INTRODUCTION
The State of Goiás, one of the largest corn producers in Brazil (Okumura et al., 2013), has been experiencing droughts and poor rain distribution (Brunini et al., 2001). The quality and productivity of this plant, with significant economic and social importance, have been affected (Berlato et al., 2005). Climatic effects, such as El Niño (Li et al., 2011), have caused more frequent dry periods and water stress condition (Doto et al., 2015). Corn farmers are irrigating their crops, but at high costs (Montero et al., 2013). In addition, rainfall scarcity has reduced water levels in reservoirs.
Sweet corn (Zea mays var. saccharata) (Poaceae) cultivation is increasing in Brazil (Teixeira et al., 2001) in order to supply agroindustries that process raw material for commercialization. This has increased the income of small and medium sized farmers and jobs on a macro scale (Barbieri et al., 2005). The sweet corn cultivation system in the State of Goiás has prospects for expansion since Brazilian agriculture is one of the major producers of common corn (Zea mays) in the world (Okumura et al., 2013). Syngenta dominates the sweet corn seed market in Brazil; the Tropical Plus® hybrid has the highest commercialization.
Zea mays is tolerant to water deficits during the vegetative phase, but exhibits high sensitivity if this condition prevails during the reproductive phase (Santos et al., 1998). Therefore, technologies that amortize the effects of water stress in economically and socially important plants, such as sweet corn, should be assessed. Silicate fertilization has been viewed as a technology that mitigates the negative effects of stress factors on plants (Ma and Yamaji, 2006) although it is not yet widely used by Brazilian farmers. Silicon has been used as an elicitor of resistance in plants under conditions of abiotic stress, such as saline, heavy metal poisoning or water stress. The accumulation of Si in transpiration organs causes the formation of a double layer of silic, with a subtle decrease in opening of the stomata, reducing leaf transpiration, which restricts the loss of water without influencing growth (Oliveira and Castro, 2002).
The corn plant has a natural ability to respond favorably after exposure to exogenous Si sources because it accumulates this element (Takahashi et al., 1990). The concentration of Si in this plant is linked to aerobic respiration, increasing its accumulation in soils or hydroponic solutions that hold considerable amounts of silicon. In summation, the genes ZmLsi1 and ZmLsi6 present in corn plants are responsible for the transport of Si soil solutions to root cells and xy-lem cells for the balance of plants, respectively, demonstrating the adaptability of this plant to Si (Mitani et al., 2009).
The beneficial effects of Si on corn plants in Brazilian agricultural are still neglected in the field, while increasingly scientific results have proven the high adaptability of these plants. The fact that Brazilian soils, especially those located in the Cerrado biome, are poor in soluble Si accessible to plants (Korndõrfer et al., 2004) may explain this fact. Farmers often avoid using silicate fertilizers for fear of increasing production costs. Nevertheless, the use of fertilizers as an exogenous source of Si in sweet corn, via soil or foliage spraying, has great potential for stimulating level of resistance to water stress (Marques et al., 2016). The outcomes of the present study may encourage the use of silicate fertilization in corn plants under water stress.
The aim of the present study was to evaluate responses in the production parameters of sweet corn hybrid Tropical Plus® under water stress with doses of potassium silicate sprayed on the leaves.
MATERIAL AND METHODS
This experiment was carried out in an experimental area of the "Instituto Federal Goiano-Campus Urutaí" located at Fazenda Palmital, rural region of the city of Urutaí, Goiás State (Brazil). Experimental site is located at 17°29'10 "S and 48°12'38" W at 697 m a.s.l.
The sweet corn plants were cultivated in a greenhouse, simple arc type, with an East-West orientation, made with a metallic structure and the dimensions: 30 m length, 7 m width, and 6.2 m arc height, covered with a low-density polyethylene (LDPE) film (0.15 mm thick) and a anti-aphid mesh on the sides.
