1. Introduction
Pectins constitute a heterogeneous group of acidic polysaccharides with properties of gelation, emulsion stabilization, and provision of dietary fiber, which characteristics make these natural products consummately useful in both the food and pharmaceutical industries. The industrial preparation of pectins employs by-products of the production of fruit juices, mainly the rinds of citrus fruits and pomace from apples. The precursor of these soluble polysaccharides is protopectin, operationally defined as the water-insoluble pectic substance that upon partial depolymerization liberates pectins in a soluble form [1,2].
Pectin extraction by conventional methods consists in an acid hydrolysis of the raw material along with heating, a method necessarily involving certain inconveniences-e. g., managing elevated temperatures in the presence of an acid, with that reagent being corrosive to the point of deteriorating the equipment and at the same time generating a contaminant requiring disposal[3]. Because of these inherent difficulties, diverse alternative strategies have been proposed for pectin extraction, including microbiologic or enzymatic methods, with the latter based on the use of protopectinases [3]. With those enzymes, agricultural residues can be exploited through the use of innovative biotechnological treatments entailing a low environmental impact.
The enzymatic extraction of pectin is a classic example of a heterogeneous catalytic reaction. In such systems, a characterization of both the properties of the corresponding enzymes and the composition and structural details of the material used as substrate, take on a special relevance [4]. In contrast to the reactions catalyzed by cellulases and hemicellulases, the heterogeneous reactions catalyzed by pectinases have undergone only very little investigation [5].
The present work was aimed at a deeper understanding of the adsorption of the protopectinase SE (PPase-SE) to the protopectin from lemon (Citrus limon) albedo along with the action of the enzyme in the resulting solubilization of pectin. To that end, we present here the results of a comprehensive study on the action of this enzymatic system with respect to the relevant adsorption features and physicochemical variables of the protopectinase involved in the solubilization of pectin. We also determined the effect of variations in the concentrations of that protopectin substrate and of the enzyme on the initial reaction velocity.
2. Materials and methods
2.1. Chemicals and reagents
Galacturonic acid monohydrate (GA), polygalacturonic acid (PGA), m-hydroxydiphenyl, and the Folin-Ciocalteu reagent were bought from Sigma (St. Louis, MO, USA), Aldrich (Detroit, MI, USA), or Merck (Darmstadt, Germany). All other chemicals and reagents were of analytical grade.
2.2. Source of the protopectinase
PPase-SE was obtained from the fungus Geotrichum klebahnii ATCC 42397 cultured in a 5-L LH-210 bioreactor (Inceltech, France) in fed-batch mode on a medium composed of glucose, urea, salts, and micronutrients [6]. After separation of the biomass by centrifuging (16,000 x g, 10 min, 4 ºC), the resulting supernatant was concentrated by evaporation under reduced pressure in a rotary evaporator, lyophilized, resuspended in deionized water (10X), and frozen for storage at -20 ºC. This solution will hereinafter be referred to as the enzyme concentrate (EC), whose polygalacturonase (PGase) activity was measured at 3,000 units.mL-1 (300 units.mg-1 protein). At the time of use this EC solution was diluted with the buffer and at the pH indicated for each experiment.
2.3. Protopectin preparation
Fresh and ripe lemon fruits (Burm, cv. Lisbon) were selected and kept at 4 °C for 12 hs before protopectin extraction. Preparing of protopectin was done according to Cavalitto et al [5]. Particles retained in 20 mesh (1000 µm > Ø > 850 µm), 35 (850 µm > Ø > 425µm) and 50 (425 µm > Ø > 300 µm) were selected for further studies. Protopectin yield was 35 % (350 mg of protopectin per gram of albedo (on dry basis)). Substrate was kept at room temperature in close containers.
2.4. Analytical measurements
Polygalacturonase activity was assayed at 37 ºC as previously described by measuring the increase in reduced groups by the Somogyi-Nelson method [7]. GA was measured by the m-hydroxydiphenyl method through the use of a standard curve prepared with GA monohydrate (0-150 mg.L-1) treated in the same way as the samples [8]. The ionic strength of the buffers and the protein solutions was assessed by measuring the solution conductivity with a Hanna HI 3291 conductimeter and was expressed in mS.cm-1 (0.1 M KCl, 12.9 mS.cm-1 at 25 °C).
