INTRODUCTION
The lisianthus (Eustoma grandiflorum (Raf.) Shinn.) is an ornamental plant originating from northern Mexico and the southern United States (Kuronuma et al., 2018). This species belongs to the Gentianaceae family and is cultivated as cut flowers. The flower was introduced to the Brazilian Market in the late 80s and began to stand out economically in the 90s, arousing farmers' and consumers' interest (Camargo et al., 2004; Souza Neto et al, 2017).
Nevertheless, lisianthus commercial production in soil has been limited by viruses and pathogens, such as Fusarium solani, which attacks the root system and causes stem and root rotting and, as a result, wilting and drying of the plant (Backes et al., 2007). The diseases become limiting factors for the crop, leading to the search for new production techniques.
Soilless cultivation using substrates allows for horticultural crop production in inappropriate areas, such as dry regions or soils with low drainage capacity (El-Behairy, 2015). The production of cut lisianthus in substrates can represent an important technological advance since it reduces the occurrence of pests and diseases and optimizes the workforce, by allowing cultivation in high soil beds (Asaduzzaman et al., 2015).
In Brazil, soilless flower cultivation is little-explored; most of the growers who use this system usually cultivate in pots with free drainage. However, costs with pots are high since many of them cannot be reused from one cycle to another. In addition, waste of water and fertilizers is very high, which causes environmental problems. Some fruit growers have been using trough crop systems, in which the substrate is placed directly into gutters, eliminating pot costs (Neto and Redig, 2017). However, the trough growing system with nutrient solution recirculation ("closed system") has not been studied for cut flower cultivation in Brazil.
The use of materials available in the producing region for the composition of substrates is very important in order to enable economic viability in flower cultivation with this cultivation system in terms of their availability, cost and asepsis, among other characteristics (Barbosa et al., 2019).
The carbonized rice husk or the raw rice husk and mixed with organic compost can be good substrate alternatives for use in southern Brazil, as it is a material with great availability and low cost (Kratz et al., 2013).
According to Klein et al. (2012), carbonized rice husk presents great potential for use as a substrate given its physical properties. It presents fast and efficient drainage and high aeration space, providing good oxygenation to roots (Caldeira et al., 2013). Another benefit of this material is the lack of propagules of spontaneous plants, nematodes and pathogens as a result of the carbonization process, which eliminates possible organisms (Asaduzzaman et al., 2015). However, the rice husk carbonization process demands high labor, can cause air pollution and provides low profits once there is a reduction of 50% in the husk volume. Therefore, raw rice husk may be an alternative material instead of the carbonized rice, as observed for fruits in closed growing systems (Strassburger et al., 2011; Peil et al., 2014). This material has great availability, easy access and low cost in Brazil's southern region (Zorzeto et al., 2016).
In addition, information regarding lisianthus plant growth (dry mass production and partitioning) is incipient. Dry mass production and partitioning analysis expresses a plant's morphophysiological conditions and quantifies the net production derived from the photosynthetic process. It represents the performance of the assimilatory system during a certain period (Benincasa, 2003). Growth is influenced by several genetic and environmental factors (Osei et al., 2018). Thus, the knowledge on the growth of a specifically cultivated variety and the effects of the growing medium is very important.
The experiment design used randomized blocks with four treatments (substrates) and six replications of 10 plants. Three control plants were harvested for the evaluations. The border plants were disregarded. The results were subjected to analysis of variance, and the means were compared using the Tukey test, with a probability of 5%.
Therefore, the aim of this study was to evaluate the plant growth and quality of lisianthus cultivated in different substrates based on rice husk, with the purpose of generating information that potentiates production in trough growing systems with leaching recirculation.
MATERIALS AND METHODS
This experiment was carried out in a greenhouse at the UFPEL Campus in the municipality of Capão do Leão, Rio Grande do Sul, Brazil (31°52'S, 52°21'W). The climate of this region is characterized by being sub-tropical, with a hot summer, Cfa according to Kõppen's classification (Kuinchtner and Buriol, 2001). The greenhouse environment was managed daily only with natural ventilation, opening the doors and the side windows.
