1. Introduction
Guava (Psidium Guajava L.) is a fruit that is characterized by its high pectin, mineral, vitamin, and essential amino acid content. Due to its high rate of respiration and high ethylene production during ripening, it is considered to be a climacteric fruit. [1] In addition, guava is classified as a perishable fruit due to the enzymes involved in the physiological and biochemical changes of senescence [2]; thus, limiting their shelf life.
In Colombia; guava fruit is primarily grown in the departments of Boyacá and Santander. Due to its economic importance which generates the primary income for around 9000 families [3,4]; thus losses due to accelerated ripening processes and inadequate post-harvest practices need to be minimized. [4,3].
The national fruit plan, together with the association of guava agro-producers and the local government, estimate an average post-harvest loss of 10% due to these factors. [5] This situation causes a negative socioeconomic impact at a departmental and national level. [6]
Various technologies have been developed to extend shelf life and maintain post-harvest quality of fruits; however, these treatments have limitations regarding long storage times, [7] high operation and maintenance costs, [8] alterations in the organoleptic properties of fruits, [9] and the presence of chemical residues within them. [10] These problems indicate a need to develop alternative treatments to prolong the shelf life of the fruit. Among these treatments is the application of maturation retardants such as citral (C10H16O). [11-12] This retardant is also beneficial due to its antimicrobial [13] and antifungal effects [14-15] as well as acting as an inhibitor for the biosynthesis of ethylene, [16] a transcendental metabolite in the fruit ripening process. [17-18].
The implementation of micro and nano-sized structures for the release of specific compounds, [19] has been developed for applications in the pharmaceutical and food products. Among these systems are polydiacetylene nanostructures such as liposomes, which have the advantage of mimicking cellular functions and offer a controlled release mechanism. Through the change of an external stimulus such as an increase in temperature, the fluidity of the lipid bilayer is altered. [20] These nanostructures can therefore be used as encapsulants due to their ability to maintain the chemical stability of the encapsulated compounds.
The current study aims to combine the encapsulating capacity of the liposomal nanostructures with maturation retardant; therefore, achieving the controlled release of citral in guava fruit (Psidium Guajava L.) from a local farm in Moniquirá, Boyacá (Colombia).
2. Materials and methods
2.1. Instruments y equipment
IKA RV 10 digital Rotary Evaporator. Cole Parker 8891.bx ultrasonic bath. Fisher Scientific, Fisherbrand Q500 ultrasonic probe. Entela model PS1 mercury lamp. Cary 50 UV spectrophotometer, VARIAN with optical path of 1 cm, spectral slit width of 1 nm, Czerny-Turner monochromator, and UV-Vis resolution limit of ≤ 1.5 (nm). Thermo Fisher Scientific GENESYS 10 UV-Vis spectrophotometer with optical path of 1 cm, spectral slit width of 1 nm used in the range of 190-1100nm. IKA series C-MAG hot plate. LSD Dyna Pro NanoStar, Wyatt Technology Corp using 1 cm cuvettes with a 2000 mL capacity and a dispersion angle of 90 degrees and a temperature of 25ºC. BRIXCO 3050 refractometer using a 0-50% scale.
2.2. Chemical reagents
10,12-Pentacosadiynoic acid (PCDA), from GFS, Inc. Soy Lecithin 16% from Calbiotech. Dichloromethane from Fisher Scientific. Sodium Hydroxide 98%, Sodium Chloride, Potassium Chloride, and 2-Nitroaniline from Merck. Citral, β-Cyclodextrin (β-CD), Methanol, Ethanol, Dichloromethane, Sodium Phosphate, and Potassium Phosphate, Oxalic Acid, and Ascorbic Acid from Sigma Aldrich. Cellulose Ester Membrane (MWCO: 14,000) from Spectra/Por® Biotech. Polycarbonate membrane with 1 µm pore size from Sartorius Stedim Biotech.
2.3. Obtaining the fruits
Fresh guava fruit (red variety) was collected and manually selected from the Las Rocas farm in Moniquirá, Boyacá. Then, they were stored in aerated plastic boxes and moved to the Environmental Chemistry Laboratory (GIQUA) at the Universidad Pedagógica y Tecnológica de Colombia in Tunja. Once in the laboratory the fruits were selected and separated according to the degree of maturity.
