1. Introduction
Filamentous fungi have been cultivated for thousands of years [1]. Industrial and environmental applications of fungi cover areas such as biological control, soil bioremediation [2], enzymes production, and obtaining food [3].
However, in recent decades due to the growing interest in multiple applications and nutritional and pharmaceutical benefits that have been found in the metabolites derived from fungi, scientists have sought biotechnological alternatives, such as submerged cultures, to improve the production of these metabolites and a better control of the production process.
Fungi, according to their morphology, can be divided into filamentous and yeast. The filamentous fungi are characterized by tubular cells called hyphae, which may have divisions (chambered) or not (coenocytic), and whose set is called mycelium. The mycelium can spread over large areas when growing on a solid substrate. However, growth in a liquid culture medium or submerged culture is characterized by the formation of spherical structures called pellets, whose size can vary even presenting a significant effect on the type of metabolites that are generated and depend on the operating conditions under which the submerged culture is performed [4].
In biotechnological crops like mushroom, the operating conditions are very important, as they affect both productionprimary metabolites, vital for the production of biomass, as well as the production of secondary metabolites which are substances of high industrial interest. The pH [5], agitation [4], percentage of dissolved oxygen [6], light intensity [7], and addition of precursors [8] have been some of the variables that have been evaluated in cultured fungi in bioreactors to induce or promote the productionof the metabolite of interest.
In this direction, the objective of this study was to evaluate the effect of operating variables (pH, agitation, and aeration) on the production of biomass and exopolysaccharides from a filamentous fungus of industrial interest in a submerged culture in a 5L bioreactor.
2. Methodology
2.1. Microorganism and culture medium
To carry out this investigation the filamentous fungus Ganoderma lucidum was used. The culture medium used for growing the fungus contained per liter: glucose 35g; peptone 7.5g; yeast extract 2.5 g; KH2PO4 1g; MgSO4.7H2O 0,5g and vitamin B10.05g. The culture medium was sterilized directly in the bioreactor at 120 °C and 15psi for 15 min before being inoculated with the fungus.
2.2. Experimental design
A Box Behnkenexperimental design of response surface was carried out to assess the influence of factors such as agitation, aeration, and pH in the concentration of biomass and exopolysaccharides. Values for each factor levels were selected based on those reported in the literature for the culture of this type of fungus. For agitation,a range from 120 to 200 rpm was evaluated; for aeration a range from 0.5 to 1.5 vvm; and pH between 4.5 and 6.5. Table 1 summarizes the operating conditions of the bioreactor used for carrying out the experimental design. Statistical analysis was performed using the software Design - Expert5®.
2.3. Submerged culture
The experiment was carried out in a 5L bioreactor with a 4L effective work volume. Treatments were evaluated according to the experimental design previously defined and the operating conditions described in Table 1 were maintained. As a strategy to increase biomass production on the third day of fermentation, a pulse of glucose 0.9g/mL was added. The pH was controlled until the sixth day of the fermentation; from this day until the end of the fermentation, only pH monitoring was carried out, control was not performed. Other operating conditions were kept constant in the respective values of the evaluated treatment design. Each culture was monitored for a period of 12 days. 15mL daily samples were collected and stored at 4°C for later analysis of the content of biomass and polysaccharides.
2.4. pH control technique
To evaluate the effect of the bistage pH strategy on biomass production, fungal cultures were performed in two stages. A first stage comprising the first six days of culture, during which the pH control was set at value of 5.5. From the sixth day to the end of the culture no pH control was performed,it was only monitored. Fermentations with pH controlon a value of 5.5 during the 12 days were also performed for establishing the differences in biomass production.
3. Analytical methods
3.1. Quantification of biomass
The sample was divided into three equal volumes to obtain three replicates; A, B, C 4mL each. Each sample was vacuum filtered using qualitative filter paper Boeco65g/m2 previously dried and weighed. The filters with the retained biomass were dried at 60°C until a constant weight was obtained. Finally, to obtain the value of the biomass concentration in g/L, the dry weight (g) obtained for each filter was divided by the volume (L) of each replicate.
From the liquid fraction obtained after filtration, a 1:5 dilution was prepared by adding 4mL of 96% ethanol to 1mL of filtrate sample. Exopolysaccharides were left to precipitate at 4°C for 24 hours. After this period, the mixture was centrifuged at 8500rpm for 30 minutes and the supernatant was taken for determination of substrate consumption by DNS method [9].