The climate of the region is classified as tropical at altitude with a dry winter and rainy summer, Cwb type according to the Kõppen classification. The soil of the experimental area was classified as Dystrophic Yellow Red Latosol (Embrapa, 1999) with a sandy loam texture and physical and chemical characteristics presented in Table 1. For the physical and chemical analysis of the soil, five samples were collected inside the greenhouse. The samples were homogenized for a representative composite sample using the 0 to 20 cm and 20 to 40 cm layers of depth. The soil samples were sent to the "Laboratório Agropecuário Ltda. (SOLOGRIA)" in the city of Goiânia, State of Goiás, to obtain the technical report.
The sweet corn Tropical Plus® hybrid was used (Syngenta Seeds Ltda), provided by the company Conservas Oderich SA (Orizona, Goiás), which produces canned vegetables, including sweet corn, and who supplied the technical information needed for the sweet corn plants. The sowing was performed on April 30, 2015 with a spacing of 80x25 cm, with three seeds per hole, 2 cm deep. The fertilization was carried out following the recommendations of Trani et al. (2011).
Table 1 Physical and chemical properties of the soil used to grow the Tropical Plus® hybrid corn (Syngenta) in a greenhouse on the IF Goiano Urutaí campus, Goiás State, Brazil
Physical properties | |||||
Deep | Coarse sand | Thin sand | Silte | Clay | Textural Class |
(cm) | g kg-1 | ||||
0-20 | 275 | 324 | 241 | 160 | sandy franc |
21-40 | 329 | 283 | 202 | 186 | sandy franc |
Deep | pH | MO | P i 1 resina | H+Al | K |
(cm) | CaCl2 | g dm-3 | mg dm-3 | mmolc dm-3 | |
0-20 | 6.0 | 24 | 300 | 21 | 4.98 |
21-40 | 5.7 | 16 | 280 | 20 | 4.34 |
Deep | Ca | Mg | SB | CTC | V |
(cm) | cmolc dm-3 | % | |||
0-20 | 57 | 22 | 84 | 104 | 80 |
21-40 | 55 | 14 | 73 | 93 | 78 |
Report issued by the Laboratório Agropecuário LTDA (SOLOGRIA), Goiânia municiplaity, Goiás State, Brazil.
The experiment design was a randomized complete block with subdivided plots in a 4x4 factorial scheme, with four concentrations of potassium silicate (0, 6, 12 and 24 L of K2SiO3 ha-1) and four soil water tensions (15, 30, 45 and 60 kPa), totaling 16 treatments, with four replications. The experiment consisted of four useful beds (the experiment blocks) with two planting lines per set, except for the borderlines, which were placed at the lateral ends of the greenhouse with just one planting line. Each planting line received an irrigation lateral line, a drip tube and auto-compensated driplines spaced 0.3 m apart, totaling 8 useful lateral lines and two located in the margins.
The soil adaptation was done by plowing with a mini motorized tractor, model TC 14, 14 HP (Yanmar Agritech®) with a rotary hoe. The beds were 7 m long and 1 m wide and had manually hoed, totaling 16 beds throughout the experiment.
A drip irrigation system was used, where each planting line received a 16 mm lateral irrigation line, with emitters spaced every 0.30 m. The emitters provided a flow of 1.4 L h-1 with a service pressure of 1 kgf cm-2. The pumping system consisted of a 1 HP motor pump assembly. The fertilizer injection was carried out using the suction system of the pumping set, as pushed by a set of manual controlling valves. Soon after the pumping system was installed, a 120 Mesch screen, in addition to recording the pressure of the irrigation system. A water distribution uniformity test was executed following recommendations of Borssoi et al. (2012) after the irrigation system was installed. The previously calculated water distribution uniformity was 97%.
The irrigation management was done with tensiometry using soil water retention curves (Tahir et al., 2012) and adjusted by the model proposed by Van Genuchten (1980) with the aid of the Soil Water Retention Curve (SWRC® software) version 3.0 (Dourado-Neto et al., 2000). Two puncture tensiometers at depths of 20 cm and 40 cm were installed to monitor the soil water tension for each experimental plot (totaling 32 tensiometers). The readings of the soil water tension values, quantified by the tensiometers, were measured using a digital tensometer (SondaTerra® model).
