dioxide (TiO2) was used to estimate the individual supplement intake, provided via supplement at the proportion of 10 g/kg of supplement 15. Indigestible neutral detergent fibre (iNDF) was used to estimate the pasture dry matter intake 14. The first five days of each trial were used for animal adaptation to TiO2 and Cr2O3. Faecal samples were collected immediately after of defecation or directly from the rectum of animals, in the last 4 days of experiment (one sample for day) at the times 18, 14, 10 and 06 hours. After collection, faeces were dried in a forced-air circulation oven (SOLAB®, Ar SL - 102) at 60 °C for 72 hours and were crushed in an 1 and 2 mm knife mill (Willye® TE-680). A composite sample was prepared for each animal and stored in glass container for subsequent analysis.
Spot urine samples were obtained on the last day of the digestibility trial by spontaneous urination and blood samples collected via jugular venipuncture, 4 h after supplementation. Blood samples were collected using vacuum tubes containing a clot activator and separator gel (BD Vacuntainer®, SST II Advance, Cidade Industrial, Curitiba - Paraná, Brazil). After collection, the samples of urine were diluted in H2SO4 (0.036 N) and frozen at -20°C 16 for later evaluation of the contents of creatinine, urea and purine derivatives. The blood was immediately centrifuged at 2700 RPM for 15 min at room temperature, and the serum was stored at -20°C, for later evaluation of the contents of urea.
Chemical analysis
The dry matter content (DM), crude protein (CP), ether extract (EE), neutral detergent fiber corrected to ash and protein (NDFap) and iNDF were quantified in supplement, pasture and fecal samples processed as mentioned above. DM, CP and EE were processed according to AOAC 17; NDFap, according to 18, using thermostable a-amylase, without using sodium sulphite, with ash corrections and protein; iNDF, according to 19, obtained after in situ incubation in F57 Ankom® bags (Macedon, NY, USA) for 288 h. In the fecal samples, chrome was evaluated by atomic absorption spectrophotometry 20, and titanium by colorimetric determination 15.
Consumption of total digestible nutrients (NDT) was determined by the sum of the consumption of each of the nutritional components of the diet multiplied by the digestibility of each, as seen in the following formula. The digestibility of each nutritional component can be observed in the first part of this work 11:
Intake TDN (kg/day) = ((I-CP*%DCP) + ((I-EE*%DEE)*2.25) + (I-NDF*%DNDF) + (I-NFC*%DNFC))
Where: I-CP and DCP is intake and digestibility of crude protein; I-EE and DEE is intake and digestibility of ethereal extract; I-NDF and DNDF is intake and digestibility of neutral detergent fiber; I-NFC and DNFC is intake and digestibility of non-fibrous carbohydrates.
Fecal dry matter excretion was estimated based on the amount of indicator ingested and its concentration in the feces 21:
Fecal DM (kg/day) = AOI / ICF,
Where: AOI is the amount of indicator ingested (g); ICF is the indicator concentration in feces (g/kg of feces).
Estimates of individual supplement intake were obtained as follows:
ISI = [(FE x ICaF) / IOG] x SOG,
Where: ISI is the individual supplement intake (kg/day); FE is fecal excretion (kg/ day); ICaF is the indicator concentration in animal feces (kg/kg); IOG is the indicator present in the supplement offered to each group (kg/day); and SOG is the supplement amount offered to the group of animals or treatment (kg/day).
Estimation of voluntary DM intake of forage (DMIF) was performed using iNDF as the internal marker 22, as follows:
DMIF (kg/day) = (FE x FIC) / FOIC,
Where: FE is fecal excretion (kg/day); FIC is the indicator concentration in the feces (kg/kg); FOIC is the indicator concentration in forage (kg/kg).
Total dry matter intake was obtained by the sum of cows concentrate and forage consumption.