Four substrates were used: carbonized rice husk (CRH); raw rice husk (RRH); CRH (70%) + S10 Beifort® commercial organic compost (30%); and RRH (70%) + S10 (30%). The physical and chemical properties of the substrates were determined in the LASPP/FEPAGRO (Laboratory of Substrates for Plants / Agriculture Research State Foundation) (Tab. 1).
Table 1 Physical and chemical properties of raw rice husk (RRH) and carbonized rice husk (CRH) substrates, used alone and mixed with commercial organic compost S10 (Beifort®) at a proportion of 30%, at the beginning and at the end (91 d after setting) of lisianthus (Eustoma grandiflorum) cultivation in troughs with leaching recirculation.
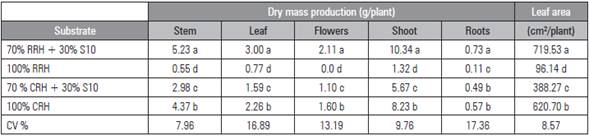
* WEA: water easily available; WHC: water holding capacity; EC: electric conductivity.
'White Excalibur'® cultivar was used and the experiment was carried out on October 9th, 2015, when the commercial seedlings presented about three to four leaf pairs.
The cultivation system was composed of four wood troughs (4.5 m long, 0.30 m wide and 0.15 m internal height). The troughs had a slope of 4% and were internally waterproofed with plastic (petroleum based geomembrane) channels to facilitate the flow of the drained nutrient solution to the catchment tanks. A 100-liter catchment tank was placed at the lowest end of each trough. A low-power washing machine pump was installed in each tank in order to propel the nutrient solution through a 20 mm PVC pipe to the highest point of the troughs. From this point, the nutrient solution was supplied to the plants through polyethylene pipes and drippers directed to the plant base. The nutrient solution was supplied for 15 min every hour from 08 a.m. to 07 p.m. The solution drained to the end of the troughs returned to the catchment tank, forming a closed system.
Each trough was divided into six plots, with two rows of plants. Spacing plants at 0.15 m, with ten plants per plot and sixty plants per trough. The nutrient solution recommended for chrysanthemum by Barbosa et al. (2008) was adapted and used for the lisianthus cultivation. It was monitored every 2 d by checking electrical conductivity (EC) and pH. The EC was adjusted to 1.5 dS m-1, and pH was maintained between 6.0 and 6.5. The monitoring of EC and pH was carried out with a manual digital conductivime-ter and pHmeter. Corrections were performed when the EC suffered variations above or below 20%. The pH was corrected with acids or bases when necessary.
The harvesting point was defined when the stem had two fully open flowers. The stem length was measured with a graduated ruler from cut point until upper portion of the highest flower. The stem diameter was measured in their lower third portion with a manual pachymeter that was also used to measure the flower diameter (distance between the petals borders on opposite sides). The leaf area was determined using leaf area meter equipment (LI-COR, model 3100). The open flowers and floral buds with ornamental potential were counted.
For the dry mass evaluations, the samples were fractionated into root, stem, leaves and flowers (when present). All plant fractions were dried in an oven at 65°C until constant weight and then weighed on a precision balance. As a result, the plant dry mass (DM) production was determined. Dry mass partitioning (in percentage) and the ratio shoot/root DM (g g-1) were calculated from the DM production data.
The four studied substrates were arranged in a randomized block experiment design with six replications composed of 10 plants. Three control plants of each replication were harvested for the evaluations. The border plants were not considered. The results were subjected to analysis of variance, and the means were compared with the Tukey test, at 5% probability.
RESULTS AND DISCUSSION
For plant growth, the highest shoot dry mass was obtained from plants grown in the RRH + S10 substrate, followed by CRH, CRH + S10 and, finally, RRH (Tab. 2).