2.4. Synthesis and characterization of citral nanocontainers
The citral nanocontainers were synthesized using the thin layer dehydration method from a mixture of PCDA and Soy Lecithin. This mixture was dissolved in dichloromethane with a molar ratio of 80:20 and a total concentration of 1mM. The solution was subjected to rotary evaporation under controlled conditions to promote the formation of a thin layer and dried under vacuum for 12 h. It was then rehydrated by the addition of 25 mL of 2 mM Citral solution. [21] The dispersed solution obtained was immersed in an ultrasound bath for 15 min and homogenized by ultrasonic probe at 75 °C, 25% amplitude, and a pulse cycle of 5:1 seconds on:off for 15 min. [22] The dispersed solution was then passed through a polycarbonate membrane in order to remove any lipid aggregate.[23] Finally, the dispersed solution of liposomal nanocontainers was refrigerated for 8 h, at 4 °C and protected from light.
The free citral was removed from the liposomal nanocontainers through dialysis with a cellulose ester membrane in an aqueous medium for 48 h with water changes every 4 h. Prior to use the liposomal nanocontainers were polymerized using UV radiation at 254 nm, for 2 min at 4 °C. [22]
The dialyzed and polymerized liposomal nanocontainers of PDA:Lecithin, were characterized using UV-Vis spectroscopy scanning in the 400-800 nm range with a scanning speed of 0.5s. The samples were analyzed before and after being exposed to a thermal stimulus of 50ºC with distilled water being used as a blank.
2.5. Characterization of the citral nanocontainers
The formation of the β -Cyclodextrin-Citral complex (β-CD-Citral) was identified by UV-Vis spectroscopy, at ~240 nm. To obtain the complex, 1g of β -CD was dissolved in 11 mL of a methanolic solution (2:1, v/v) and then heated to 55 °C. The citral solution was then slowly added and stirred for 4 h until it reached room temperature. The solution was then dried at 30 °C in a drying oven for 8 h, then, stored in a desiccator for 12 h. Finally, the complex was dissolved in 10 mL of distilled water and its spectrum was recorded.
2.6. Evaluation of temperature as a mechanism for the release of citral
A thermal stimulus was applied from an initial temperature of 15 ºC to 50 ºC in increments of 5 ºC to 2 mL of the suspension nanocontainers containing PDA:Lecithin encapsulating citral. The transformation of PDA was analyzed using UV-Vis spectroscopy in the range of 400-800 nm. The colorimetric transformation of PDA [24] in the nanocontainers was evaluated through the colorimetric response (% RC); between the initial phase (blue) and the final phase (red) as shown in equation 1. As a control, nanocontainers without a maturation retardant were used.
Where PB0 = Ablue/ (Ablue+ Ared). PB0y PBicorresponds to the relationship in maximum absorbance between the blue band (Ablue, at 650 nm) and the red band (Ared, at 540 nm) presented by the sample without a stimulus (PB0) and with stimulus (PBi).
2.7. Evaluation of the effect of citral nanocontainers onguava ripening
The guava fruit which with green in appearance according to the control letter, were divided into 4 treatment groups of 10. [25] The groups were as follows: as a control guava without any treatment (BV), guava with citral nanocontainers (L+CV), guava treated with liposomal nanocontainers (LV), and guava treated with citral solution (CV). Treatment was applied by spraying 2 mL of solution per fruit. The fruit was then dried at room temperature and stored in an aerated wooden box for 15 days. Every third day the percentage of weight loss, color, total soluble solids, titratable acidity, ripening index, and vitamin C content were measured.
Percentage of weight loss was estimated by gravimetric by measuring the difference in daily weight using an analytical balance.
Color was determined through the photographic comparison of 2 fruits per treatment with a color chart of guava fruits. [26]
Total soluble solids (%SST) were determined by refractometry. According to AOAC 932.12/90. The data are reported in Brix according to the guideline NTC 4624 of 1999. [27]
Percentage of titratable acidity (%H) was determined by the AOAC method 942.05/90 on fruit extract (10% w/v). The results were expressed as percentage of citric acid according to NTC 4623 of 1999. [28]
From the relation between SST and % acidity, a ripening index [29] percentage was determined with the equation 2, which indicates:
Vitamin C was evaluated using the colorimetric method of 2-Nitroaniline. [30] The extract was obtained of 1 g of fruit sample and, of this extract was taken three samples. Next, each sample was dissolved in an oxalic acid solution (0.15% w/v) and filtered.