The pellet obtained from the centrifugation was washed by adding 80% ethanol in a proportion 1:4 of water:ethanol. Then the mixture is centrifuged again at 8500rpm for 30 minutes. Subsequently, the supernatant was discarded and the pellet re-suspended in 5mL of 1MNaOH for determination of exopolysaccharides using the Dubois method [10].
3.2. Determination of substrate consumption
A 500μL sample of the supernatant obtained after the first centrifugation was placed in a 25 mL volumetric flask and the volume was completed with distilled water to obtain an equivalent dilution of 1:50. From this dilution, 500μL were taken for determining the consumption of substrate using the Determination of Reducing Sugar method following the DNS protocol described by Miller [9].
3.3. Production of exopolysaccharides (EPS)
In order to determine exopolysaccharide production, the methodology described by Dubois [10] was followed. The mixture of pellet and NaOH1M was brought to a water bath at 60°C for about 1 hour with vortex agitation every 15 minutes. Three samples of 0.1 mL were taken from this solution in order to make the replicas of the determinations. Once the treatment to the samples was done, absorbance readings at a wavelength of 492nm were performed.
4. Results and discussion
Table 2 shows the data from Box Behnken experimental design for two response variables: biomass production (g/L) and exopolysaccharide production (EPS g/L), with pH,aeration, (vvm), and agitation (rpm) as factors involved in the process. The results of experimentation for the response variables were normalized by a mathematical transformation that consisted in dividing the values by the volume of medium in the bioreactor.
4.1. Biomass production
Table 3 shows the results for each of the estimated effects and interactions. The standard error of each of these effects is also shown. Fig. 1 presents a Pareto hart for this response variable. In this diagram the main effect of the agitation and pH variables on the production of biomass can be clearly identified. Also, the side effect of the aeration variable. These results are consistent with the results of theANOVA of the experimental design that are presented in Table 4. As it is shown, there is a statistical significance with a confidence interval of 95% (P-value <0.005) for the main factors of pH and agitation with a direct intervention in the production of biomass. In addition, the aeration has a quadratic effect on this variable.
Table 2 Box Behnken experimental design for biomass and exopolysaccharides production.

Source: Own elaboration in Startgraphics®
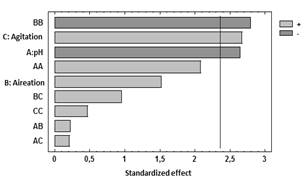
Source: Own elaboration in Startgraphics®
Figure 1 Standardized Pareto chart for the response variable Biomass.
Table 4 Analysis of Variance for Biomass in Submerged Culture.
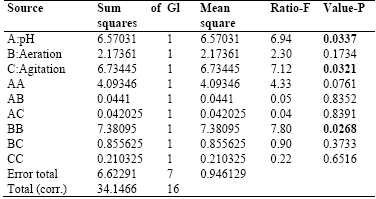
Source: Own elaboration in Startgraphics®
R-square = 80.6045 percent
R-square (adjusted by G. L.) = 55.6674 percent
Standard error of the estimate = 0.972692
Mean absolute error = 0.457529
Durbin-Watson = 1.93958 (P = 0.5466)
Lag 1residual autocorrelation = -0.0290972
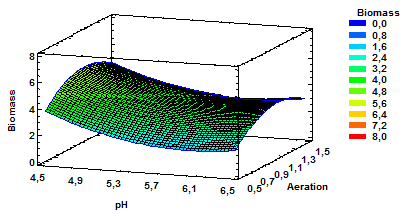
Source: Own elaboration in Startgraphics®
Figure 2 Estimated Response Surface for Biomass with Variable pH and Aeration at a Constant Agitation of 160 rpm.
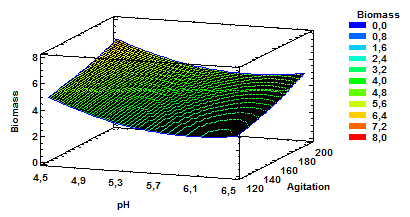
Source: Own elaboration in Startgraphics®
Figure 3 Estimated response surface for biomass with variable pH and agitation at a constant aeration of 1.0 vvm.