Creatinine, uric acid and urea were quantified by the kinetic colorimetric, enzymatic colorimetric and fixed-time kinetic methods, respectively, using an automatic device for biochemistry (Mindray® model BS200E) and kits. The daily urinary volume was calculated by employing the ratio between the day excretion of creatinine (ECU), adopting the equation proposed by 23 and its concentration in the spot samples as reference:
ECU (g/day) = 0.0345 x BW0.9491
Where: BW is body weight.
Allantoin in the urine was analyzed as described by Chen and Gomes 24. The total excretion of purine derivatives was calculated as the sum of the amounts of allantoin and uric acid excreted in the urine. Absorbed purines (Y, mmol/day) were calculated from the excretion of purine derivatives (X, mol/day), by the following equation proposed by 25:
Y = (X - 0.301 x BW0.75)/ 0.80
Where: 0.80 is the recovery of absorbed purines as purine derivatives; 0.301xBW0.75 is the endogenous contribution to the excretion of purines.
The ruminal synthesis of nitrogen compounds (Y, g micN/day) was calculated as a function of the absorbed purines (X, mmol/day), using the equation described by 25.
Y = 70X / 0.93 x 0.137 x 1000
Where: 70 is the N content in the purines (mgN/mol); 0.137 is the purine N:total bacterial N ratio; and 0.93 is the digestibility of the bacterial purines.
Microbial efficiency was expressed as g microbial CP/kg of total digested organic matter (g micCP/kg DOM).
Metabolites of energy status
Blood samples were obtained via puncture of the jugular vein, for analysis of glucose, NEFA and βHBA on days 30, 105, 180, 230 and 280 of gestation. After collection, the blood was immediately centrifuged at 3600 rpm × 20 minutes, the serum stored (-20°C) for further analysis.
The method for quantification of NEFA and βHBA was the colorimetric methods, using a Bioclin® kit and Randox® kits respectively, using the automatic equipment for biochemistry of Mindray brand, model-BS200E. Glucose levels were quantified by enzymatic colorimetric method using commercial kits of the brand Bioclin®. In these analyzes we used the automatic equipment for biochemistry of the brand Mindray, model-BS200E.
The different procedures were carried out according to the protocols recommended by the companies supplying the respective kits.
Evaluation of carcass traits by ultrasonography
At the end of each supplementation period, carcass traits of the cows were evaluated by ultrasound (Aloka, model: SSD 500 v, with linear probe of 18 cm). Images of the carcass were obtained from the right side of the animal where images were taken to measure the thickness of the subcutaneous fat, from an average of two measurements, the first between the thoracic vertebrae T12 and T13 and the second one in the region pelvic between the ischium and the pubis. Images were analyzed in BioSoft Toolbox® II for meat software (Biotronics Inc., Ames, Iowa, USA).
Postpartum reproductive assessments
Using transrectal ultrasonography (Aloka 500 with a 5 MHz linear probe, Aloka, Wallingford, CT) were performed on days 10, 18 and 30 postpartum evaluations to determine uterine involution and return of cyclic luteal activity. After delivery, the non-pregnant horn was measured and considered uterine involution when the horn that supports the gestation is measured close to that of the non-pregnant horn. The measurements were longitudinally and vertically over the curvature of the horn. Presence of fluid and position of the uterus (abdominal or pelvic) were evaluated to determine complete uterine involution.
Forty-five days postpartum, the matrices were protocolized and inseminated by IATF, the protocol used was as follows: Day 0: application of intra-vaginal slow-release progesterone device plus 2 ml of estrogen (estradiol benzoate); Day 7: withdrawal of the intra-vaginal slow-release progesterone device plus application of 2 ml prostaglandin F2α (Cloprostenol); Days 8: application of 0.5 ml of estrogen (estradiol cypionate); Day 10: 48 hours after application of estrogen was performed artificial insemination in all matrices; Day 31: the intra-vaginal slow-release progesterone device was reapplied in all matrices; Day 38: withdrawal of intra-vaginal device from slow release of pro gesterone in all matrices. Pregnant cows were sent to the batch of pregnant women and counted as a positive result of the first IATF, empty cows continued the protocol with application of 2 ml of prostaglandin F2α (cloprostenol); Day 39: application of 0.5 ml of estrogen (estradiol cypionate) in the empty matrices; Day 41: forty-eight hours after application of estrogen was performed artificial insemination in all matrices. Twenty-eight days after the second insemination the diagnosis of gestation was made by ultrasonography and the percentage of total gestation was determined (Figure 1).
