1. Introduction
Nowadays, studies are orienting towards the development of new multifunctional materials with properties such as ferroelectricity, ferromagnetism, and multiferrosity. Bismuth ferrite (BiFeO3) is a good example of multifunctional materials,since it has high Curie and Néel temperatures (TC = 1083 K, TN = 673 K) [1,2]. Besides, it exhibits ferromagnetic (FM), ferroelectric (FE), and ferroelasticity properties based on magnetization, electric polarization, and elastic effort, respectively [3]. These properties make the BiFeO3 a material of great interest in the scientific field. Although some materials (BiMnO3, TbMnO3, TbMn2O5, YMnO3, LuFeO4, and Ni3B7O13) show similar properties, only bismuth ferrite presents ferroelectricity and antiferromagnetism at room temperature [4,5].
Bismuth ferrite exhibits a G-type antiferromagnetic (G-AFM) ordering with a long-wavelength period ~62 nm, weak ferromagnetism and linear magnetoelectric effect [6]. In addition, it has a bad circuit ferroelectric, and polarization remnants due to charge defects linked to oxygen vacancies [7].
Recent studies have focused on improving the magnetic properties of bismuth ferrites through smaller particle sizes and avoiding the secondary phases. The bismuth substitution for rare earth elements such as samarium (Sm) [8], gadolinium (Gd) [9], neodymium (Nd) [10,11], holmium (Ho) [12] dysprosium (Dy) [13], praseodymium (Pr) [14] and lanthanum (La) [15], which allowed high densification and the elimination of not multiferroic phases [16], as well as their application in spintronics devices like field-effect transistors, electrical switching, nanoelectronics, magnetoelectric random access memories (MERAMs) and sensors [17].
In this paper Bi1-xLuxFeO3 system (x = 0.00, 0.02, and 0.04) was produced by the solid-state reaction method and characterized, studying the effect of lutetium doping on its morphological, structural, and magnetic properties.
2. Materials and methods
The Bi1-xLuxFeO3 (x=0.00, 0.02 and 0.04) samples were synthesized by solid-state reaction method. Stoichiometric amounts of the oxides Bi2O3, Fe2O3, and Lu2O3 with 99.9% purity were dried, weighed, calcined at 750 °C for 9 h and sintered at 800 °C for 9 h, with intermediate milling and pellet pressing.
The solids obtained were characterized by X-ray diffraction (XRD), using the DRX equipment PANalytical X'Pert PRO-MPD equipped with an Ultra-fast X'Celerator detector in Bragg-Brentano arrangement, using Cu Kα radiation (λ = 1.54060 Å) from 20° to 80° 2 Theta. The results were refined with the GSAS and PCW software. The morphological properties were evaluated by scanning electron microscopy (SEM) with FEI Quanta 200-r equipment. Finally, the magnetic characterization was carried out in a VersaLab-type magnetometer of vibrating sample from Quantum Design company, the measurements were made in the temperature range from 50 - 320 K and magnetic fields from -30 to 30 kOe. The zero-field cooled (ZFC) method was used to measure the magnetization as a function of temperature (at 1000 Oe).
3. Results and discussion
The diffractograms of the Bi1-xLuxFeO3 system are in Fig. 1. BFO, BFO2 and BFO4 are x = 0.00, 0.02 and 0.04, respectively. XRD signals revealed high crystallinity of the solids obtained. The patterns analysis evidenced the majority formation of bismuth ferrite (BiFeO3) with file code JCPDS-01-086-1518, rhombohedral structure, and space-group R3c (161), with preferential orientation on the (1 1 0) plane and the secondary phase Bi2Fe4O9 with file code JCPDS- 00-025-0090, orthorhombic structure, space-group Pbam (55) with a preferential orientation on the (1 2 1) plane [17]. The formation of the secondary phase was can be attributed to the reduced range of thermal stability that BiFeO3 presents. This decomposition is associated with high bismuth volatility, considering the synthesis temperature [18]. The XRD analysis shows that the secondary phase decreases when the percentage of lutetium doping increases, which stabilizes the main crystalline phase [19,20]. These results are in accordance with the reported by other authors [21].
