INTRODUCTION
Passionfruit crops have contributed to regional development in different countries such as Colombia, Ecuador and Brazil; the availability of moisture is a constraint on development because of low rainfall in cultivable land; thus, soil salt concentration is increased. In most cultivated plants, sensitivity to salinity and drought conditions are associated with the inability to prevent and/or tolerate high concentrations of salts. In light of this, the capacity for salt exclusion is an important mechanism of tolerance (Moriuchi et al., 2016).
Productivity and development of plants of the genus Passiflora are affected by solar radiation, temperature, sunshine and soil moisture loss. Factors such as water stress and extreme conditions of salinity (≥2.5 dS m-1) limit the productive potential of passion fruits (Dias et al., 2012). Therefore, to achieve good yield and good quality of fruits when environmental conditions are not favorable, it is imperative that we understand how these plants adapt to extreme conditions of salinity and hydric conditions to start breeding programs for improving their adaptability to abiotic stress. As of date, there is little information on the physiological mechanisms that determine differential behaviors in Passiflora. Strategies for osmotic adjustment in halophytes and glycophytes are expensive in terms of physiological maintenance, determined by the efficiency in the synthesis of organic compounds, transport and ion repair, changing structures and cellular components (Saied et al., 2010). In general, halophilic plants have a greater ability to accumulate ions in cells in the aerial part of the plant, as compared to glycophytes; however, there is little information on the excretion of salt as an adaptation strategy that allows for the control and regulation of salt contents in the organs of P. tarminiana plants, which are predominantly glycophytes.
The excretion mechanism is characterized by high selectivity for sodium and against potassium and calcium (Kim and Park, 2010). In addition, chloride excretion was observed in many halophytes. However, excretion of NaCl crystals has not been reported in P. tarminiana.
In accordance with Montaña et al. (2014), the emergence was statistically significant in seeds treated with 30 mM NaCl (0.71 dS m-1), and, with a higher concentration, 60 mM (1.25 dS m-1), 90 mM (1.69 dS m-1) and 120 mM NaCl (2.30 dS m-1 of the substrate), the emergence was reduced. A benefit in germination was observed with the addition of NaCl, and higher concentrations saw a decline in the chlorophyll contents of the seedling cotyledons. Thus, the ability to germinate and emerge under low saline stress conditions could indicate that the purple passion fruit possesses a genetic potential for salt tolerance, at least during the primary developmental stages.
According to Miranda et al. (2010), this behavior does not necessarily suggest that plantlets initiated in saline stress conditions can continue to grow and complete their adult plant life cycle under these conditions. Casierra-Posada et al. (2011) evaluated the growth of banana passionfruit seedlings (P tripartita var. mollissima (Kunth.) L. H. Bailey) under saline stress and found that the leaf area and dry matter (DM) production were drastically reduced by salinity, especially by 80 mM NaCl. The DM partitioning was also affected by salinity, reducing DM accumulation in leaves and increasing the DM in petioles + stems + shoots and roots. The findings from that study indicated that banana passion fruit seedlings are moderately sensitive to salt stress.
Meanwhile, the impact of salt restriction in the development and abundance of the structures involved in salt excretion, such as glands, have not been investigated. P. tarminiana specie was considered, previously evaluated, characterized and selected in several producing regions of Colombia, based on the improvement program (Ocampo et al., 2017), highlighting the need of identifying an accession of Passifloraceae family capable to tolerate stressful conditions. Thus, the purpose of this work was to investigate the ability of P. tarminiana to thrive under high salinity levels, to identify the mechanism for Na excretion and, finally, to find morphological and structural features that can explain this plant's tolerance.
MATERIALS AND METHODS
This study was conducted between January 2015 and February 2016 under hydroponic conditions in a greenhouse at the Universidad de Caldas, with the coordinates 5°03'23.31" N at 75°29'41.56" W an altitude of 2,130 m a.s.l., an average external temperature of 18°C, an average annual rainfall of 2,000 mm and an average relative humidity of 78%.