Statistical analyzes
The PROC MIXED of the SAS (Statistical Analysis System, version 9.0) software was used for all statistical analyses. For all statistical procedures we adopted α=0.10 of probability for type I error, due the high incidence of type II error in this kind of study. Means were submitted to analysis of variance and regression, comparison different periods of supplementation, being UNS, MID, LATE = unsupplemented during gestation, supplemented from the 30 to 180-day of gestation and supplemented from the 181 to 281-day of gestation. Sex of the fetus was tested as fixed effect and removed from the model while not significant. Initial BW was used as a co-variable when significant and removed from the model while not significant. The variables on the effect of different supplementation (treatments) on the measured parameters were analyzed in a completely randomized design. Posture of the uterus and presence of fluid in the uterus were evaluated by generalized models.
Result
Intake and Performance
During the period between 30-180 days of gestation, MID matrices presented higher consumption of TDN (P =0.002) and CP (P =0.001) compared to UNS and LATE. Greater consumption of MS (P =0.001), PDM (P =0.041), TDN (P 0.001) and CP (P =0.001) were observed for LATE matrices during 181-281 in comparison with UNS and MID (Table 2).
As observed in Table 3, there was no difference in live weight and subcutaneous fat thickness of the matrices during gestation (P> 0.10).
Table 2 Dry matter and nutrient intake of dietary treatments offered to cows during gestation. UNS, cow unsupplemented during the gestation; MID, cows supplemented during 30 to 180-day of gestation; LATE, cows supplemented during 181 to 281-day of gestation; PDM, Pasture dry matter; TDN,Total digestible nutrients.
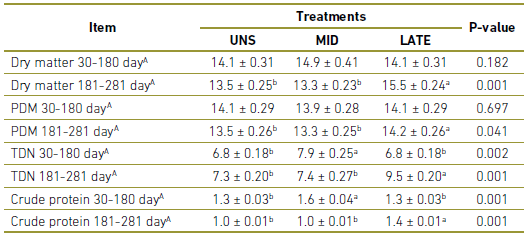
A In kg.
a,b Values within a row with different superscripts differ significantly at P<0.05.
Table 3 Performance of cows during gestation. UNS, cow unsupplemented during the gestation; MID, cows supplemented during 30 to 180-day of gestation; LATE, cows supplemented during 181 to 281-day of gestation.
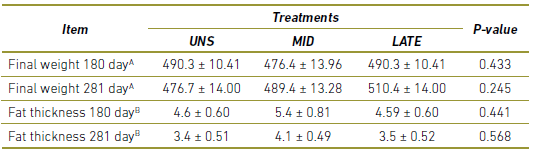
A In kg
B In mm.
a,b Values within a row with different superscripts differ significantly at P<0.05.
Energy status and nitrogen balance
A lower concentration of NEFA and BHBA (P <0.10) at 180 and 281 days of gestation was observed for MID and LATE matrices respectively (Figure 2A-B). Higher glucose concentration during pregnancy was observed for MID matrixes at 180 days (P =0.088) and LATE at 281 days (P =0.094) of gestation. For the rest of the days evaluated, no statistically significant difference (P> 0.10) was observed for the concentrations of NEFA, BHBA and glucose (Figure 2C).
As shown in Figure 3, higher microbial efficiency was detected for UNS and MID matrices during 30-180 days of gestation (P =0.033). During this same period, MID matrices presented higher concentration of SUN (P =0.001) and UUN (P =0.001) compared to UNS and LATE, meanwhile, no differences were observe between the treatments for MICN (P = 0.215). Greater MICN (P =0.001) and UUN (P =0.001) for LATE matrices were observed between 181-281 days of gestation when compared to UNS and MID, however, there was a higher concentration of UUN for LATE compared to MID.
