1. Introduction
Electrical signals are present in many physiological activities. But this study started long ago, when plants as Mimosa pudica, Drosera, and Dionea muscipula, attracted the attention of researchers as Pfeffer, Burdon-Sanderson, Darwin, Haberlandt, and Bose [1-4]. Who discovered that the tactile movements of these plants activated action potentials that were propagated from the stimulation site to motor organs, where the movement of sensitive cells occurs, in response to the stimuli, similar to nerves in animals [5-7]. Still, this electrical behavior is also important in non-sensitive upper plants [8,9]. These signaling mechanisms may include hormones (e.g. abscisic acid, salicylic acid, ethylene, jasmonic acid, etc), chemicals (e.g. Ca2+, K+, sugars, proline, amino acids, polyamines, reactive oxygen species (ROS), etc.) or physical events (e.g. propagation of electrical or hydrostatic pressure waves, changes in osmotic pressure, etc.) [10,11].
In plant cells, ion channels mainly relate to three physiological functions: cell osmoregulation, cell signaling by amplification, and electrical signals propagation, that include the control of membrane potential. Depending on the opening or closing of ion channels, the plasma membrane can be depolarized, repolarized or hyperpolarized; developing unique electrical properties based mainly on the transport of H+, K+ and anions [12-15]. Growth (the result of cell division) and differentiation (due to asymmetricity) are the two key developmental processes in all organisms. In fact, it is known that cell polarity and uneven differentiation as a result of uneven organization of cellular components precede differentiation [14,15].
Plants have been documented to develop electrical signals in the processes of respiration and photosynthesis [16] phloem transport [17], acid rain [18], irradiation with various lengths of wave [19], and the rapid deployment of plant defenses throughout the plant [20]. The bio-electrochemical system in plants regulates not only responses to biotic and abiotic stress, but also photosynthetic, growth, development and adaptation processes [20]. In this context, several agents including phytohormone gradients, light, pH, temperature and electro-magnetic fields can perturbate previously homogeneous systems and induce their subsequent heterogeneity and polarity.
There are different types of electrical signals in plants, such as local electrical potential (LED), action potential (AP), variation potential (VP, or slow wave - SW) [21,22][8]. APs after a stimulus-induced by non-damaging attacks such as illumination, cold, mechanical, and electrical stimuli, reaches a certain threshold regardless of the stimulus's force, which leads to membrane depolarization [23,22]. VPs are slower signals related to stimulus strength with variable shape, amplitude, and time, lasting periods of 10s to 30m, induced by damaging stimuli such as burns and cuts [24]. LEP is a sub-threshold response induced by change in environmental factors (e.g. soil, water, fertility, light, air temperature and humidity). Although LEP is only locally generated and is not transferred to other parts of a plant, it has tremendous impact on the physiological status of the plant [23,22]. In contrast, both AP and VP can transmit from the stimulated site to other parts of the plant.
Approaches used to measure electrical activity in plants mainly include intracellular and extracellular measurements [2,22]. Intracellular measurements directly and individually record the value of cell membrane potential; the procedure generally consists of inserting a microelectrode into a cell's cytoplasm carefully using micromanipulators. Whereas extracellular measurements are based on the acquisition of the total sum of signals produced by the depolarization-repolarization process for large groups of cells. Extracellular measurements are widely used in animal electrophysiology (electrocardiograms (ECG), surface electromyography (SEMG) and electroencephalograms (EEG)) [25,26].
Studies have been carried out on electrical signals in plants under various conditions (laboratory, greenhouse, etc.). For example, in [22], the authors report the development and testing of an electrophysiological sensor for use in greenhouse conditions, without a Faraday cage, and the automatic classification of these signals using supervised machine learning allowing the detection of these physiological modifications due to environmental conditions. In [27], a system for constant monitoring of cucumber plants' electrical signals in a greenhouse was developed, showing that the electrical signals in plants respond to environmental changes. On the other hand, [9] determined the electrical responses, both VP and AP, of tomato plants wounded by flame.