2.5. Adsorption isotherms of PPase-SE to the protopectin from lemon
The effect of particle size (#25, # 35, and #50 mesh) and the nature of the buffer were evaluated. Buffers tested had the following compositions: (a) acetic acid and sodium acetate (AcB, 20 mM), (b) citric acid and sodium citrate (CitB, 10 mM), (c) sodium citrate / sodium phosphate dibasic (CPB [12.5 mM / 6.25 mM]). These buffers-all at a pH of 5.0-had respective electrical conductivities of 1.2, 1.4, and 1.9 mS.
The adsorption isotherms were constructed by adding 10 mL of each buffer to 200 mg of protopectin in a 50-mL Erlenmeyer flask in an ice bath to give a suspension of 20 mg.mL-1 followed by orbital shaking (150 rpm; Centricol Co., Colombia) for 5 min to obtain a complete hydration. Next, all the excess liquid was removed by means of a pipette with a filter cloth on the tip to prevent the passage of particles. The same operation was then repeated nine times with the hydrated protopectin, but each time with the addition of 10 mL of the same buffer containing 25 U.mL-1 of PPase-SE. Extracted samples were stored at -20 ºC until analytical determinations. The amount of enzyme adsorbed by the protopectin, expressed as U.mg-1 of protopectin, was calculated by the difference between the activity added and that remaining in the supernatant buffer after every incubation according to eq. (1).
where AA and AS are the PGase activities added and that remaining in the supernatant, respectively, VA is the volume of the solution, and 200 corresponds to weight in mg of the protopectin used in the assay.
2.6. Physicochemical variables evaluated during the solubilization of pectin
The effect of particle size and buffer composition on the pectin-solubilization reaction, expressed as uronic acids released from the lemon protopectin, was evaluated. Reaction mixture was composed by 30 mg of protopectin and 1.5 mL of buffer with PPase at a concentration of ca. 1.7 U/mg-1 of protopectin (equivalent to 35 U/mL-1). This value corresponds to the concentration that gives the maximum adsorption of PPase-SE to protopectin at 30 ºC. The reaction was carried out for 6 h with shaking at 150 rpm.
2.7. Kinetic study of the action of PPase-SE on lemon protopectin
Lemon protopectin (#35 mesh) at a concentration of 20 mg.mL-1 in CitB (25 mM, pH 5.0) was treated with ca. 34 U.mL-1 of PGase activity in a reaction volume of 1.5 mL for 4 h at 37 ºC at 150 rpm. These conditions were chosen after carrying out preliminary tests involving, first, a range of protopectin concentrations from 2 up to 120 g.L-1, and then, a series of enzyme concentrations at 9, 20, 35, and 50 U.mL-1 with the protopectin concentration held constant at 20 mg.L-1. The initial reaction rates were calculated from the slopes of curves of galacturonic acid (GA) released as a function of reaction time during the first 5 min.
2.8. Determination of the porosity of the protopectin particles
An accurately weighed amount of protopectin (W) was placed in a graduated cylinder and the volume occupied in mL (V1) was recorded. Next, the same volume (V1) of a displacement liquid having no interaction with the particles that might modify their structure was added. Since for this purpose an organic compound is preferably used, particularly a 3- or 4-carbon alcanol; isopropanol was chosen. The total volume of the admixture (V2) is then registered, and with this final value the true density (TD) is calculated according to eq. (2).
In contrast, the observed density (OD) equals W/V1, with the porosity (P) of the particles therefore being eq. (3) [9].
2.9. Scanning electron microscopy
Scanning electron microscopy was performed with a J5M-5910LV, JEOL instrument (Japan) after coating the sample with gold-palladium at a pressure of 40-50 Pa and a voltage of 15 kV.
2.10. Determination of the degree of esterification of pectin by titrimetry
After dissolving 50 mg of pectin in 10 mL of water and adding 2 drops of phenolphthalein indicator, titration is performed with 0.5 M NaOH (V1). Next, in order to produce the complete demetilation of the sample, 1 mL of 0.5 M NaOH is added and left to stand for 15 min. Then, the mixture is neutralized with the same volume of 0.5 M HCl along with vigorous shaking. Finally, the titration is repeated with the same concentration of NaOH (V2). The degree of esterification (DE), as a percent, is finally determined by eq. (4).
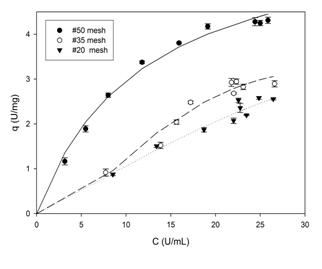
Source: The Authors
Figure. 1 Adsorption isotherms of PPase-SE to lemon protopectin in AcB (20 mM, pH 5.0) at 4-8 ºC with shaking at 150 rpm. The concentrations of enzyme and substrate were 25 U.mL-1 and 20.mg.mL-1, respectively.