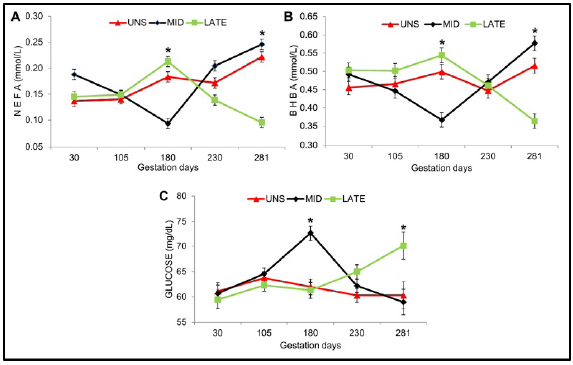
Figure 2 Pre-partum energetic status of females during gestation. UNS, unsupplemented during gestation; MID, supplemented from the 30 to 180 day of gestation and LATE, supplemented from the 181 to 281 day of gestation. (A) NEFA: fatty acids not esterified, (B) BHBA: beta hydroxybutyrate and (C) Glucose during gestation. Bars represent means ± s.e (n = 9). Asterisks indicate significant differences between the groups (*P<0.05).
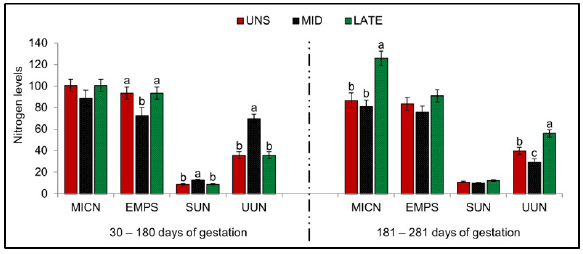
Figure 3 Microbial efficiency and nitrogen balance. UNS, unsupplemented during gestation; MID, supplemented from the 30 to 180-day of gestation; LATE, supplemented from the 181 to 281-day of gestation. MICN, microbial nitrogen (gr/day); EMPS, microbial efficiency (gr PB/Kg digestible organic matter - DOM); SUN, blood urea nitrogen (mg/dl); UNN, urea nitrogen in urine (gr/day). Bars represent means ± s.e (n = 9). Letters indicate significant differences between the groups (P <0.05).
Postpartum Reproductive Parameters
There was no significant difference between treatments for uterine involution at 10, 18 and 30 days postpartum (Figure 4A). During the postpartum evaluation period, there was no presence of ovarian corpora luteal. Uterine involution was observed at 30 days of gestation with a size of 14.40 ± 1.31, 15.70 ± 1.20 and 14.70 ± 1.25 mm, respectively for the UNS, MID and LATE treatments.
UNS, MID and LATE treatments presented 4/9, 6/9 and 7/9 pregnant matrices respectively during the first artificial insemination, without statistical difference in the gestation percentage between the treatments for the first and second artificial insemination (Figure 4B).
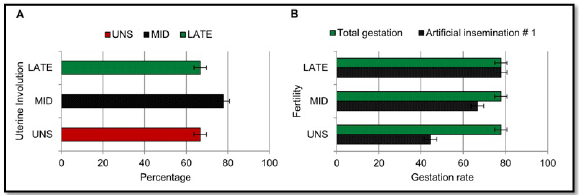
Figure 4 Postpartum reproductive parameters of cows supplemented during pregnancy. UNS, unsupplemented during gestation; MID, supplemented from the 30 to 180-day of gestation; LATE, supplemented from the 181 to 281-day of gestation. Bars represent means ± s.e (n = 9). Asterisks indicate significant differences between the groups (*P<0.05).
Discussion
Increased lipomobilization during the last four weeks of gestation was not associated with delay in first ovulation and altered fertility in multiparous cows 26. Similar data was observed in the present experiment, where, although no difference was observed between treatments in relation to Fat thickness, higher concentration of NEFA and BHBA at 281 days for MID did not affect uterine involution and fertility. Increased concentrations of NEFA and BHBA in MID matrices at 281 days of gestation can be explained by the fact that pregnant cows metabolize energy reserves and alter their metabolism to meet the energetic demands of the growing fetus during the last gestation period 27.