0.1 mL of 2-Nitroniline solution was added to a test tube followed by 0.2 mL of sodium nitrite. The solution was then stirred until a yellow discoloration of 2-nitroaniline was achieved. 3.8 mL of ethanol was then added followed by 0.5 mL of test extract and 0.1 mL of oxalic acid. The entire solution was then mixed and left to sit at room temperature for 5 min. Then, 1.2 mL of sodium hydroxide (10% w/v) was added with an additional 1 mL of distilled water and the absorbance measured at 540 nm. The calibration curve was created with ascorbic acid, at concentrations of 0.02-0.1 mg/L.
An ANOVA analysis of variance was performed using the results obtained in order to determine if there were any statistically significant differences for any of the treatments. Additionally, t-tests were performed to evaluate the data obtained from the physicochemical analysis.
3. Results
3.1. Synthesis of PDA: Lecithin nanocontainers encapsulating citral
The synthesis of nanocontainers was performed using the self-assembly of lipids and monomers in lipid bilayers. This occurs due to the hydrophobic effect and electrostatic interactions which are characteristic of long amphoteric carbonate chains in aqueous mediums. When energy is supplied by ultrasound the chains organized in lipid bilauers can close in on themselves. [31] Topo-polymerization of the liposomal nanocontainers were triggered by ultraviolet radiation, [32-33]and the colorless solution turned blue.
The UV-Vis spectrum at 15ºC and 50ºC as shown in Fig. 1, confirms the formation of the liposomal PDA nanocontainers. The characteristic absorption band of the PDA was identified at a wavelength of 656 nm. This band is attributed to the π-π* transition of the polymer conjugation of eno-ino bonds; the blue phase of the PDA in a planar structure. Additionally, this absorption band has a characteristic shoulder band at ≈ 590 nm which corresponds to the vibrational structure of the polydiacetylene polymer. [34] Report that the mixture of 86% phosphocholine and PDA present the characteristic spectrum of PDA. [35] This therefore confirms that the components of the nanocontainers self-assembly are in a defined spatial structure originating in the blue phase (solid line), which is characteristic of PDA.
The materials based on PDA recently polymerized exposure to a thermal stimulus show characteristic bands in the UV-Vis spectrum. Increasing the temperature of the liposome dispersion to 50 °C, exhibits a change in the spectrum absorption band of 656 nm (blue phase) shifted to 640 nm. This shift is also coupled with an approximate decrease of 40% in the intensity of this band, fig1 (dashed line). The presence of an additional signal at 540 nm called the red phase is also observed with a shoulder band at around ≈500 nm. [36] This band is attributed to the PDA side chain, which is altered in the presence of physical or chemical agents and by the release of tension on the side chains of the polymer conjugate chain. The flat structure becomes a contorted structure, generating changes in the relocation of the orbitals. [37]
Fig. 2 presents the UV-Vis spectrum which is characteristic of citral presenting a band at a wavelength of 244 nm while the spectrum of B-CD lacks absorption bands. The spectrum of the citral nanocontainers after a thermal stimulus of 50ºC, presented a characteristic band at 540 nm. The encapsulation of citral was confirmed by the formation of the B-CD-Citral complex which was produced when it was released from the liposomal nanocontainers at 50 °C indicated by a band at 230 nm as being the product of a reaction between released citral and β-CD. The difference in absorption can be associated with the overlap of the π orbitals of the geometric isomers of citral. [38].
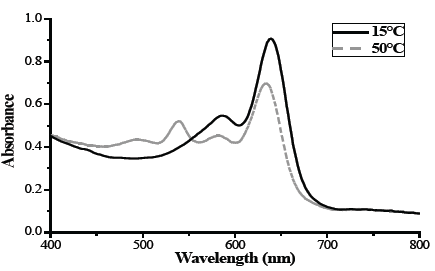
Source: The Authors.
Figure 1 Characterization of the nanocontainers by UV-Vis spectrophotometry at 15 and 50 °C.
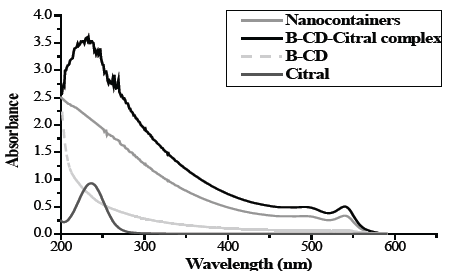
Source: The Authors.