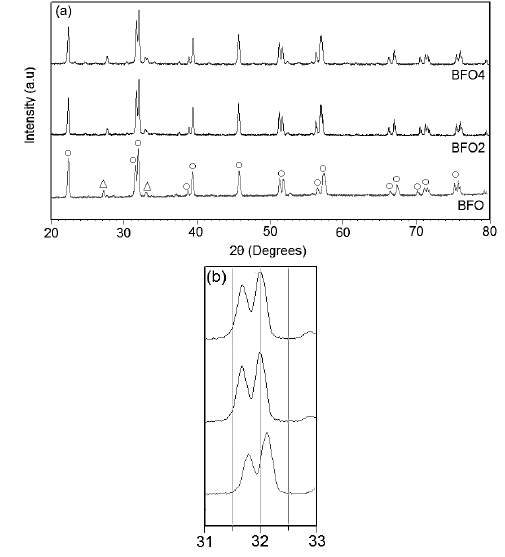
Source: The Authors.
Figure 1 (a) X-ray diffractograms of the Bi1-xLuxFeO3 samples: O main phase (BiFeO3), ( Bi2Fe4O9 phase, and (b) zoom-in of the main signals.
Fig. 1 (b) shows the zoom-in of the 31o-33o 2 Theta range. A shifting toward smaller angles was observed, which indicated the structural distortion generated by the ionic radius difference of substituent cation, which shows the correct lutetium doping in bismuth ferrite [22,23].
Rietveld refinement allowed determining the lattice parameters (Table 1). Fig. 2 shows the refined X-ray diffractogram of BFO2, a high correlation between the experimental and theoretical data was observed, with preferential orientation in the plane (1 1 0) located at 32o 2 Theta and Fig. 2 (b) shows the cell unit obtained with PCW software, which is characteristic of these ferrites [24].
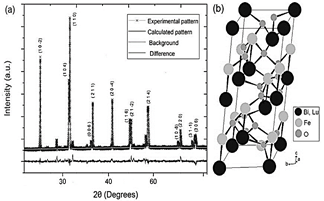
Source: The Authors.
Figure 2 (a) Results of the Rietveld refinement of BFO2 sample and (b) unit cell obtained from experimental X-ray data and plotted with PCW software.
Fig. 3 shows the micrographs obtained for each material, particle size distribution was determined using the image J. software. In the micrographs can be observed the presence of interconnected particles with well-defined edges, irregular shapes and sizes. Particle sizes were between 2.25 and 4.50 μm, the values are attributable to lutetium insertion at the A site of the ferrite. These results were correlated with the data obtained by Rietveld refinement (Fig. 4). Lu3+ cation has an ionic radius of 25% less than Bi3+ cation, causing the unit-cell volume reduction and the decrease in particle size [25]. Additionally, doping can suppress oxygen vacancy concentration, which leads to smaller particle sizes. This behavior is common in the substitution of rare earth elements into the bismuth ferrite structure [26].
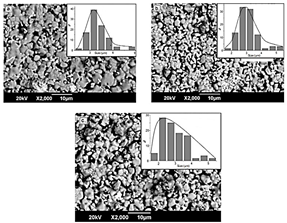
Source: The Authors.
Figure 3 Scanning electron microscopy images and particle size distribution for (a) BFO, (b) BFO2, and (c) BFO4.
Magnetic hysteresis loops, obtained at 50 and 200 K (Fig. 5), exhibits typical magnetism of bismuth ferrites systems. These magnetic properties are associated with unpaired electrons on the Fe3+ d-orbital, located at the B site. Remnant magnetization values increase with the higher x values (Table 2), which is due to the ionic radium of Lu3+ is lower compared to the one for Bi3+. Therefore, doping affects the bonds between Bi-O and Fe-O [27-28].