It was expected that MID matrices when supplemented between 30 and 180 days of gestation will present an increase in microbial nitrogen and in microbial efficiency; this absence of the statistical difference in the concentration of microbial nitrogen between the treatments and lower microbial efficiency for MID matrices when compared with UNS and LATE during 30-180 days of gestation, can be explained by the ability of cattle to become more efficient in the presence of nutritional restrictions, because although matrices UNS and LATE have a lower concentration of blood urea nitrogen, in search of an adequate nitrogen balance, they reduce the glomerular filtration of nitrogen, thus presenting lower urea nitrogen in the urine and thus increasing the concentrations of systemic urea nitrogen available for return to the rumen through the ruminal walls or by means of saliva; greater contribution of urea nitrogen to the ruminal environment increases the population of ruminal bacteria, provided there is presence of available energy sources 5, such as the case of the present work where the animals were kept in pasture. Similar data were found in the work done by 28, and it can be observed that non-supplemented animals became more efficient in the nitrogen balance, due to the lower concentration of blood urea nitrogen, reduced nitrogen elimination through urine and increased ruminal microbial efficiency.
This same situation of metabolic efficiency can be observed in MID matrixes between 180-281 days of gestation, where despite not receiving supplementation in this experimental period, the concentration of urea nitrogen in urine decreased (decrease in glomerular filtration), maintaining the concentration of blood urea nitrogen and microbial efficiency.
From the above, the absence of statistical difference for weight gain and final weight between supplemented and non-supplemented matrices is explained, since the increase in microbial efficiency improves the digestion of the fibrous carbohydrates present in the forage, with a positive effect on the consumption by decreasing the effect of ruminal repletion (NDF) and greater contribution of volatile fatty acids available for maintenance and animal production.
The best energy status presented by the MID and LATE matrices at 180 and 281 days of gestation respectively, due to the higher glucose concentration and the lower concentration of NEFA and BHBA, can be explained by the effect of the supplementation received during the specific periods of supplementation, which generates a greater consumption of DM (for LATE), TDN and CP (for MID and LATE) during the respective periods of supplementation during pregnancy. Based on the data of the present investigation and those reported by (28, 29), we can affirm that the energetic protein supplementation regardless of the productive phase in which the animals are found, improves the consumption of the nutritional components of the diet, favoring the energetic, protein balance and productive performance.
Increased thickness of subcutaneous fat at delivery has been associated with a high probability of postpartum gestation 4. Although supplemented animals presented a balanced energy balance when compared to non-supplemented, no difference in subcutaneous fat thickness and body condition score was observed at 180 and 281 days of gestation, and may explain, in a way, the equality in gestation percentage between the treatments.
Even if no statistical difference was observed in relation to the percentage of gestation, I should note that in order to reach the same percentage of total gestation, matrices not supplemented during gestation would spend higher numbers of semen doses when compared with supplemented matrices, in addition of to represent an important economic contribution for the producer for the sale of milk during the lactation months and the sale of the progeny.
Upon observing Figure 4B, a greater number of LATE matrices compared to MID matrices presented a graded uterus at the first insemination. It could be explained by the prepartum metabolic condition (Figure 2) in which they were. LATE matrices thanks to supplementation change metabolic states from negative to positive energy balance, contrary to what happened in MID matrices, moving from positive to negative energetic states, needing more postpartum time (Second insemination) to equalize the number of pregnant matrices.
Conclusion
Supplementation during gestation improves energy balance with decreased levels of NEFA, BHBA and increased glucose levels, with no change in uterine involution and postpartum fertility. Pregnant matrices with a certain degree of nutritional restriction have the capacity to become more efficient, improving nitrogen balance, maintaining microbial efficiency and animal performance. Supplementation during pregnancy does not alter pre-delivery subcutaneous fat thickness or postpartum fertility.