This review covers from a historical background of the plant electrophysiology up to the uses that researchers have given to this behavior, together with an understanding of the mechanism of generation and conduction of the electrical signal in plants. Using a bibliometric analysis, the plant electrophysiology trend is towards the application in agriculture and environmental monitoring, promoting the development of mixed societies of biological and artificial components, which take advantage of the intrinsic detection capacity of plants.
2. Bibliometric analysis
A systematic review of research that recorded the analysis of the electrical activity in plants under conditions of biotic and abiotic stress was performed, emphasizing the type of electrode used, the potential measured, and the study's application. The search strategy was developed by identifying the following relevant terms: Plant electrophysiology, plant electrical measurements, and pollution, plants as environmental biosensors, and electrical potentials in plants used in the electronic databases: EBSCO, IEEE, ScienceDirect, Scopus and Web of Science. The inclusion criteria emphasized recording electrical activity in plants in specific applications such as monitoring and study systems, plant disease development, pollution detection, and other applications. Exclusion criteria included mathematical modeling investigations and behavioral simulation.
Plants are structures with ideal adaptive capacities where electrical processes play an important role. The objective of this research is to obtain a better understanding of the behaviors, interactions and communications between the plants, as well as the applications that have been reported. Plants are exposed daily to a wide variety of environmental factors, which induce disturbances in them, including variation in light, air and soil pollution, temperature, water deficiency, mechanical damage, application of nutrients, insect attacks and pathogens, etc. The electrical response that plants have to these biotic and abiotic stimuli allows the development of biosensors for application in different areas such as agriculture, environmental monitoring, detection of geological events, detection of pollutants, to name a few. Research has been carried out for a long time in the field of plant electrophysiology, although studies have been reported since 1873, in recent years annual scientific production has increased, having an annual growth rate of 2%, as shown in fig. Motivating the appearance of new scientific journals and their growth over time (Figs. 1 and 2).
The study of the electrophysiology of plants has been opening the way in many different fields to the intrinsic behavior of plants, increasing the number of authors over time, indicating that it is an area that is constantly growing. Considering the number of articles published, the Fig. 3 shows the scientific production of the 20 main researchers.
This scientific production has been concentrated mainly in 3 areas and some pilot studies. As can be seen in the Fig. 4, the reported works are concentrated in greater quantity in experimental analyzes applying controlled stimuli, occupying 42% of the studies. On the other hand, the detection of pests and pollutants with 22% and 24% respectively, show to be areas of interest for the researchers considering the enormous potential that exists in using the plants as biosensors of the environment regardless of whether the application is agriculture or environmental analysis (Fig. 4).
In response to environmental changes, plants permanently adjust their metabolic and physiological processes. They continually collect and systematize information about their environment. This is the result of an incredibly complex set of molecular, biochemical and physiological mechanisms for signal perception and activation of multiple cross-signaling pathways. Plants mainly generate various types of intracellular and extracellular signals in the form of AP and VP, in response to environmental changes. This information together with computerized methods offers the possibility of obtaining low-cost biosensors that can be used as a network in different applications, as studied above. These trends make it possible to obtain mixed societies of biological and artificial organisms in agriculture and mainly in environmental monitoring.
Many authors through laboratory studies have demonstrated the potential of using plants as sensors to detect what biotic and abiotic stresses are for plants. In agriculture it is possible to detect by monitoring its electrical activity the application of chemical products, which would bring sustainable and ecological agriculture, optimizing inputs as well as water resources. Monitoring of the environment and pollution is another interesting application since it is possible to use plants as low-cost sensor networks to analyze environmental behavior from homes to correlate it with diseases and air purification needs. Considering that plants have pathways for transmitting electrical signals to respond quickly to environmental stressors.