Plant material and saline treatment
As plant material P. tarminiana specie of commercial interest was considered, previously evaluated, characterized and selected in several producing regions of Colombia, based on the improvement program (Ocampo et al., 2017); procedence from the University of Caldas (Manizales, Colombia), identification (m15-Calmol01/2015) (Hurtado-Salazar, 2016). Passiflora accessions were collected in farmers' fields and sown in a germplasm bank, financed by the Ministry of Agriculture (Bogota, Colombia).
Two hundred forty seeds of commercial accession m15-Calmol01 (P. tarminiana) were sown, germinated in a hydroponic bed containing rice husk as the substrate. The moisture was maintained until the time of transplant. After 15 d of germination, 54 seedlings were selected, ensuring the greatest possible uniformity of the root system. Subsequently, the seedlings were transferred to hydroponic beds, 6.0x1.2 m, to ensure irrigation according to the preset treatments (33 and 100% water saturation and salt saturation levels EC: 2.5, 4.0 and 5.5 dS m-1).
The vertical shoot driving system was used (one thread), with a No. 12 galvanized wire, at 2 m from the floor of the greenhouse hydroponic beds. The seedlings were staked to a single stem, with weekly pruning of the side branches. When the main stems surpassed the wire by 20 cm, they were bent downward, forming a loop, and the secondary and tertiary branches were maintained.
The salinization treatment with NaCl applications (25, 40 and 55 mM) was initiated 8 d after transplantation to achieve the desired electrical conductivity in each treatment. The irrigation with each solution had a treatment of salinity. In the control treatment, the plants were watered with a nutrient solution without a further increase in salts. The experiment had a completely randomized design with a split-plot arrangement, where the plot contained the water saturation factor (33 and 100%), the subplot contained the salt saturation levels, with five replicates, and the experiment unit was composed of a single plant.
Hoagland and Arnon's nutrient solution (1950) was used, as modified by Niu et al. (2015). To ensure the salinity of the treatment, each solution was brought to an EC value with the addition of reagent level sodium chloride (99% purity). The nutritional solution was renewed once a week. After 7 months of treatment, the third fully expanded upper leaves were selected for scanning analysis of the epidermal structures.
A quantitative characterization was carried out using four samples of the leaf micromorphology from digital images of the adaxial and abaxial leaf surfaces, recorded in an electron microscope for environmental scanning (SEM Scaning Eletecron Microscope, QUANTA 250 FEI, Philips, Eindhoven, The Netherlands). Ten repetitions of each leaf sample were taken. The epidermal structures and stomatal density were analyzed with direct counting in the micrographs of the different samples of fresh leaves. The samples were collected from the upper leaves from the third pair of the apex and the last basal pair of the plants in the saline treatments. Similarly, the elemental composition of the leaves was determined with energy dispersive X-ray microanalysis (EDX model GENESIS APEX2i) coupled to a scanning electron microscope (SEM) as described by Hunsche et al. (2010). In the SEM-EDX procedure, the X-rays were recorded separately on both sides (adaxial and abaxial) of fresh leaves, as well as the salt crystal deposits. The equipment settings (working distance of 10 mm, 0.3 Torr sample chamber pressure, 20 kV beam energy, 5.3 spot size and 509 amplification) were optimized to collect X-rays from the surface layers of the tissue, which were kept constant during the measurements. Micrographs were taken randomly from the mid portion of the second upper leaf.
For the statistical analysis of the results, an analysis of variance and mean comparison tests were performed with Duncan's test, using the SAS (Statistical Analysis System, v. 9.2, SAS institute, Cary, NC, USA) statistical package.
RESULTS
In the plants grown in high salinity (5.5 dS m-1), salt excretion was done through crystal deposits on the surface of the leaves (Fig. 1 - a1, b1 and c1). In the control treatment, however (2.5 dS m-1), no presence of crystals was observed, and an increase in the density of salt crystals was denoted with the increase of salinity in the medium, from 2.5 to 5.5 dS m-1. In the SEM micrographs (Fig. 1 - b2, c2, d1, d2 and d3), the salts were crystallized as a cubic system, which is well known as the NaCl system (Driesner and Heinrich, 2007). This hypothesis of ion exclusion is corroborated with an EDX microanalysis (Tab. 1). This analysis showed a higher presence of sodium in the lower leaves, as well as the other analyzed ions.