Table 2 Dry mass production of stem, leaves, flowers, shoot and roots; and plant leaf area of lisianthus (Eustoma grandiflorum) plant cultivar 'White Excalibur' grown in different rice husk substrates in troughs with leaching recirculation.
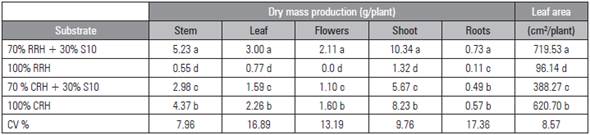
Means followed by the same letter in the column do not differ at the 5% probability according to the Tukey test. RRH: raw rice husk, CRH: carbonized rice husk, S10: organic commercial compost (Beifort®).
The accumulated DM production of the plants grown in RRH + S10 presented the following order (g/ plant): stem 5.23, leaves 3.00, flowers 2.11 and roots 0.73, totaling 11.07 (Tab. 2). The same order was observed in the CRH and CRH + S10 substrates. Similarly, a study developed by Hernandez et al. (2011) with the 'Echo Blue' lisianthus cultivar showed that the stem was the organ with the greatest DM accumulation, followed by leaves.
In contrast, plants cultivated in RRH had higher DM production (g/plant) in the leaves (0.77), followed by stems (0.55) and roots (0.11). Plants cultivated in RRH did not produce flowers. The absence of flowers can be ascribed to the very low total biomass production, only 1.43 g/plant. So, plant development did not occur properly in this substrate and, as a consequence, the plants did not produce flower stems.
For plant leaf area (PLA), the plants cultivated in RRH + S10 presented the highest average (719.53 cm2), differing from CRH (620.7 cm2), CRH + S10 (388.27 cm2) and RRH (96.14 cm2) (Tab. 2). The PLA of the plants cultivated in RRH + S10 and CRH substrates was close to that of the 'Echo Blue' cultivar, as observed by Hernandez et al. (2011).
The dry mass partitioning into the different plant organs (Tab. 3) showed similarity among the treatments RRH + S10, CRH + S10 and CRH. As expected from the DM production data (Tab. 3), the stems represented the highest fraction of the produced DM, followed by leaves, flowers and roots. On average, in the three substrates, stems comprised 48.4%; leaves, 26.2%; flowers, 18.4%; and roots, 7.0% of the total plant DM.
Table 3 Dry mass partitioning among the different plant organs and shoot/root dry mass ratio of lisianthus plant (E. grandiflorum) cultivar 'White Excalibur' grown in different rice husk substrates in troughs with leaching recirculation.
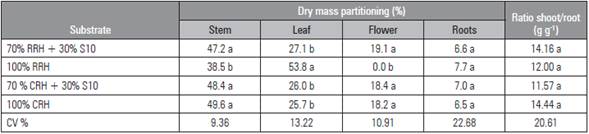
Means followed by the same letter in the column do not differ at the 5% probability according to the Tukey test. RRH: Raw Rice husk, CRH: carbonized rice husk, S10: organic commercial compost (Beifort®).
Plants grown in RRH substrate showed different DM distribution ratios. In this case, the leaves comprised 53.8% of the total plant DM, followed by stems (38.5%) and, finally, by roots (7.7%) (Tab. 3). This modification of the DM partitioning was attributed to the absence of flowers in the plants grown in this substrate.
The DM distribution to the roots did not differ among the substrates. The roots comprised, on average, 7.0% of total DM. Despite the different DM productions (Tab. 2), the ratio shoot/root DM was not affected by the substrates (Tab. 3). On average, this ratio was 13.04 g g-1. The ratio shoot/root DM indicates the root activity in the sense of providing water and mineral nutrients to the shoot in order to support adequate plant growth.