In the first 30 d after planting, the same irrigation management was used for all treatments in order to assure the natural establishment of the plants. The irrigation was similar for all plots with permanent soil moisture control at near field capacity. In the first two days after seeding, a 20 mm blade was applied, followed by daily levels close to 2 mm according to the soil water retention curve in order to maintain the soil at close to the mean matric potential (-10 kPa).
The potassium silicate was applied via foliar sprayings in the subplots with the help of a manual action costal sprayer (20 L). Drift protection was used to ensure maximum application precision and to avoid contamination of the adjacent subplots, which did not receive the doses of potassium silicate. An aggregate of four applications was done throughout the experiment.
Throughout the sweet corn plant cycle, cultural treatments were performed, such as cutting of plants and leaving a single plant per hole. The dominance of invasive plants was dealt with during the crop cycle through manual weeding. The phytosanitary control was done according to the need for prevention and control throughout the experiment.
All production parameters were quantified at 95 d after planting, in accordance with the average cycle of the Tropical Plus® hybrid, which is earlier than that of the common corn (Okumura et al., 2013). The following plant parameters were quantified: 1st ear (cm) height, 2nd ear (cm) height, ear length with (cm) and without straw (cm), ear diameter with (cm) and without straw (cm), ear weight with (cm) and without straw (cm), cob diameter (cm), cob fresh weight (g), number of rows per cob, unit grain depth (mm), unit grain width (mm), fresh (g) and dry (mg) weight of total grains per cob, fresh (g) and dry (mg) weight of a unit cob, fresh (g) and dry (mg) weight of total straw per plant, fresh (g) and dry (mg) stem weight, fresh (g) and dry (mg) weight of all leaves per plant, number of grains per row per ear, ear yield (t ha-1), grain yield (t ha-1) and industrial yield (t ha-1).
All quantified data were checked for assumptions with analysis of variance. The normality was verified with the Lilliefors adherence test and, in a complementary way, visually using the symmetry of the histogram obtained by the SAEG (System of Statistical and Genetic Analysis) (Ribeiro and Melo, 2009). According to this procedure, all the quantified variables followed normal distribution and, therefore, the values of their averages, as disclosed in the figures and tables, were presented in full, without the need for transformation.
After confirmation of the implication (or not) of the factors under interaction or alone according to the ANOVA with a factorial arrangement, the means were compared using the Tukey test at the 5% probability level. Statistical analyzes (ANOVA and means tests) were done with SAEG, while the images were constructed in SigmaPlot®, v. 11 (Systat Software Inc).
RESULTS
The soil water tensions and potassium silicate doses under interaction did not affect the yield parameters 1st ear height (F = 0.20, gl=36, P>0.05, CV=10, (F = 1.24, gl= 36, P=0.04, CV=9.42)), 2nd ear height (F = 0.18, (F = 1.34, gl=36, P=0.25, CV=5.68)), stem-to-straw diameter (F=1.24, gl=(F=1.00, gl=36, P=0.45, CV=20.25)), grain depth (F = 0.30, CV=4.03) (F = 0.38, gl=36, P>0.05, CV=12.41), fresh (g/ F = 0.66, gl=36, P>0.05, CV=14.45) and dry (F = 0.37, gl=36, P>0.05, CV=13.26), (F=3.40, gl=36, P=0.41, CV=10.12) grain weight, fresh weights (F=0.96, gl=36, P>0.05, CV=11, F = 0.67, gl=36, P=0.05, CV=14.06), fresh (F=0.67, CV=28.07) and dry (F = 1.29, gl=36, P=0.27, CV=21.08) stem weight, fresh weight (F = 0.84, gl=36, P>0 (F = 1.68, gl=36, P=0.12, CV=11.45)), number of grains per row (F = 0.73, gl (F = 0.66, P=0.05, CV=14.45)) and industrial yield (F = 0.05, CV=7.79) 51, gl=36, P>0.05, CV=12.97), isolated or not.