3. Results
3.1. Adsorption isotherms of PPase-SE with lemon protopectin
The processes of adsorption forms an essential part of heterogeneous catalysis and depend on both the structure of the adsorbent particle and the composition of the reaction medium in addition to other operational variables such as pH and temperature. As mentioned above, the effect of buffer composition and particle size were studied. All the enzyme assays were performed at temperatures of 4-8 ºC in order to diminish the initial velocities of the catalysis.
3.1.1. Adsorption isotherms in AcB (20 mM, pH 5.0)
Fig. 1 shows the adsorption isotherms of PPase-SE to lemon protopectin in AcB. As can be seen, with particles of #50 mesh the system behaves according to the model of Langmuir, as described by EQ. (5).
where q is the activity of the enzyme adsorbed by the protopectin (in U.mg-1 of protopectin), C is the activity of the enzyme at equilibrium (in U.mL-1), qm is the maximal enzyme-adsorption capacity of the protopectin (in U.mg-1 of protopectin), and Kd is the dissociation equilibrium constant for the adsorption process.
The parameters of the model, for these particles of #50 mesh size, were calculated by means of a nonlinear regression through the use of the program Sigma Plot (SYSTAT Software Inc.) and were: qm = 6.5 ± 0.7 (21.5 mg of protein.g-1 of protopectin) and Kd = 11.9 ± 3.0, with an R2 = 0.98.
Comparatively, the results of studies on the adsorption of cellulase to a microcrystalline cellulose material of undisclosed mesh size indicated a Kd of close to 4 mg of protein.mL-1 and a qm value of 150 mg of protein.g-1 of cellulose [4]; while depending on the specific type of cellulose adsorbent used and the pretreatment conditions, Kd values of 0.19 a 16.7 mg protein.m-1 were reported for the cellulase of Trichoderma reesei[10]. In addition, with a cellulase from Trichoderma zongibrachiatum and a commercial cellulose, values for qm of between 0.38 and 69 mg of protein.g-1 of cellulose have also been indicated [11]. In contrast, no data are available in the literature on equilibrium constants for the PPase-protopectin system, but the value obtained here at least in comparison to the reports for the interaction between cellulase and cellulose-would suggest a high affinity of this enzyme for its substrate.
Despite the low temperatures at which the adsorption was carried out (4-8 ºC), the enzymatic reaction intractably continued with the end result that throughout the duration of all the adsorption assays the presence of solubilized pectin was always detectable. Likewise, other authors have described high levels of cellulose hydrolysis through the action of cellulase at both 4 ºC and 50 ºC [10].
The isotherms obtained with protopectin particles of mesh sizes #35 and #20-with respective diameters greater than 0.43 and 0.85 mm (Fig. 1)-have inflection points associated with changes in the concavity of the parametric function, which shifts to convexity occur in the description of processes of multilayered adsorption. The latter are not contemplated in the Langmuir model and, for their part, explain the discrepancies with that model observed here. Nevertheless, the particles of #50 mesh size-with diameters greater than 0.30-exhibited a greater PPase-SE-adsorption capacity in accordance with the greater surface-to-volume ratio of those particles of smaller diameter; with this behavior constituting another characteristic of heterogeneous catalysis [4.11.12].
The scanning-electron photomicrographs in Fig. 2 illustrate the structure of the particles of mesh sizes #50 and #35; average size, amorphic structure and high degree of porosity. That feature was determined experimentally for the particles of both of these mesh numbers through the approximate method of volume displacement and proved to be comparable between the two size classes at 0.81, which result is consistent with the notion that the size of the particles, and not their porosity is the principal governing influence on the adsorption capacity of the protopectin for the PPase-SE enzyme.
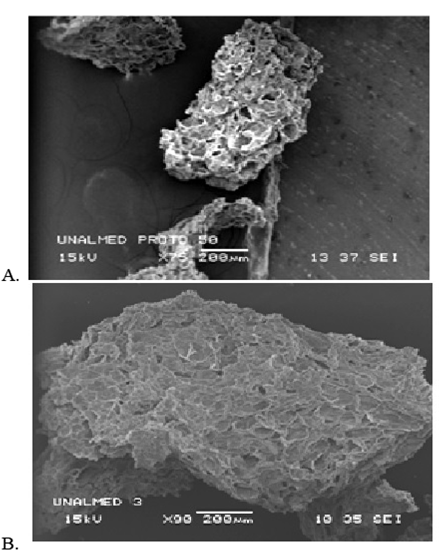
Source: The Authors
Figure. 2 Scanning-electron photomicrographs of lemon protopectin particles: (A) mesh #50 particles, X75; (B) mesh #35 particles.