Figure 2 UV-Vis absorption spectrum of a) Citral, b) β-CD, c) β-CD-Citral complex, d) Citral nanocontainers.
The size of the citral liposomal nanocontainers was evaluated by DLS. In Fig. 3, nanocontainers were determined to have an average radius of 352 nm for 76.2% of the mass and 92.5 nm for 23.8% of the mass. A general average in size of 212 ± 10 nm was established. The above values indicate that the size of the liposomal citral nanocontainers vary widely as a result of the synthesis of this compound using the ultrasound method. [39]
Additionally, the sample presented lipid aggregates due to the amount of time needed to measure the sample. [40]
3.2. Evaluation of temperature as a mechanism for the release of citral
Guava fruit grow at Environment temperature in the range near to 20-32 °C, in the farms to near to Suarez River. [41] This provides an opportunity to measure the effect of temperature on the transformation of the PDA polymer from the blue phase to the red phase. This change in phases allows the membrane greater fluidity, helping to release its content. Fig. 4 presents the UV-Vis spectra of the liposomal nanocontainer of PDA: Lecithin at different temperatures. The initial absorption spectrum at room temperature, 15 ± 1°C presents the characteristic absorption band of PDA. As the temperature is gradually increased by 5 degrees to reach 50 °C, the absorption band at 654nm decreases in intensity and the maximum wavelength is red shifted. In addition, the presence of a new band at 550 nm is observed at 35 ºC. [42]
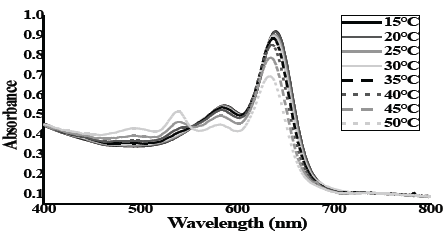
Source: The Authors.
Figure 4 UV-Vis absorption spectra of nanocontainers PDA: Lecithin subjected to heat treatment.
At ≈560 nm an isosbestic point is shown which confirms the simultaneous existence of two different isomers and the transformation of one phase into another due to an increase in temperature. [43] An increase in temperature of the solution containing the liposomal nanocontainers of PDA causes an increase in the dynamic behavior of the side chains. [44-45] This results in altered conformations in the packing and orientation of the main chain which create spaces in the bilayer that facilitate the transport of internal content within the liposome towards the outside.
The changes in side chains are associated with the %RC value in Fig. 5. Since the packing of the side chains is affected by temperature changes, it is expected that the citral molecules encapsulated in the liposomal nanocontainers can access the spaces created in the lipid bilayer and be released as a consequence of the chemical gradient.
The direct influence of temperature on the structure of the lipid bilayer of the nanocontainers and its release of citral can be observed. In the Suarez River Region, a maximum temperature of 32ºC is recorded, which according to the colorimetric response for that temperature range will result in an average of RC of 3%. It can then be inferred that the temperature is hot enough to allow the release of citral from the nanocontainers. Then, this agent will act on the fruit preventing the ripening.
The direct influence of temperature on the structure of the lipid bilayer of the nanocontainers and its release of citral can be observed. In the Suarez River Region, a maximum temperature of 32ºC and, according to the colorimetric response for that temperature results from an average of RC of 3%. It can then be inferred that the increase of temperature is to allow the release of citral from the nanocontainers. Due to an increase in the dynamic behavior of the side chains and, as a result, a modification in the main chain allowing the release of citral. Therefore, this agent acts on the fruit preventing the ripening.
3.3. Evaluation of the effect of citral nanocontainers on the guava ripening
The effect of nano-encapsulated retardant agents on guava fruits of the red variety was evaluated at a laboratory scale. An analysis of the following physical-chemical properties: weight loss, color, total soluble solids, acidity, ripening index, and vitamin C were performed on 10 different samples corresponding to 4 treatments.
3.4. Weight Loss
In Fig. 6, guava weight loss was analyzed which demonstrated that longer lengths of time in storage result in greater weight losses. Both treated and reference (or blank) fruits had less weight loss in comparison to the untreated fruits. The control fruits after 15 days of storage had a 66.1% weight loss which was higher when compared to other treatments.