The parameters spike weight (F = 3.41, gl=36, P=0.02, CV=9.68), without straw (F=4.13, gl=36, P=0.01, CV (F = 6.52, gl=36, P=0.04, CV=9.81), fresh cob weight (F = (F=3.46, gl=36, P=0.02, CV=5.85) and ear yield (F=3.41, (Fig. 1) were affected by the soil water stress (Fig. 1).
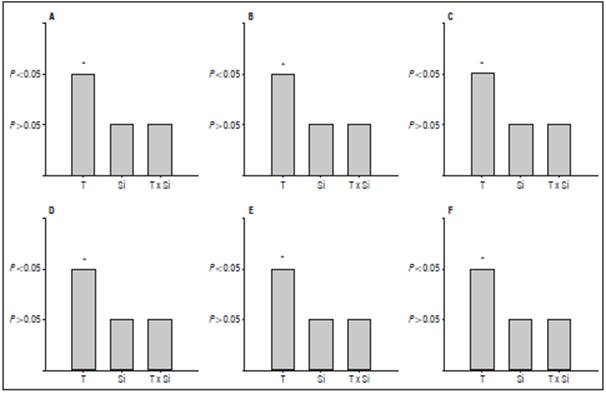
Figure 1 Summary of the ANOVA (factorial with subdivided plots) of the soil water tensions (T) (plots) and potassium silicate doses (Si) (subplots), isolated or under interaction (T x Si), for the significant production parameters (Test F) of the sweet corn (Zea mays var. Saccharata) hybrid Tropical Plus®. Instituto Federal Goiano, Urutaí, Goiás, Brazil. A: spike weight with straw; B: spike weight without straw; C: corn cob diameter; D: corn cob fresh weight; E: number of rows per cob; F: spike yield.
Higher values for shank weight, with and without straw, were reached at 15 and 30 kPa, as compared to the 45 and 60 kPa voltages (Fig. 2). The diameter of the cob, fresh weight of the cob and number of rows per spike were also higher with the tensions between 15 and 30 kPa (Tab. 2). The tension of 60 kPa had lower values for these parameters, including the ear yield (Tab. 2).
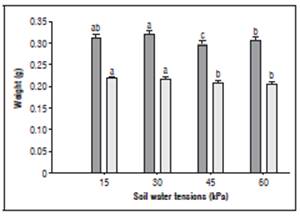
Figure 2 Weight (mean ± SEM) of sweet corn (Zea mays var. Saccharata) Tropical Plus® hybrid spikes with straw (dark gray bars) and without straw (light gray bars) under the effect of soil water tensions (kPa). Instituto Federal Goiano, Urutaí, Goiás, Brazil. Means with different letters, for each variable, indicate significant differences according to the Tukey test (P<0.05).
Table 2 Soil water tensions (SWT), cob diameter (CD), cob fresh weight (CFW), Rows number per ear (RNEAR) and spike yield (SY) (SWST) (mean ± EP1) of sweet corn (Zea mays var. Saccharata) Tropical Plus® hybrid (Syngenta) under different soil water stresses tensions (kPa). Federal Goiano Institute, Urutaí, Goiás, Brazil

Means with different letters, per column, indicate significant differences according to the Tukey test (P≤0.05).
DISCUSSION
The lack of effect of the soil water tensions and potassium silicate doses, isolated or under interaction, was observed on most yield parameters. The interaction between these two factors should be important for maize plants as a result of giving resistance to water stress, as previously reported (Marques et al., 2016). The absence of significant effects of the Si dose factor (isolated) on the productive parameters of the sweet corn did not necessarily imply that it had no influence on the results. The physiological and biochemical effects of Si under stress conditions such as water balance, reduction of oxidative stress and maintenance of adequate mineral absorption by the root system (Zhu and Gong, 2014) were entirely ignored in the present study. The fact that the water stress did not affect most sweet corn yield variables may have been due to the physiological and biochemical effect from the Si in the sweet corn plants. Si can mitigate water stress effects on plants of economic importance, such as Zea mays (Gao et al., 2006). This may have partially been noted in the present study with the absence of a soil water tension effect in most production parameters that normally respond to water stress.