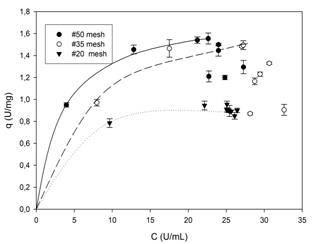
Source: The Authors
Figure. 3 Adsorption isotherms of PPase-SE to lemon protopectin in CitB (10 mM, pH 5.0) at 4-8 ºC with shaking at 150 rpm. The concentrations of enzyme and substrate were 25 U.mL-1 and 20.mg.mL-1, respectively.
3.1.2. Adsorption isotherms in CitB (10 mM, pH 5.0)
The adsorption kinetics of PPase-SE in Cit B (showed in Fig. 3) were similar between the particles of mesh size #50 and #35 both in terms of the maximum amount of enzyme adsorbed and with respect to the evidence of an initial adsorption (represented by lines) followed by a process of desorption.
The desorption portion of the curve exhibits kinetics that reflect the Langmuir model adequately with parameters of qm = 18 ± 0.1 (6.0 mg protein.g-1 of protopectin) and Kd = 3.5 ± 0.8 (R2 = 0.99) for mesh-#50 particles and of qm = 1.9 ± 0.5 (6.1 mg protein.g-1 of protopectin) and Kd= 7.2 ± 4.5 (R2 = 0.96) for mesh-#35 particles. The immediate adsorption observed with the mesh-#20 particles impedes an adequate modelling of the system since kinetic measurements are impossible under those conditions. Furthermore, the data for the mesh-#35 particles show a large variation (ca. 63%) owing to the scant number of points as a result of the rapid adsorption of the enzyme.
A comparison of the values of qm for the mesh-#50 particles in the presence of 10 mMCitBversus 20 mMAcB reveals that the latter buffer facilitates a greater adsorption. This difference could be explained in the following three ways: (1) At pH 5.0 the citrate ion has two negative charges, whereas the acetate has only one. (2) Application of the Henderson-Hasselbalch equation indicates that a greater quantity of negative species is present in CitB than in AcB. (3) The citrate ion is a larger molecule than the acetate. Any one of these three differences, or a combination of them, could account for the greater interaction of the citrate ion with the enzyme-it having a residual positive charge-thus reducing that protein's affinity for adsorption to the protopectin surface, with the latter, for its part, possessing a negative charge at the experimental pH.
Other reports have indicated that in cellulose-cellulase systems a comparable adsorption-desorption dynamic also occurs [12] in keeping with the profiles of Fig. 3. With either system, such kinetics can be explained in two possible ways: (1) CitB effects a greater solubilization of pectin (between 450 and 1,100 mg.L-1 of GA depending on the particle size at each point of the adsorption isotherm) than AcB (between 300 and 450 mg.L-1 of GA with the same dependencies), thus producing at the same time a more rapid disintegration of the substrate particle to create a greater total area of reactive surface and a more rapid binding of the enzyme and subsequent release for further reaction elsewhere. (2) The enzymatic catalysis operates exclusively on an amorphically structured surface of the particle, which portion through the catalyic action then becomes crystalline, as suggested by other authors [10,13] and also observed in these experiments (cf. Fig. 4).
3.1.3. Adsorption isotherms in PCB (6.25 mM, pH 5.0)
Fig. 5 shows the adsorption isotherms of PPase-SE to lemon protopectin in PCB. As can be seen, the #50-mesh particles exhibit the same kinetics within the region of adsorption as seen previously in CitB and AcB. That kinetics-consisting in interactions between the enzyme and the buffer components-define in large part the maximum adsorption capacity of the protopectinase for the enzyme. In this buffer, that peak adsorption value of 2.5 U.mg-1of protopectin was intermediate between the figures obtained with the other two buffers.
The explanation for this result is based on the capacity that the dibasic phosphate ion has, with its single negative charge, of exchanging a proton with the citrate ion, thus neutralizing one of its two negative charges. After that step, the resultant degree of interaction between the two ionic species and the enzyme would be less than is otherwise possible in the buffer with the citrate alone.