3. Electrical activity in plants
Plant cells also produce and transfer bioelectrical signals as extracellular signals in response to changes in their environmental conditions [22]. Also, investigations confirmed that the neurotransmitters found in higher plants (e.g. acetylcholine ergic) participated in the bioelectrical activity of higher plants to regulate the membrane permeability of plant cells and the physiological processes in higher plants [5-7]. Accordingly, electrical signals are probably the initial response of the plant to an exterior stimulus. This type of response may trigger physiological variation (e.g. elongation growth, respiration, moisture absorption, substance unloading at the phloem, reduction of the turgor pressure and variation of photosynthesis and transpiration, gas exchange, and activation and transcription of the protease inhibitor gene), and thus may mediate the interrelationships between each organ and tissue inside the plant as well as between itself and the external environment [28].
During plant growth, electrical signals in plants can show different characteristics due to weak light, high humidity, and low potassium [29]. This suggests the potential use of these electrical signals in applications that indicate the physiological state of plants for the adjustment and control of biotic and abiotic conditions. Electrical signals in plants can carry local stimulation information to other cells, tissues, and organs so that they respond appropriately. Therefore, electrical signals are very important and have important physiological effects on plants [27,28].
3.1 Variation Potentials (VP)
VPs are induced in the xylem parenchyma cells by a local change in a hydraulic wave or a chemical stimulus. They are characterized by amplitudes and velocities that decrease with increasing distance from the generation site and xylem tension. Their ionic base differs from the underlying APs in that they are induced by a transient quenching of a P-type ATPase-H+ and a turgor-dependent activation of Ca2+ mechanosensitive channels [2,28]. The VP are mainly caused by injuries such as burns [30] or hot water treatment [31]. This potential does not follow the all-or-nothing principle, are correlated with stimulus strength, and last a few seconds to 30 minutes [28]. Additionally, VP have a slower transmission rate than APs; most of the plant AP studied so far have a velocity in the range of 0.005-0.2 m*s-1 [32], while the velocity of the VP are in the range of 0.001-0.01 m*s-1 and their amplitude decreases with increasing distance from the stimulation site along their path [28].
3.2 Action Potentials (AP)
An AP generally travels with constant speed and magnitude [33]. APs are generally triggered by noninvasive stimuli [32]. They are transmitted along the phloem over long distances [34] and regulate the rapid movements of the leaves in plants sensitive to touch such as Mimosa [29] and Venus [35]. Furthermore, cold shock [36], as well as in some specific physiological functions [37]. As in animal neurons, APs follow the all-or-nothing principle [38].
APs in higher plants are the information carriers in intracellular and intercellular communication during biotic and abiotic environmental changes [18,39]. Plants possess most of the chemistry of the neuromotor system in animals, that is, neurotransmitters, such as acetylcholine, cellular messengers such as calmodulin, cellular motors, for example, actin and myosin, voltage-gated ion channels, and contact sensors, light, gravity and temperature. Although this cellular equipment has not reached the same great complexity as the case of the nerves, a very simple neural network has been formed within the phloem that allows plants to successfully communicate over long distances. The reason that plants have developed pathways for the transmission of electrical signals through oscillations in their molecule, ion, and voltage fluxes probably lies in the need to respond quickly to environmental stressors.
The lengthening or growth of the roots and of the leaves has been associated with electrical signaling and, therefore, with the displacement of ions, such as Ca2+, Cl- and H+, through the membranes [40]. Ion flux waves constitute an alternative long-distance and rapid signaling network in plant that integrates and responds to a number of external and environmental signals, which are often related to stress signaling, e.g. ROS [41]. The quality of light, that is, of the spectrum as a whole, which wavelengths of irradiance are perceived, influences the type of electrical signals that are developed [41]. For example, blue light (400-500 nm) induced rapid AP in soybeans, while 500-630 nm did not induce any membrane potential [42], leading to the conclusion that irradiation of blue light induces positive phototropism.
