Introduction
In Northern Australia, leucaena (Leucaena leucocephala ssp. glabrata) is planted in single or double hedgerows several meters apart with a perennial C4 grass planted in the inter-row, and occasionally with a C3 grass in the winter months. This legume-grass pasture system is highly productive for grazing cattle in the >600 mm rainfall zone, with producers reporting greater liveweight gains and profitability compared with other tropical pastures (Shelton and Dalzell 2007; Radrizzani et al. 2010). However, in some regions of Australia leucaena is considered a serious weed, primarily because of the seediness of commercial cultivars, but also because of the wide historic distribution of the non-commercial weedy type L. leucocephala ssp. leucocephala (Shelton et al. 2003; Walton 2003). This weedy non-commercial subspecies has been present in Australia since the late 1800s and pre-dated agricultural production with subspecies glabrata (White 1937). In some regions of Australia, e.g. pastoral lease-hold land in Western Australia and Northern Territory, establishment of commercial leucaena plantations is forbidden due to the perceived environmental weed risk. Consequently, there is a need to develop seedless (sterile) cultivars in order for these regions to share the benefits of leucaena-grass pasture systems.
There are several non-transgenic approaches to developing sterility in leucaena including: cytoplasmic male sterility (CMS) (Saxena and Kumar 2003); interspecific hybridization (e.g. sterile triploids) (Sorensson and Brewbaker 1994); and induced sterility via mutagenesis (Blomstedt et al. 2012). The key to the success of any of these methods will be the ability to produce enough sterile propagules to be viable for commercial use.
Cytoplasmic male sterility
CMS is a genetic system that enables plant breeders to breed hybrid varieties in a range of species, including sunflower, maize, sorghum, rice and pigeon pea to name a few. An advantage of this system is that potentially sterile varieties can be released to producers in the form of seed. To date, no CMS systems have been identified in leucaena, although there have been recent reports of CMS being discovered in another forage legume, pigeon pea (Cajanus cajan) (Saxena and Kumar 2003). This finding in pigeon pea is significant because previously no CMS system had been identified in any legume species. CMS may be developed in leucaena by intercrossing different Leucaena spp. and possibly through mutagenesis.
Interspecific hybridization - Sterile triploids
The genus Leucaena contains diploid and tetraploid species that can potentially be crossed to develop sterile triploid leucaena plants (Sorensson and Brewbaker 1994). Diploid leucaena species, e.g. L. collinsii, L. greggii, L. retusa, L. magnifica and L. macrophylla, are predominantly self-incompatible (SI) meaning that individual trees are self-sterile and require pollen from another tree to set seed. Conversely, many of the tetraploid Leucaena species, e.g. L. leucocephala, L. diversifolia and L. confertiflora, are self-compatible (SC) so that individual trees are self-fertile and do not need to be outcrossed to another individual to set seed. All of the commercial leucaena cultivars grown in Australia today are from the tetraploid species L. leucocephala, and as such are SC. Knowledge of the mating systems can help facilitate the planting of crossing blocks that can be used to develop sterile triploids. This system would require a single genotype of a diploid plant to be cloned and planted in a production field as a female alongside a tetraploid pollinator, e.g. a current commercial cultivar, to be used as the male pollen donor. Seed harvested from the diploid female parent would be sold to producers for commercial use. Alternatively, if sterile triploid seeds can be made by hand crosses, the resultant plants can be multiplied by vegetative propagation and distributed to producers for commercial use. One caveat here is that pollen mentoring has been demonstrated in leucaena seed orchards (University of Queensland tried this approach to make KX2 F1 hybrid seed), which resulted in pure female seed contamination.
Interspecific hybridization - Other
One tetraploid leucaena species that is SI and not SC is L. pallida (Sorensson and Brewbaker 1994) and this species was used as a source of psyllid resistance in several previous research projects with the breeding objective of delivering psyllid-resistant cultivars. During one of such breeding programs to develop psyllid-resistant leucaena, progeny from the interspecific cross Leucaena pallida × L. leucocephala were developed and selected for high IVDMD (in vitro dry matter digestibility), psyllid resistance and self-compatibility. Eventually, breeding line 12 met all the criteria and was released as cv. Redlands. However, of the 40-50 breeding lines that were under consideration at the time, several produced very little seed and were considered shy-seeding. Some earlier generations of these lines were observed to produce no seed. Consequently, these lines are potentially useful for the purpose of breeding sterile leucaena. For these ‘sterile’ plants to be useful on a commercial basis they would need to be propagated clonally. It should be noted that these lines were never conclusively proven to be sterile. They would need to be evaluated in a wide range of environments to make sure photoperiod × temperature interactions did not trigger flowering and seed set outside of the Brisbane environment where the breeding project took place.