The desorption stage in this buffer is similar to that seen in CitB, and the causes of those kinetics are likewise attributable to the same structural effects as cited for that buffer-namely, the progressive solubilization of pectin with time.
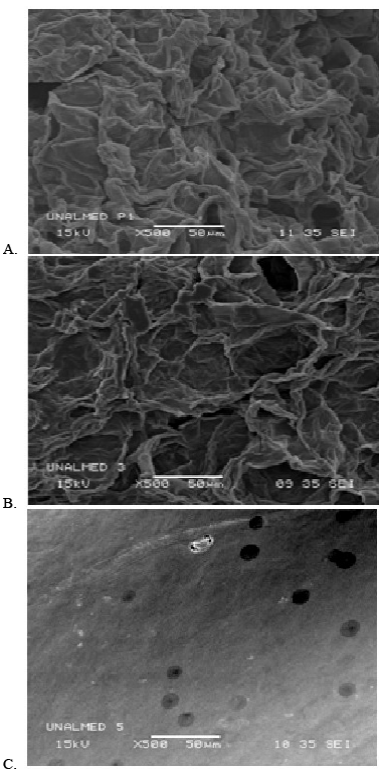
Source: The Authors
Figure 4. Scanning-electron photomicrographs of lemon protopectin mesh #35 particles. (A) Amorphous structure before treatment. (B) Amorphous structure after exposure to CitB (10 mM, pH 5.0). (C) Crystalline structure after the action of PPase-SE (30 U.mL-1) in CitB (10 mM, pH 5.0). Incubation was at 4-8 ºC with shaking at 150 rpm.
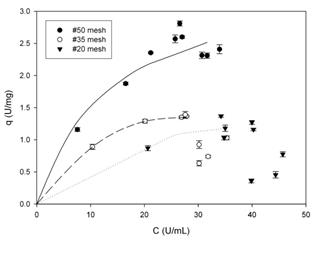
Source: The Authors
Figure. 5 Adsorption isotherms of PPase-SE to lemon protopectin in PCB at 4-8 ºC with shaking at 150 rpm. The concentrations of enzyme and substrate were 25 U.mL-1 and 20 mg.mL-1, respectively.
3.2. Effect of particle size and buffer composition on pectin solubilization
Fig. 6 summarizes the effects of particle size (#50- and #35-mesh particles) and the nature of the buffer on the solubilization of pectin at pH 5.0. As can be see, particle size has no significant effect in pectin solubilization. For a given particle size, the buffers CitB and PCB, however, more greatly facilitated the solubilization-and there to an equivalent extent between the two-than did the AcB. All of these statistical comparisons were mediated by the Student t test (at a significance level of p<0.05). Since the best solubilization was achieved with either of the two buffers CitB and PCB, CitB was selected as the buffer to be used with particles of #35 mesh size (>0.43 mm).
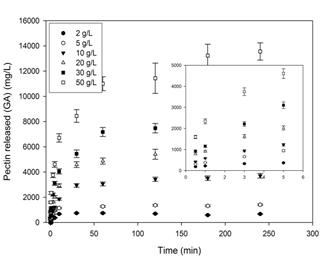
Source: The Authors
Figure. 7 Effect of the concentration of lemon protopectin (mesh #35 particles) on the solubilization of pectin. The particles were incubated for the duration of time indicated on the abscissa with 35 U.mL-1 of PPase-SE in CitB (25 mM, pH 5.0) at 37 ºC with shaking at 150 rpm.
This difference between CitB or PCB and AcB could be attributed to the ability of the citric acid to chelate divalent ions, especially Ca2+, that constitute part of the structure of protopectin and that are responsible for maintaining the linear chains of homogalacturonate, thus conferring a structural rigidity on the molecule and favoring its insolubility. By such chelation, citrate would promote the separation of the homogalacturonyl chains with a consequent solubilization of the pectin [14,15]. Various authors have utilized ethylenediaminetetraacetic acid for the same purpose [16,17]. In this regard, synergistic effects between enzymes and certain organic acids on the extraction of pectin have also been reported. The degree of esterification of pectin that was measured here was 78 an extent indicating a high level of methoxyl groups.
3.3. Kinetic study on the solubilization of pectin in the protopectin-PPase-SE system
3.3.1. Effect of protopectin concentration
Fig. 7 shows the concentration of pectin released as a function of time at different initial concentrations of the protopectin substrate. The kinetics are characterized by an initial linear segment during the first 5 min followed by a progressive plateauing thereafter. The curves become horizontal with the solubilization sooner at the lower concentrations of the substrate.