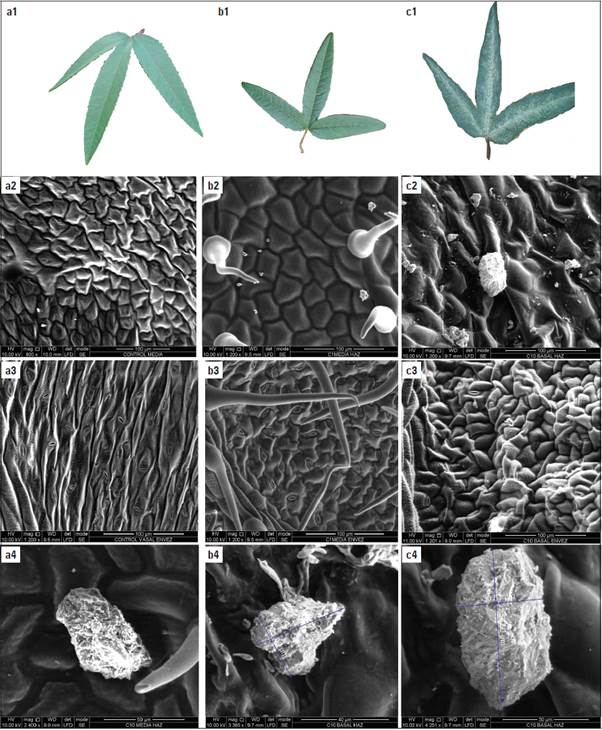
figure 1 a1, b1 and c1: salt excretion from the adaxial side of Passiflora tarminiana leaves treated with 2.5; 4.0; and 5.5 dS m1, observed with the naked eye. a2, b2 and c2: micrographs of the adaxial side of leaves of plants treated with 2.5; 4.0; and 5.5 dS m-1, a3, b3, and c3: micrographs of the abaxial side of the leaves of the plants treated with 2.5; 4.0; and 5.5 dS m-1. d1, d2, d3: micrographs of salt crystals observed on the adaxial leaf surface of plants treated with 5.5 dS m-1.
Basal leaves were analyzed to check the effect of salt exclusion by "saline glands". The contents of ions in the secretions collected from the leaves of the seedlings samples were measured and expressed as percentages. White crystals, composed mainly of Na, K, Al and Si, were observed. In leaves sampled in the field, minimal amounts of K were found (0.04 to 0.67 in the middle and 1.87 to 2.38 in the basal part). The leaves grown with high salt concentrations showed higher amounts of secretion than the leaves of untreated seedlings. As observed, the abundant ions included Na+ (0.32 to 0.63 in the middle and 1.15 to 2.72 in the basal part) and Si+ (21.19 to 32.45 in the middle and 21.51 to 37.66 in the basal part) in the secretions of the seedling leaves (Tab. 1).
Table 1 Composition of the crystals present in Passiflora tarminiana leaves, both sides (adaxial and abaxial), in the mid and basal part of the stem of the plants. X-ray spectrum of salt crystal deposit on a leaf surface treated with 5.5 dS m-1. The spot diameter was 1 mm; the acceleration voltage was 25 kV; the analysis time was 15 s; and the working distance was 10 mm.
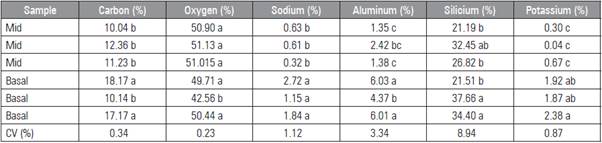
Means followed by different letters differ significantly according to the Duncan's test (P≤0.05).
P. tarminiana leaves are characterized by a smooth surface and trichomes on both leaf sides, whereas cuticular folds were observed only at the upper side along with stomata at the lower leaf lamina (Fig. 2). The abaxial surface was undulated because of a regular alternation of the ribs (Figs. 2B and 2D). Meanwhile, three epidermal structures were found on both sides of the leaves. Short trichomes were present with sharp edges (Figs. 2A and 2C) only in the adaxial side and along the edges. Papillae were observed as protuberances on the surface of the abaxial leaf epidermis. They were numerous and occupied the entire surface of the leaf (Figs. 2B and 2D). Stomata were present only in the abaxial leaf surface (Figs. 2B and 2D), classified as hypostomatic and with equal stomatal density, independent of the saline treatment. In the control plants, the stomata protruded on the surface, while those treated with the saline medium (5.5 dS m-1) had stomata below the layer of epidermal tissue.