Mutagenesis
Inducing sterility by mutagenesis is a relatively easy and direct way of producing sterility in plants by exposing seeds to a mutagen. Ethyl methanesulfonate (EMS) is a chemical mutagen that is often the agent of choice because it has caused high rates of sterility in target plant species (Kurowska et al. 2011). A characteristic of EMS as a mutagen is that it causes single base pair mutations, e.g. GC to AT or AT to GC transitions. While many genetic changes may occur using this mutagen, not all result in a detectable phenotype, and consequently, it is always necessary to check that other characteristics, e.g. quality attributes, have not been altered in candidate plants. In the study reported here we will describe a new methodology for using EMS to create sterile leucaena plants. We have now completed several experiments with EMS applied to seed of the commercial cultivar, Redlands. The objective of these initial experiments was to determine what concentration of EMS would cause an emergence percentage of about 50%, to find a general relationship between EMS treatment and mutation rate and to identify putative sterility in any mutagenized plants. When using mutagens it is generally accepted that some seed death will occur and a 50% emergence rate is generally considered a good compromise between seed death and an adequate mutation rate (Blomstedt et al. 2012).
Materials and Methods
Mutagenesis
Experiment 1. Seeds of cv. Redlands (bred from the interspecific hybrid Leucaena pallida × L. leucocephala) were surface-sterilized using a 30-s rinse with 10% bleach solution before being treated with a chemical mutagen, EMS (ethyl methanesulfonate). Six concentrations of EMS (0.00, 0.10, 0.25, 0.50, 0.75 and 1.00% w/v) and 2 periods of imbibition (16 and 40 hours) were used to treat 300-seed batches of pre-scarified seed. Seeds were scarified with a sharp blade by removing a small piece of the testa at the end opposite from the radicle. For each treatment, seeds were placed in glass beakers set on a gentle rocking platform and completely immersed in 500 ml solutions of the EMS treatment.
Experiment 2. Since the emergence rate of all treated seed in Experiment 1, including the control, was much lower than expected, a second experiment (Experiment 2) was conducted. Seeds were treated on germination paper that had been placed in sterilized germination trays and dampened with the same EMS concentrations used in Experiment 1. Germination trays were kept in the dark for 3 days before the seed was rinsed with deionized water.
Experiment 3. For this experiment, conditions were the same as for Experiment 2 except that the following EMS concentrations were tested to identify the concentration that resulted in a 50% emergence rate: 0.00, 0.25, 0.30, 0.35, 0.40, 0.45 and 0.50% w/v.
Field planting
All germinated seeds (M0 generation) were then sown in small forest tubes filled with a peat mix when the radicle was 2‒10 mm long. (NB. M0 is the first after the mutagen is applied, and seed from M0 plants will be M1 etc.). The seedlings were placed in a shade house until field planting when they were 20‒30 cm tall. Plants of Experiment 1 (108) were sown in the field on 18 December 2017 at Redlands Research Station, Cleveland, Queensland, Australia in rows 1.0 m apart, and with a 0.5 m intra-row spacing. Control plants (0.00% EMS) of cv. Redlands were planted approximately every 30 plants along the row. In addition, 546 M0 plants from Experiment 2 and 605 M0 plants from Experiment 3 were planted at Redlands Research Station on 1 February and 10 April 2018, respectively, using the same procedures described for Experiment 1.