The treatment identified by CV presented less weight loss after 15 days of storage at 34.0%. The difference was negligible when compared to the L + CV treatment. A statistical analysis was performed on the L + CV treatment and compared with the slopes of the linear regressions of each treatment in table 1. The analysis demonstrated that the best treatment to delay weight loss in guava fruit was the citral solution (CV).
The results demonstrated that treatments CV and, L + CV delayed weight loss in guava fruit. The application of citral was found to be key in the biochemical processes related to the process of ripening. These processes include the enzymatic degradation of the cell wall of the fruit which is evidenced by the softening of the fruit, modification in its texture, and changes in color. citral can also be said to interfere with the loss of water of the fruit during the ripening and senescence. [46]
3.5. Color
Fig. 7 shows the results of the change in color of the epidermis of the treated fruits. The fruits observed a gradual change in color varying from green to the characteristic yellow color indicating a semi-ripe or mature state. This change is attributed to the degradation of chlorophyll and the synthesis of new pigments such as carotenoids and anthocyanins [47] and act as an indicator of external quality and ripeness.
By the ninth day of storage the fruits treated with citral nanocontainers (L+CV) had a green color with slight yellow spots. This indicates that the fruits are in the best condition for commercialization and consumption. After the 15th day of storage the fruit turned a dark brown color, which can be considered over mature and in a state of decomposition.
Fruits denominated blank (BV), showed a greater change in color after the sixth day of storage. They showed a green color with yellow spots and after 15 days a yellow color with brown spots developed indicating that the fruit was over-ripe.
Fruits treated with liposomes (LV) showed a gradual change in coloration of the epidermis after the ninth day. This change is attributed to the lipid composition of nanocontainers. Biofilms that act as a barrier against moisture loss are developed with lipids as their main components. [48-49]. This contributes in controlling the loss of firmness, changes in the taste, and the appearance of the fruits. After the 15th day there was a change in color from a light green to a brown color indicating over ripeness. This is attributed to the degradation of lipids during the climacteric stage and senescence. [50]
Fruits treated with Citral (CV) presented a change in coloration from the ninth day. At the end of the storage time the fruits had a yellow color with a slight brown color and therefore considered mature. The results allow the inference to be made that treatments containing Citral are more effective in extending the shelf life of the green guava and delaying the change in color of the epidermis of the fruits.
3.6. Total soluble solids
One of the main indicators of fruit quality is the amount of total soluble solids. Fig. 8 shows the amount of total soluble solids in the guava during storage. Comparing ºBrix values, both treatments L+CV and LV reported an initial value of ± 5.5 ºBrix. As storage time increases the amount of total soluble solids also increases.
As storage times increased a significant difference was observed in fruits exposed to treatment L+CV which reported values between 1.5-2.0 ºBrix less than other treated fruits at sixth day. This demonstrates that the Citral nanocontainers treatment tends to decrease the content of soluble solids such as sugar, salts, and short chain acids among other compounds as the fruits ripen.
It was also noted that the BV treatment control fruits had a high content of total soluble solids with 10.5 °Brix after day 12; however, there was no significant difference between the fruits regardless of treatment. Additionally, guava fruit of the red variety showed values of relatively low soluble solids compared with the data reported in the literature. [51] This could be attributed to the state of ripeness of the selected fruits.
3.7. Acidity
Fig. 9 demonstrates that the acidity in the fruit decreases as the amount of storage time increases. This coincides with the literature and is directly related to the ripening of the guava fruit, wasting the organic acid. [52]
The acidity of the L+CV treated fruit during storage decreased by 50%. When comparing the applied treatments, the L+CV fruits had higher acidity content then those treated with CV. This demonstrates that the treatments have a side effect which is reflected in the lower acidity of the fruits. This is attributed to the fact that the fruit has a high carboxylic acid content which directly influences the ripening of the fruit and results in a decreased acid content.
The results show that the Citral nanocontainers (L+CV), is the best treatment to reduce the loss of acidity in the fruits. This is important because it is related to other factors in the fruit ripening process. Acidity of the fruits plays an important role in the aroma and flavor of fruit. This also influences the nutritional value and metabolism of the fruit given that acidity is an important aspect of respiration and its conversion to sugars during the ripening of the fruit. [53-54].
The ripening index in Fig. 10 was determined by the mathematical relationship between the total soluble solids (°Brix) and the percentage of acidity. A positive relationship between the two variables as storage time increased was observed.