Differences in the yield parameters were more evident when different maize genotypes were compared (Santos et al., 2004). The similar effect of the genetic configuration of the sweet corn Tropical Plus® hybrid in all treatments may also be responsible for the lack of effect on the evaluated yield parameters. Zea mays is relatively tolerant to water stress during the vegetative stage, but extremely sensitive if the stress condition persists during the reproductive stage (Song et al., 2010). Different soil water stresses were managed in the sweet corn plants during their entire vegetative and reproductive cycle, including in the stage between pre-flowering and grain filling. This stage is critical, where the plant can no longer satisfactorily recover from a water stress experienced in previous stages (Çakir, 2004). Therefore, the hypothesis that sweet corn adult plants have attenuated the negative effects of water stress experienced during its vegetative phase must be discarded. This would be an effective strategy for the plant to overcome stress conditions experienced in the vegetative stage without reducing yield parameters in the reproductive stage (Ferreira et al., 2011).
The effect of the soil water tensions, with lower values for the sweet corn ear weight, with and without straw, at the higher ones, agrees with reports that indicated sensitivity to water stress (Almeida et al., 2016). Lower values for cob diameter and cob fresh mass under water stress conditions was reported (Parizi et al., 2009). These variables are strong yield indicators in corn crops because, the greater the corn mass, the greater the grain mass is (Shaheenuzzamn et al., 2015). This may also explain, in part, the significant differences for cob diameter and fresh weight as productivity spiked with the different soil water tensions. However, it does not fully explain why this factor did not affect the other yield parameters of the corn ear yield. The soil water tension effect may have been compensated for by the potassium silicate doses even though this factor (Si doses) did not affect most yield production parameters.
The lowest variation in the means of rows per spike, among the parameters affected by soil water tensions, may have been due to the genetic component of the evaluated Tropical Plus®hybrid, agreeing with other studies that also did not report differences (Ferreira et al., 2011). The decreasing number of rows per spike as the soil water stress increased was also seen in a study that evaluated the effect of different irrigation levels and calcium silicate doses on the common corn material BR 106 (Marques, 2013). The average number of rows per ear of sweet corn (independent of the soil water tensions content) was close to the 16 rows per spike that sees good acceptance on the Brazilian consumer market, reinforcing the genetic resistance of the Tropical Plus®hybrid even with water stress (Pereira et al., 2002).
The maximum productivity of the Tropical Plus® hybrid (~28 t ha-1 at 30 kPa) was higher than that indicated by the Syngenta company in Brazil (~17 t ha-1) (www.syngenta.com.br). This value varies when increasing crop yields while increasing the Tropical Plus® hybrid plant population from 40,000 to 100,000 plants/ha with a maximum yield of ~9 t ha-1 for winter-spring cultivations and ~12 t ha-1 for summer crops under field conditions (Souza et al. 2013a; Souza et al. 2003b). The Vivi, Tropical Plus® and Dow SWB 551 hybrids, with 5.73, 5.35 and 2.0 t ha-1, respectively, have also had similar ear yield production in the field (Teixeira et al., 2009). Differences in productivity results may be explained by the fact that this experiment was conducted in a greenhouse, reducing the influence of biotic and abiotic effects on the sweet corn plant production (Rosa et al., 2016).
The sweet corn cultivars KSC403, Merit and Obsession in Iran (Mashhad) had a similar response pattern, with decreasing growth under water stress condition (Tafrishi et al. 2013). The yield parameters had high sensitivity to the water stress, being a very responsive component in water stress evaluations in Zea mays species (Moradi et al. 2012).
Water stress reduces sweet corn plant productivity with losses to the corn agribusiness in Brazil. The role of silicon as an elicitor of resistance against water stress effects has been reported, and, for this reason, the non-significant effect of the Si (isolated or under interaction with the soil water tensions) should not be neglected. The spraying of potassium silicate throughout the vegetative stage on the sweet corn plants allowed for the absorption of silicon and its storage and transport to the plant yield structures (Mitani et al., 2009). This indicates a higher plant affinity with silicon in the vegetative stage than in the reproductive stage. Silicon may be important in post-harvest tomatoes (Marodin et al., 2016), another important plant in Cerrado biome agriculture