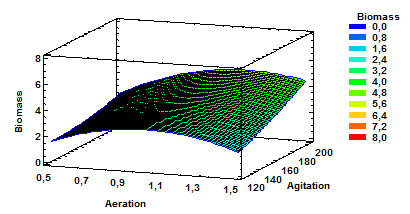
Source: Own elaboration in Startgraphics®
Figure 4 Estimated Response Surface for Biomass with aeration and agitation as variables at a constant pH of 5.5.
R-square statistic indicates that the model, thus adjusted, explains 80.6045% of the variability in biomass in the medium. Statistical adjusted R-square, which is more appropriate to compare models with different numbers of independent variables, is 55.6674%, which shows an acceptable reliability model for predictive purposes. The standard error of the estimate shows that the standard deviation of the residue is 0.972692. The mean absolute error (MAE) of 0.457529 is the average value of the residue. The Durbin-Watson (DW) statistic tests the residue to determine if there is any significant correlation based on the order in which data is presented in the file. Since the P-value is greater than 5.0%, there is no indication of serial autocorrelation in the residue with a significance level of 5.0%.
The results show that the chosen factors do intervene in the response variable biomass in the medium and, therefore, optimization values can be taken into account. Fig. 2 corresponds to the estimated response surface for biomass having as variables pH and aeration with agitation of 160 rpm. As noted, the increased production of biomass is obtained by using combinations of mean values for aeration and lower values for pH within the range evaluated.
The results for estimated response surface for biomass having as variables pH and agitation and constant aeration of 1.0vvm finds are presented in Fig. 3. As shown, the increased production of biomass is obtained with a combination of higher values for agitation and lower values for pH. It can also be established that higher values of agitation at a constant aeration of 1.0 favors biomass production even with higher pH values within the range of values evaluated.
Fig. 4 shows estimated response surface for Biomass having as variables agitation and aeration and constant pH of 5.5. These results confirm that agitation high values combined with mean aeration values or even high values of aeration favor the production of biomass.
The results of the optimization of the response variable biomass in submerged culture of the fungus in 5-liter bioreactor are presented in Table 5. This table shows the combination of levels of factors aeration, agitation and pH within the ranges evaluated, with which the maximum biomass production would be reached. In order to obtain a biomass value of 6.73 g/L in submerged culture, a pH of 4.5, aeration of 1.17, and agitation of 200 rpm should be established.
4.2. Exopolysaccharide production
Table 6 shows the results for each of the estimated effects and interactions and the standard error of each of these effects.
Table 6 . Estimates Effects for the Exopolysaccharides-EPS (g/L)
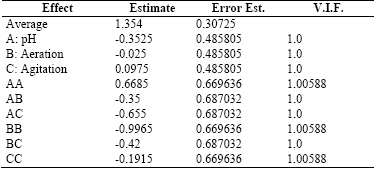
Source: Own elaboration in Startgraphics®
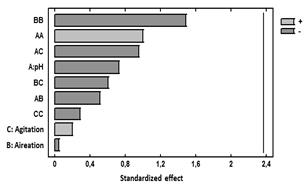
Source: Own elaboration in Startgraphics
Figure 5 Standardized Pareto chart for the response variable Biomass.
Table 7 Analysis of Variance for the response variable Polysaccharides (EPS)
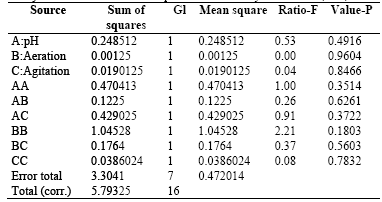
Source: Own elaboration in Startgraphics®
R-square = 42.9664percent
R-square (adjusted by G. L.) = 0.0percent
Standard error of the estimate. = 0.687032
Mean absolute error = 0.388765
Additionally, Fig. 5 presentsa Pareto chart for this response variable. In this diagram, it can be seen that for this variable response there is no significant effect of the main effects agitation, pH, and aeration or side effects. The combination of the levels of these factors in the ranges evaluated does not influence exopolysaccharide production.
ANOVA results obtained for this response variable, which are presented in Table 7, indicate no significant statistical difference (P-value> 0.005) for the treatments performed on the experimental design. This confirms that none of the major factors or combinations of second order intervene in the EPS production in submerged culture of the fungus. R-square statistic indicates that the model, thus adjusted, explains 42.9664% of the variability in Response EPS. Statistical adjusted R-square, which is more appropriate to compare models with different numbers of independent variables, is 0.0%, which invalidates the model for predictive purposes.