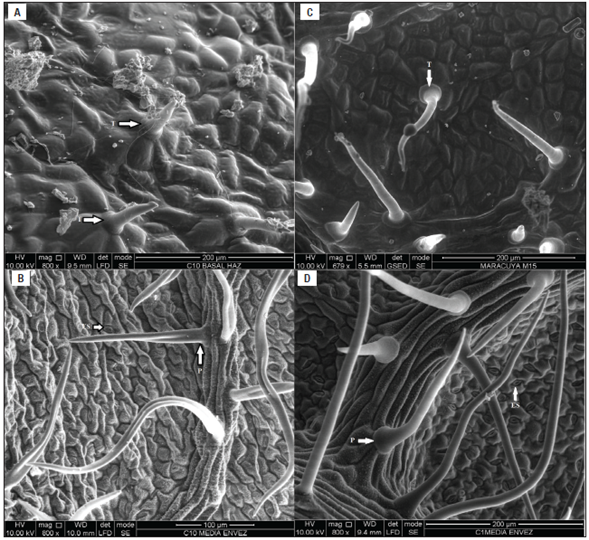
figure 2 SEM micrographs of adaxial (A, C) and abaxial (B, D) of the leaves of P. tarminiana treated with 5.5 dS m-1. T: trichomes, ES: stomata, and P: papillae.
The results showed that the salinity promoted variations in the stomatal density, with a range of 189.32 to 196.34 and a tendency to decrease as a consequence of increased salt concentration applications. Thus, the analyzed plant material was classified into two statistical groups (Tab. 2). The stomatal density was lower in the P. tarminiana leaves when the salinity levels and drought increased. The conditions of 5.5 dS m-1 and 33% moisture presented the lowest values of stomatal density with 189.32 stomata/mm2. The largest number of stomata was seen with 2.5 dS m-1 and 100% moisture with 196.34 per mm2 (Tab. 2).
DISCUSSION
According to Shin et al. (2012), high selectivity for sodium excretion was found in Glaux maritime L. However, in other species, small crystals were found with relatively large amounts of potassium, calcium and magnesium salts among those excreted in Odyssea paucinervis Stapf and Tamarix aphylla (L.) H.Karst. (Somaru et al., 2008). In addition, an EDX microanalysis showed that the salt deposited on the surface of the leaves of plants under salt stress was essentially sodium (Tab. 1). Each crystal contained many cube-shaped elements and probably a salt gland below (Fig. 1 - d1, d2, d3). Hunsche et al. (2010) studied the mechanisms of salt tolerance in seedlings of fiori (Grewia tenax Forssk.) and tamarind (Tamarindus indica L.) grown under controlled conditions and treated daily with NaCl solutions and confirmed with energy dispersive X-ray microanalysis that, in both species, the absorption of sodium and chloride increases with increased NaCl concentrations in the treatment solution.
Sodium is excluded from the tops of many plants, and hence accumulation of this ion in the leaves of such plants, which apparently are rather sensitive to it, is avoided. The barrier to sodium transport is effective at moderate external sodium concentrations. At high external concentrations, and as a result of prolonged growth in a saline medium, sodium contents are found to increase in the stems and sometimes also in the leaves of so-called Na non-accumulator plants. It is assumed that sodium movement might be restricted by the membranes of certain extrastelar tissue, excluding it from the vascular system. According to Yoshida et al. (2016), the autoradiographs of bean plants (Phaseolus vulgaris L.) show that Na22 is retained in the basal parts of the plant.