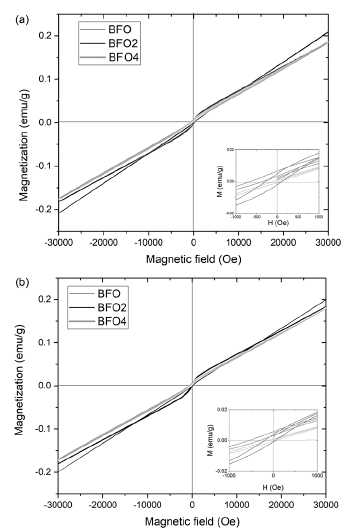
Source: The Authors.
Figure 5 Magnetization as a function of magnetic field (a) 50 K and (b) 200 K of the Bi1-x LuxFeO3 samples.
Table 2 Values of coercive field and remnant magnetization for the Bi1-xLuxFeO3 system.
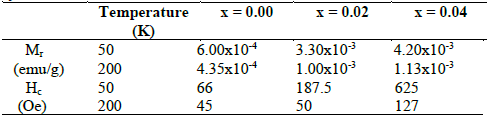
Source: The Authors.
The results show a linear increase in magnetization in regards to the applied field without magnetic saturation. This is attributed to antiferromagnetic ordering and spin structure in the material [29]. The zoom-in section of hysteresis loops showed a slight ferromagnetic behavior for each material, the small hysteresis loops of BiFeO3 is due to parasitic ferromagnetism caused by the magnetic moments and spin canting effect in the antiferromagnetic network [30,31].
The magnetization results are in accordance with the phases found by XRD. Ferromagnetic ordering is associated with the presence of a secondary phase (Bi2Fe4O9) and the antiferromagnetic ordering is caused by the main phase [32].
Magnetization as a function of temperature is shown in Fig. 6. Some transitions occur at two temperatures: at 250 K is presented the typical transition PM-AFM characteristic of bismuth ferrite systems and at 120 K was presented a slight curvature attributed to the lutetium insertion. It should be noted that Lu3+ ion is non-magnetic, and Bi3+ ion has a low energy multiplet, which can contribute to the Van Vleck-type magnetic susceptibility. Under 120 K, the negative values are due to the susceptibility of the antiferromagnetic phase [33].
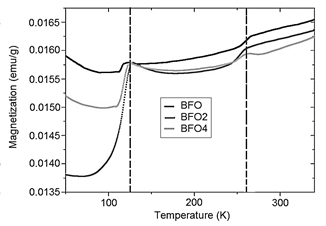
Source: The Authors.
Figure 6 Magnetization as a function of temperature from 50 to 320 K for Bi1-xLuxFeO3 samples under 1000 Oe magnetic field.
Some researches attribute the increase of magnetization to size particle reduction, which is because of enhancing the uncompensated antiferromagnetic spins in the material surface. In contrast, the magnetization increase was not associated with a direct contribution of lutetium, since it is not a magnetic ion. Nevertheless, it was attributed to the high structural stability of the new material and the decrease of the lattice parameters. [34,35].
4. Conclusion
Lutetium doped Bismuth ferrite in low percentages (2% and 4%) was synthesized by solid-state reaction using lower temperatures than those reported in the literature. The materials showed a majority phase of rhombohedral structure and space-group of R3c (161) with the presence of the secondary phase (Bi2Fe4O9). These results were confirmed by Rietveld refinement, which showed that the insertion of lutetium cation improves structural stability and decreases the lattice parameters. SEM analyzes showed the formation of particles with smaller sizes by increasing the x value, this is due to the small Lu3+ ionic radius. The increase of magnetization, allowed to demonstrate that lutetium substitution was performed efficiently and favored the secondary phase diminution and size particle reduction, generating better magnetic properties.