However, these results may be related to the experimental difficulties of quantifying the exopolysaccharides in the liquid medium of submerged culture. The complex nature of the matrix that forms in the submerged culture, that becomes much more evident as the time process progresses, leads to the homogeneity of the samples for analysis can vary.
This is mainly due to morphological variation the fungus has during growth. Which in turn generates different phases within the bioreactor and originates a separationof the crop in one or more fractions hindering homogeneous test sample for quantification of the EPS in the liquid culture medium. Fig. 6 shows images of the submerged culture in which it is possible to see the rheological changes that occur in submerged culture of the fungus.
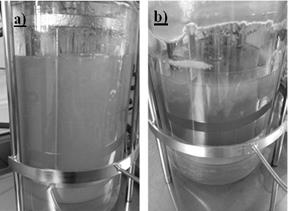
Source: Own elaboration
Figure 6 Images of the submerged culture of Ganoderma l. a) submerged culture on day 4 of fermentation and b) submerged culture on day 12 of fermentation.
Fig. 6a) shows an image from the first days of fermentation. A homogenous culture in which no separation of medium in different phases is observed, there is no separation of biomass or the EPS from the liquid medium and samples that are taken during these days are representative of the entire crop. In Fig. 6b) the change in the rheology of the culture at the end of fermentation can be seen, and a phase separationin the liquid medium in the bioreactor is observed.
Images allow to distinguish a liquid phase at the bottom of the container, while on the surface, a more viscous liquid, gel-like or colloidal, phase that possibly contains part of EPS and mycelium cell produced. Also, at the top of the container, besides liquid medium, a large amount of biomass with adhered gelatinous material that could be part of the EPS generated during cultivation can also be noted.
Considering the rheological change in the medium, achieved in an observational manner, a relationship between polysaccharide production and process variables can be indirectly established. When the culture was conducted using high values of agitation and aeration, an increase in the viscosity of the liquid medium was observed. This change may be indicative of exopolysaccharide production by the fungus to mitigate the effect of agitation and aeration in the bioreactor and reduce hydrodynamic stress [11].
On the other hand, crops with a bistage pH technique may favor, in the first instance, the production of biomass and, then, the production of exopolysaccharides increasing yields of both products in the cultivation [5]. Figs. 7 and 8 show the results of monitoring crops which were carried out under these two strategies, that is, with pH control and bistage pH control.
Fig. 7 shows the kinetics of biomass production for the culture performed with pH control at 5.5 during the 12 days of the process. An approximately exponential growth is observed until day 4 reaching a value of average biomass of 2.02+0.10 g/L. Then the biomass produced remains constant until day 7 when it starts to fall to a value of 1.04+0.13 g/L. From day 9 until the end of cultivation, day 12, the amount of biomass in the crop rises to the same average value achieved in the 4th day.
Fig. 8 shows the kinetics of production of biomass for submerged culture with bistage pH control technique. That is, pH control to a value of 5.5 for the first 6 days of the process and, thereafter, uncontrolled, just recording the changes. As can be seen, for day 6 the production of biomass in the culture with bistage pH control technique was 4.44 + 0.78 g/L equivalent to twice the biomass produced under controlled culture conditions of pH presented in Fig. 7. An important feature of these crops to highlight is that the maximum biomass production didnot show up at the end of the crop in the 12th day, but this maximum value was obtained during the first six days of the process.
It is possible that these results may be related to the formation of cell aggregates or pellets that the fungi form during the first days of culture. Increasing the size of these aggregates was quite significant until day 4. From this day on, cell aggregates (pellets) began to disintegrate and cultivation again became homogeneous. However, increased viscosity is observed at the end. The same effect has been reported by other authors [4, 11], where pellet formation and their size is related to exopolysaccharide production. According to some studies, exopolysaccharides are produced at lower pH values than those used for the production of mycelial biomass [5].
5. Conclusions
Considering the results of analysis of experimental design and optimization performed, it can be concluded that the agitation and pH have a significant effect on biomass production in the cultureof filamentous fungi. The optimum value within the range evaluated for agitation is 200rpm. The best value for pH to maximize production of biomass within the range evaluated corresponds to 4.5. As for aeration, a value of 1.1 vvm has been established as the optimum within the range evaluated. Finally, production EPS may be favored bybistage pH technique strategy as the production of these metabolites is performed at different conditions to those defined for the production of biomass.