In general, the P. tarminiana species uses specific functional cellular mechanisms to prevent or remedy the harmful effects of absorbed salts. When plants are acclimated to an altered environment, they are subject to adjustments in leaf morphology, chloroplast composition (pigment) and activity of biochemical processes that prevent oxidative damage to the photosystem (Saied et al., 2010). These events take place simultaneously and quickly in order to mobilize all the metabolic apparatus for adaptation and survival of the plants. The adaptation of plants to NaCl involves metabolic events such as synthesis of organic solutes and ion partitioning (Moriuchi et al., 2016). However, responses to salinity include changes in leaf properties such as thickness, surface micromorphology and cuticular wax. These results contradict Casierra-Posada et al. (2011), who suggest that salinity does not only reduce the growth of banana passion-fruit seedlings, but also alters the DM partitioning in different plant organs, as an adaptive mechanism to salinity. This study suggested that the banana passionfruit is moderately sensitive to saline stress. In another study by Casierra-Posada et al. (2013), a DM reduction closely followed the trend in the photosystem II maximum quantum yield (Fv/Fm), which suggests that the major impact of salinity on the banana passionfruit plants was due to negative effects on photosynthesis.
In this Passiflora, Na+ secretions of the fractions are probably mediated by two different mechanisms (accumulation and excretion), which, according to Kim and Park (2010), can be independent. In this study, it was found that salt excretion began on the fourth week of the saline treatment. Therefore, the independence and the succession of two mechanisms seemed obvious. The salt absorbed by the roots is probably accumulated in the tissues and then excreted by glands. Moreover, according to Casierra-Posada et al. (2011), salinity stress imposes additional energy requirements on plant cells and diverts metabolic carbon to storage pools so that less carbon is available for growth.
The number of stomata per area may vary depending on the environmental conditions (humidity, temperature, light), to which the leaf is subjected during cell division and elongation (Arioli et al., 2008). Several studies have shown that, with a higher intensity of salinity of the solution, there is a decrease in stomatal density (Reyes-López et al., 2015), which provides a greater distance between the stomata, leading to a reduced transpiration. There is a hypothesis that, despite morphological and physiological differences, a species may have stress tolerance mechanisms and remediation expressed by metabolic changes induced by salinity and drought. A methodological view with negative consequences for this study says that the seedlings were grown from seeds and, therefore, there was a genetic variability of unknown magnitude (Saied et al., 2010), which may have affected the response to the addition of salt in the measured parameters although further studies to reinforce this hypothesis are needed.
In P. tarminiana, excess salt was probably secreted by glands distributed throughout the adaxial leaf surface. The glands appear in longitudinal lines in accordance with the Na+ in the adaxial surface (Fig. 2D). These glands were relatively large compared to other epidermal structures. According to Esteban et al. (2013), this expulsion of ions is fully reversible and its expression diminishes when conditions become favorable (low salinity). The dissolution of salt crystals may occur, forming drops associated with the transpiration of the plant. Therefore, as a detoxification mechanism developed mainly to avoid osmotic imbalance in tissues, salt excretion by leaves is an example of "adaptation", as it has positive effects on the photosynthetic performance of plants, protecting them from overheating and stress from excess radiation during the most severe periods. This same author, in different species such as Mesembryanthemum crystallinum L., observed that sodium accumulates and is deposited in a gradient along the growth axis, with the highest concentration deposited on parts of the youngest leaves where it is sequestered within the central vacuole the cells. This is in contrast to what happens in glycophytes, which drive the sodium to the mature parts of the plant in an attempt to maintain functional roots.
In addition to the removal of sodium from photosynthetic tissues, bladder cells act as water deposits for photosynthetic cells (mesophilic) located within the leaf (Esteban et al., 2013). Possibly, this is the behavior in P. tarminiana. In general, P. tarminiana seems to be able to survive with salinity up to 5.5 dS m-1 (NaCl), which can be considered as high, with the removal of toxic ions. Possibly, the excretion of excess salt was done through epidermal glands. Sodium, in addition to other inorganic ions, is used for osmotic adjustment. Further research is needed to clarify the epidermal structures that excrete salt in stressed plants of the Passifloraceae family.
CONCLUSIONS
Passiflora tarminiana presented a mechanism of tolerance and adaptation in conditions of abiotic stress resulting from excess salinity through the excretion of salts in the lower leaves, possibly through the presence of salt excretory glands. In addition to salt excretory glands, other structures of the epidermis such as papillae and trichomes were observed, all of them probably involved in P. tarminiana salinity tolerance.