For plant quality (Tab. 4), the RRH + S10 and CRH substrates provided the longest stems, 65.1 cm and 61.8 cm length, respectively, differing from CRH + S10 (46.6 cm) and RRH (only 16.5 cm length).
Table 4 Stem length, stem diameter, number of open flowers, flower diameter and number of floral buds with ornamental potential in lisianthus plants (Eustoma grandiflorum) cultivar 'White Excalibur' grown in different rice husk substrates in troughs with leaching recirculation.

Means followed by the same letter in the column do not differ at the 5% probability according to the Tukey test. RRH: Raw Rice husk, CRH: carbonized rice husk, S10: organic commercial compost (Beifort®).
The plants grown in RRH 100% produced stems that were inappropriate for commercialization, differing significantly from the other treatments (Tab. 4). The first three substrates provided proper stem length regarding the marketable requirement, between 40 and 70 cm (Veiling Holambra, 2019). The plants grown in substrates RRH + S10 and CRH stood out in this characteristic since consumers prefer longer stems. Stems over 60 cm in length get a higher commercial price.
The plants produced in substrates RRH + S10 and CRH (Tab. 4) presented stems length similar to those found for 'Mariachi Blue Picotee' and 'Mariachi Blue' cultivars with perlite cultivation (De La Riva-Morales et al., 2013), but lower than those found for 'Echo' cultivar in soil cultivation (Camargo et al., 2004) and larger than those found for 'Echo Champagne', 'Mariachi Pure White', 'Echo Pink' and 'Avila Blue Rim' cultivars in soil cultivation (Backes et al., 2007). One has to take into account the fact that the exposed results are related to different cultivars and growing conditions. Thus, in addition to the plant genetic material, the growing system is a factor with a significant effect on plant development.
The substrates RRH + S10 and CRH provided plants with bigger stem diameters (SD), 6.4 and 6.1 mm, respectively (Tab. 4), followed by CRH + S10 substrate, with 5.4 mm, and RRH (2.8 cm). SD is an important parameter for vertical support of stems. According to Veiling Holambra (2019), E. grandiflorum plants need to have, at least, 4.0 mm for SD to ensure adequate support for flowers and proper marketable standards. In this sense, the three mentioned substrates provided stems that met the market requirements, with emphasis on the substrates RRH + S10 and CRH. On the other hand, besides the absence of flowers, the RRH substrate provided inadequate stem diameters, which were much lower than the minimum standard required for commercialization.
For the flowers parameters (Tab. 4), substrates RRH + S10 and CRH presented the highest averages: 3.8 and 3.0 open flowers per stem, flower diameter of 6.6 and 6.2 mm, and 5.1 and 4.1 floral buds, respectively. The substrate CRH + S10 presented lower averages: about 2.5 open flowers, flower diameter of 5.9 mm and 3.6 floral buds. Although this could be unattractive for consumers, stems with two/three buds at the beginning of the floral opening stage are recommended to be harvested. On the other hand, harvesting stems with many open flowers can lead to damage during handling and reduced post-harvest durability.
The substrate had significant effects on E. grandiflorum shoot growth and development since RRH + S10 and CRH provided the highest plant growth (Tab. 2) and more adequate stems in terms of market requirements (Tab. 4).
Although the four substrates presented different DM production in all aerial organs of the plants (Tab. 2), the dry mass distribution (Tab. 3) was similar for the three substrates in which plants presented flower development (RRH + S10, CRH and CRH + S10). In this case, on average, the stems comprised 48.4% of the total DM production, which demonstrated that they were the strongest sinks for assimilates. On the other hand, on average, in the three substrates, the flowers comprised only 18.5% of the total DM production, which demonstrated that they were weak sinks for assimilates regardless of the treatment analyzed.
The cultivation in RRH substrate prevented flower development and modified the dry mass distribution among the plant organs (Tab. 3). The leaves were the strongest sinks for assimilates. Thus, the absence of generative growth caused deep changes in plants grown in this substrate.