Phenotyping
One hundred and eighty-three surviving plants of Experiment 1 (including controls plants) were phenotyped on 3 occasions (270, 299 and 370 days after field planting) for mutations using a simple phenotypic scoring system. An estimate of mutation rate was calculated by scoring each plant relative to control plants for several attributes, including: flower set (1 = no flowers, 2 = 1‒10 flowers, 3 = 11‒50 flowers and 4 = >50 flowers); pod set (1 = no pods, 2 = 1‒10 pods, 3 = 11‒50 pods and 4 = >50 pods); flower color (1 = white, 2 = not white); plant habit (1 = arboreal, 2 = non-arboreal and 3 = prostrate); vigor (1 = normal and 2 = reduced vigor); and seedling viability (1 = viable and 2 = dead). The mutation rate was considered an underestimate since some single-base mutations at the DNA level would not have resulted in an observably different phenotype and the number of seeds that failed to germinate during the treatment period were not considered for the calculation.
Results
Effects of EMS concentration and period of imbibition on emergence
In Experiment 1, the emergence rate did not exceed 16% for any treatment because the durations of seed imbibition were too long (16 and 40 h). Most seeds rotted in the aqueous solutions of the EMS and the effects increased with the period of imbibition (Figure 1). Therefore, in subsequent experiments, seeds were placed on germination paper moistened with solutions of EMS, making sure that anaerobic conditions were avoided. As a result of the modified protocol, emergence rates in Experiment 2 were as high as 80%. Based on the results of Experiment 2, the target of 50% emergence rate of treated seed was hypothesized to fall between the 0.25 and 0.50% EMS treatments. This was tested in Experiment 3 by including additional rates of EMS within the range of 0.25‒ 0.50%. The combined results of Experiments 2 and 3 are shown in Figure 2 where a curvilinear relationship between EMS concentration and average emergence was observed; 50% emergence occurred at an EMS concentration of 0.35%.
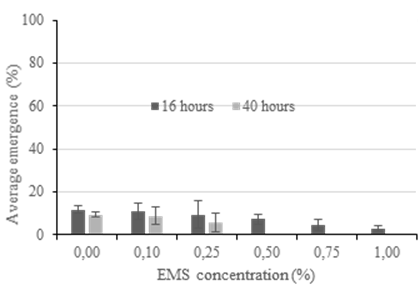
Figure 1 Experiment 1. The effect of increasing ethyl methanesulfonate (EMS) concentration and duration of seed imbibition on average emergence of cv. Redlands. Seeds treated in solutions of EMS. Data shown are means of all reps (n=36).
Effects of EMS treatment on mutation rate
On average, the control plants of cv. Redlands were assigned the following scores: flower set = 4 (>50 flowers); pod set = 4 (>50 pods); flower color = 1 (white); plant habit = 2 (non-arboreal); vigor = 1 (normal); and seedling viability = 1 (viable). Figure 3 shows the relationship between EMS concentration and mutation rate and, not surprisingly, the highest rate of mutation based on assessments of phenotype were induced by 1.00% EMS, the highest rate of EMS used in these experiments. However, the trade-off was that emergence rate of seeds treated with 1.00% EMS was <5% in Experiment 1 and 0% in Experiment 2.
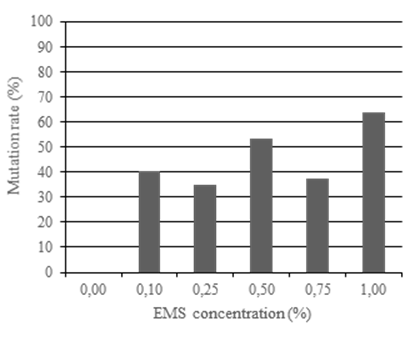
Figure 3 Experiment 1. The effect of increasing ethyl methanesulfonate (EMS) concentration on mutation rate, as measured by observable phenotype. Plants were assessed in a field planting at Redlands Research Station, Cleveland in Autumn-Spring 2018. Data collected from 183 plants including controls (3 plants died).
Effects of EMS treatment on flower and pod set
Based on field assessments made on 3 separate occasions over a 100 day period, each rate of EMS reduced flower and pod set relative to the control treatment (Table 1). An EMS concentration of 1.00% had the greatest effect with reductions in flower set and pod set of 23‒37% and 37‒50%, respectively (Table 1). Twenty-seven putatively sterile plants that had a flower set of 4 (>50 flowers) but failed to produce pods were identified (Table 2). Other plants that flowered profusely but produced only a few pods were also identified. Some plants did not flower during the assessment period.