The treatment L+CV stood out for having the lowest increase in the ripening index during storage with values between 5.1-21.4%. There was also a difference ≥ 2.5 units when compared to fruits without treatment (BV). The results suggest that the best treatment for guava is using Citral nanocontainers.
Additionally, towards the ninth day of storage, the best treatment is LV. It started with a 22% maturity and ended with 39% maturity. In order to compare this treatment with the other treatments, results from the fruit ripening index were applied to hypothesis tests for two samples assuming equal variances with a confidence of 95% (Table 2).
Table 2 Contrast t-test using two measurements assuming equal variances for the ripening index in guavas treated with Citral.
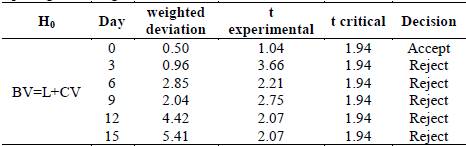
Source: The Authors.
Table 2 shows that the initial analysis does not present a significant difference in the fruits studied. The results indicate that the null hypothesis from that day can therefore be accepted. The fruits analyzed from day 3 present a significant difference and it can be inferred that the treatment had a significant effect on the ripening of the fruits, delaying senescence.
Fig. 11 shows that the vitamin C content starts at 0.01 to 0.02 mg and has an undefined behavior in all treatments. This result is due to the fact that the Vitamin C content increases as the ripening time increases, reaches a peak and then decreases.
Fruits denominated by L+CV, BV and, LV registered values around 0.01 milligram Vitamin C per gram of fresh fruit. Fruits denominated by CV registered a value around 0.020 attributed to the presence of Citral in the fruits. Fruits denominated by BV presented their maximum vitamin C content on day 3 of storage whereas other treated fruits presented their maximum vitamin C content on day 6. These results indicate that the fruit reaches their maximum maturation between day 3 and 6.
According to Sauvageot (1969) cited by Valencia et al 2002, the loss of vitamin C is related to oxygen and temperature. Increasing oxygen levels increase the rate of oxidation of vitamin C in the pulp of the fruit. [55] The results obtained using the Citral nanocontainers treatment had a large impact on decreasing the production of vitamin C during storage.
This result does not present a definite trend. For this reason, an analysis of variance of the vitamin C content in the guava fruits was performed and shown in table 3. This table demonstrates that there is a significant difference between the maximum values in vitamin C content by the different treatments in guava fruits.
Table 3 Analysis of variance of a factor of vitamin C content in guava fruit treated with Citral.
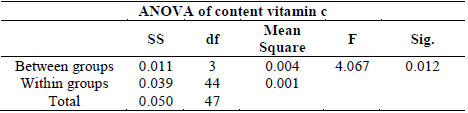
Source: The Authors.
The result of the analysis of variance (ANOVA), which assumed equal variances, allowed for an analysis using the multiple comparison test of tukey as shown in table 4. After analyzing the table, the best treatment identified to maintain vitamin C production was L + CV.
Table 4 Tukey test of maximum vitamin C content for each treatment of guavas with Citral.
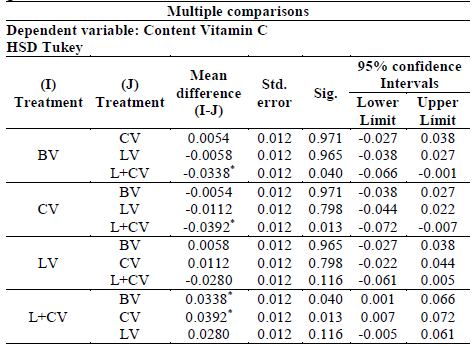
*. The difference in means is significant at the 0.05 level
Source: The Authors.
After multiple comparisons guava fruit denominated by BV presented a significant difference with respect to the fruits treated with Citral nanocontainers (L+CV). It was determined to retain a higher concentration of vitamin C.
The significant difference between the fruits treated with Citral nanocontainers (L+CV) and Citral (CV) was that the former presented higher concentration of vitamin C. Other treatments did not present nearly as significant differences.
4. Conclusion
The treatment of the guava with Citral nanocontainers presents better results in most of the physicochemical parameters analyzed. These results allow a connection to be made between the composition of the nanocontainers and Citral in contributing the increasing the shelf life of the fruits until 5 days. The principal reason for this is that the nanocontainers act as a method to transport Citral which is as retarding agent released under the environmental temperature of the region.