Similar results were reported by different authors for the cellulose-cellulase system [13,18,19]. Furthermore, as mentioned above in the discussion of the kinetics of the adsorption isotherms, the conversion of the protopectin surface to the crystalline state upon the action of PPase-SE hampers the further hydrolytic process since the enzyme readily degrades only the amorphous portion of the substrate and is far less accessible to the crystalline regions [13]. In addition, this plateauing has been previously attributed to a thermal instability of the enzyme, though such an explanation would hardly apply in this instance since the hydrolyses were carried out at 37 ºC [20].
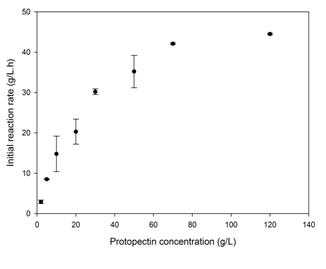
Source: The Authors
Figure. 8 Effect of the concentration of lemon protopectin (mesh #35 particles) on the initial velocity of the hydrolysis reaction. Concentration of PPase-SE, 35 U.mL-1 in CitB (25 mM, pH 5.0); incubations at 37 ºC with shaking at 150 rpm.
Fig. 8 depicts the effect of protopectin concentration on the initial rate of the hydrolysis reaction. Higher concentrations of the substrate than shown in Fig. 7 (i. e., 70 and 120 g.L-1) were also investigated in order to extend the graph further. These kinetics conformed satisfactorily with the Michaelis-Menten model exemplified by Eq. (6).
wherevo is the initial velocity of the reaction in g.L-1.h-1, vm the maximum initial velocity, and KM the Michaelis-Menten reaction velocity constant in g.L-1.
The corresponding parameters obtained here were vm = 57.3 ± 5.6 g.L-1.h-1 and KM = 30.2 ± 7.7 g.L-1. An analysis of variance confirmed the correctness of fit of these data to the Michaelis-Menten model at an uncertainty level of p<0.05 and an R2 correlation of 0.99.
This kinetics was also cited by other authors for the cellulose-cellulase heterogeneous-phase enzymic-catalysis systems [10,12,13]. Other reports in the literature, for a suspension of 50 g.L-1 of cellulose in CitB (50 mM, pH 4.8), gave values of vo at 9.1 g.l-1.h-1 along with a vm = 13.0 g.L-1.h-1 and a KM = 22.8 g.L-1 [10]. Even though these comparisons deal with a different system from the one investigated here, nevertheless the order of magnitude of the values between the two bears a significant similarity.
3.3.2. Effect of PPase-SE concentration
Fig. 9 shows the solubilization of pectin as a function of the concentration of PPase-SE, with that process exhibiting a typical saturation-limited profile; but here the concentration of the enzyme had no effect on the vo, whose value was 24.8 ± 2.2 g/L.h.
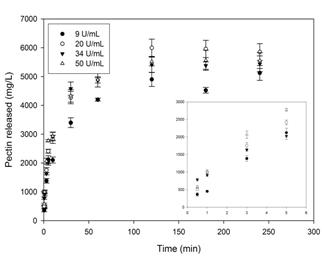
Source: The Authors
Figure. 9 Effect of the concentration of PPase-SE at 35 U.mL-1 in CitB (25 mM, pH 5.0) on the solubilization of pectin from lemon protopectin (mesh #35 particles) at 37 ºC with shaking at 150 rpm.
That the reaction kinetics for the solubilization of pectin from protopectin are independent of the concentration of enzyme-in contrast to the circumstance with homogeneous enzymic systems-demonstrates that in this instance the area of the substrate exposed to the action of the enzyme, and not the amount of the latter, determines the kinetic parameters.
4. Conclusions
On the basis of the findings described here, we can construct a model for the enzymatic extraction of pectin through hydrolysis by PPase-SE. We have shown that the binding of the enzyme to the protopectin substrate occurs in accordance with the model of Langmuir for small-sized particles, though an increase in particle size can generate deviations from that model that suggest a multilayered adsorption. Nevertheless, the structural zones of the protopectin that are altered through the enzymic action produces processes of desorption; and therefore both the area of the substrate available and the nature of the structure exposed constitute the limiting entities in the catalysis. Furthermore, within the reaction system, buffers that have the capacity to chelate divalent metals (e. g., CitB and PCB) promote the solubilization of pectin through the binding of the Ca+2 ions that are essential for the stabilization of the protopectin structure.