Therefore, the RRH substrate did not provide quality compatible with lisianthus cultivation for marketable cut flower since the plants did not grow adequately and did not develop generatively, which resulted in flower absence (Tab. 2 and 3). The plants presented a low leaf area and DM production, which were associated with the lower water holding capacity (Tab. 1) and the low adsorption of nutrient ions of the RRH.
On the other hand, the mixture RRH + S10 benefited root growth and, as a consequence, resulted in a higher PLA and DM production in all aerial organs, including flowers (Tab. 2). Plant leaf area is related to plant growth since leaves are responsible for the interception of the solar radiation that influences photoassimilate accumulation and DM production. Therefore, the highest PLA of the plants grown in RRH + S10 contributed to a greater growth and biomass accumulation (Tab. 2), which resulted in a greater generative growth and stem quality (Tab. 3). Possibly, these results are ascribed to the improvement of physical and chemical properties (Tab. 1) of RRH + S10 substrate provided by the organic compost, as compared to RRH alone.
The S10 addition to RRH increased the easily available water, guaranteed a greater water holding capacity and improved mineral nutrient reserve for the plants. This can be confirmed by the higher values of the first two characteristics and the higher EC observed in this substrate at the end of the crop cycle (Tab. 1). On the other hand, the presence of RRH in the mixture guaranteed good aeration of the root environment. In contrast, the addition of S10 to CRH excessively increased the water holding throughout the plant growth cycle (Tab. 1), which possibly damaged plant growth and quality.
In terms of alteration of the substrate properties after the lisianthus cultivation, a considerable increase in the wet density was observed for all substrates (Tab. 1). The substrate DM increased using RRH and decreased using CRH, CRH + S10 and RRH + S10. The dry density of all substrates increased considerably as a result of the materials accommodation during the growing period.
The total porosity of the four evaluated substrates did not change significantly. However, the aeration space was reduced considerably in CRH, CRH + S10 and RRH + S10, which decreased the aeration levels to a range considered low for horticultural substrates used in recipient cultivation; whereas, the RRH did not affect this parameter. The lower aeration space did not alter the easily available water of the four substrates. The water holding capacity of the RRH + S10, CRH and CRH + S10 substrates increased considerably after the crop cycle, whereas it was not affected in RRH (Tab. 1).
The physical changes of the substrates after the crop cycle were related to the rearrangement of particles and material decomposition during the growing period. RRH alone presented a lower amount of small particles to be rearranged and higher decomposition resistance than the other substrates. Both characteristics led to practically no change in the main physical characteristics of this substrate even after 91 d.
For the changes in chemical properties during the crop cycle, the EC of all substrates increased considerably, which was more expressive in the mixtures containing organic than in the pure materials (Tab. 1). Possibly, the addition of S10 promoted the adsorption and release of ions into the substrate solution throughout the growth cycle, increasing EC to 0.42 dS m-1 for CRH + S10 and 0.33 dS m-1 for RRH + S10 after the lisianthus cultivation. This increase was 0.20 dS m-1 for CRH and 0.14 dS m-1 for RRH.
The pH of all substrates increased. RRH presented a higher pH after cultivation than the others. All substrate pH increases were related to the frequent nutrient solution supply throughout the crop cycle.
Thus, changes in the physical and chemical properties of all substrates were evident. Therefore, more studies evaluating different substrate compositions in recirculating nutrient system on E. grandiflorum production for cut flowers are needed in order to verify which one may be more suitable for plant production.
CONCLUSION
The use of raw rice husk alone as a substrate caused damage to the plant growth and prevented flowering of the E. grandiflorum by modifying the dry mass partitioning among the plant organs.
The mixture raw rice husk + S10 organic compost (RRH + S10) and the carbonized rice husk (CRH) can be used as substrates for E. grandiflorum cut flower production in troughs with leaching recirculation since both provided standard stems for commercialization, especially the former, which promoted greater plant growth.