Table 1 The effect of EMS (ethyl methanesulfonate) concentration on average (a) flower set and (b) pod set on Experiment 1 plants. Plants were assessed over a 100 day period (271‒370 days after field planting, DAP). Traits were scored using a 1‒4 scale as follows: flower set (1 = no flowers, 2 = 1‒10 flowers, 3 = 11‒50 flowers and 4 = >50 flowers); and pod set (1 = no pods, 2 = 1‒10 pods, 3 = 11‒50 pods and 4 = >50 pods). Percentage of flower or pod set, relative to the control plants, is given in parentheses. Planting date was 18-19 December 2017.

Table 2 Pod and seed set scores of 27 putative sterile plants from an EMS (ethyl methanesulfonate) mutagenesis experiment (Experiment 1). The sterile plants were identified from field assessments over a 100 day period (271‒370 days after field planting). Traits were scored using a 1‒4 scale as follows: flower set (1 = no flowers, 2 = 1‒10 flowers, 3 = 11‒50 flowers and 4 = >50 flowers); and pod set (1 = no pods, 2 = 1‒10 pods, 3 = 11‒50 pods and 4 = >50 pods). Planting date was 18-19 December 2017.
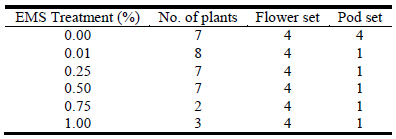
Discussion
A method for mutagenesis of leucaena
A major initiative of this study was to develop sterile leucaena using a mutagenesis approach. We have now made significant progress in developing the methodology required to mutagenize seeds of leucaena. An EMS rate of 0.35% was effective at producing the target germination rate of 50% in a single genotype of leucaena (cv. Redlands). Given cv. Redlands was bred from the inter- specific hybrid L. leucocephala × L. pallida, it cannot be assumed that an EMS rate of 0.35% will be suitable for other cultivars/species of leucaena. Consequently, additional genotypes will need to be tested.
Identification of putative sterile plants
Importantly, after field testing 179 mutagenized plants from Experiment 1, we have identified several putative sterile leucaena plants. Plants that flowered but did not set pods are potentially female sterile. Plants that flowered and set a few pods are potentially male sterile or self-incompatible, with flowers on these plants presumably receiving pollen from neighboring plants, resulting in fertilization and pod set. At this point we are unable to be more definitive about the nature of sterility observed in each of these plants. Male sterility will be investigated by testing the viability of freshly collected pollen grains using standard staining and pollen germination techniques.
Although 179 mutagenized plants were assessed in the current study, Experiments 2 and 3 collectively resulted in over 1,200 mutagenized plants. These will be assessed for further sterile candidates in future seasons when all control plants are flowering profusely. Given the high frequency of putative sterile plants from Experiment 1, we anticipate identifying a large number of target candidates in Experiments 2 and 3.
Once the mode of sterility can be determined it will also be necessary to check if desirable attributes (e.g. high IVDMD, psyllid resistance etc.) of the control genotype (cv. Redlands) have been retained. We were also able to identify plants that failed to flower during the 100-d observation period. It will take several seasons, as well as planting at higher and lower latitudes, to determine if these plants will remain flowerless permanently.
Propagation of sterile plants
One challenge of developing sterile plants is being able to economically multiply any candidate for commercial use. Two approaches are being considered to propagate candidate sterile plants. We have started to investigate methods of vegetative propagation, by dipping the ends of freshly cut branches into solutions of commercial, off-the-shelf root-forming hormone, IBA/NAA.
A second more challenging approach is to develop a CMS (cytoplasmic male sterile) system in leucaena. This system is typically used to make hybrid sunflower, sorghum and rice. However, in the case of sterile leucaena, where seed production is to be avoided, R lines that restore fertility of a CMS female A line are not required. Consequently, it would be sufficient to maintain the A line by crossing with a B line counterpart. In this case, the commercial entity grown by leucaena producers from seed would be a sterile A line. It is possible that some of the sterile plants identified in the present study are CMS. The candidate CMS plants will need to be crossed with candidate B line plants (possibly cv. Redlands) and the resultant seed tested for fertility.