4. Methods to measure electrical activity
4.1 Plant intracellular measurement
Intracellular measurement directly records a cell's plasma membrane potential, usually using a glass microelectrode with a tip diameter lower than 1 µm. Different methods have been used such as a glass microelectrode with 500 mM KCl and Ag/AgCl immersed in the bath solution using a micromanipulator [24], microelectrode filled with 100 mM KCl was inserted into a mesophyll cell [43,30], 3 M KCl filled glass microelectrodes Ag/AgCl [44], electrodes made from capillary glass [45,26]. In [38], they fabricate glass microelectrode from microcapillaries (WPI) were with tip diameters less than 1 µm and were back-filled with 100 mol m~3 KG. In [46] is reported a glass micropipette pulled on a vertical puller, with aperture diameter at the tip of 0.05 µm, filled with a 1% aqueous solution of LYCH and back-filled with 3 M LiC1. In this method, the microelectrode is inserted into the cell, through a micromanipulator with a stereomicroscope, and the reference electrode is placed in the bath solution. The ingredients and concentrations of the bath solution vary depending on the plant cells' conditions to be analyzed.
4.2 Plant extracellular measurement
Measurements of extracellular potential allow detecting differences in the stable electrical potential of groups of cells for extended periods (several days), this technique can be carried out mainly by two methods, through surface recording or measurements with inserted electrodes [47,48]. In many cases inside of a Faraday cage and using several filters with a recording system composed of preamplifiers, A/D converter and devices for recording, sampled at 400 Hz with a gain of 4 and several filters are applied: low pass at 30 Hz and band-stop at 50-60 Hz and 100 Hz.
The surface recording method is non-invasive and has been reported to be physically stable, generally consisting of electrodes attached to the plant's surface, wrapped in cotton to provide adequate contact [49,50]. Different electrodes, electrode wetting solutions, and bonding methods have been reported.
Electrodes as: Ag/AgCl electrode [24][51], nonpolarizable reversible Ag/AgCl electrodes prepared from Teflon coated silver wire [52-54], graphite patch electrodes [55].
The union of the electrode with the plant has been analyzed in different ways as: Ag/AgCl electrodes connect to the plant surface using an aqueous conductive gel similar to that of ECG [56,57], Ag/AgCl connected with conductive gel and the reference electrode a solution (1 mM KCl, 0.5 mM CaCl2 and 0.1 mM NaCl) surrounding the roots [58-60][43], Ag/AgCl-wire (0.4mm in diameter) wrapped in cotton moistened with 01% (w/v) KCI solution [38][47], Ag/AgCl electrodes covered by a conductive hydrogel [61], felt-tip calomel electrodes attached to de plant with 1 mM KCI ionic bridges [62,63][9], Ag/AgCl electrodes moistened with 100 mM KCl agar [64], Ag/AgCl electrodes prepared with 0.2 mm silver wire and 0.05mm elastic cooper wire [65], Non-polarizable Ag-AgCl electrodes connected by an agar-coated cotton thread protruding from a glass pipette containing 0.1M KCl gelled with 1% agar [66]. Besides, the bath electrode method has been used in [27,67,68,46], where is used a cotton thread soaked in an experimental solution (KCl 0.1 mM, MgCl2 0.1 mM, CaCl2 0.5 mM, Na2SO4 0.05 mM), or an Ag/AgCl-pellet electrode impaled into agar with KCl.
The electrode insertion method commonly uses thin metal wires that, when inserted into the shoot or the plants' leaf vein, come into contact with the internal tissue incorporating large groups of cells. This method produces wounds in the plants that can alter the external stimulus measurements. Different electrodes have been reported as thin metal tips made of platinum (Pt) or Ag/AgCl wires have been reported as thin metal tips made of platinum (Pt) or Ag/AgCl wires [49,69-71,46], metal probe [72], Ag/AgCl electrodes from Teflon-coated silver wires [73], silver wires (0.2 mm in diameter) [62,63,9], microelectrode filled with 100 mM KCl [64], wires (50/im diameter 10% iridium-platinum) [66], reversible Ag/AgCl electrodes prepared with AgCl on 5mm long silver wire tips without Teflon coating in a 0.1 MKCl aqueous solution [61], Ion-selective microelectrodes describe by [74]. In [16], two types of electrodes were used: glass microelectrodes from boro-silicate glass (Hilgenberg, Malsfeld, Germany) filled with 3 mol/L KCl solution, and conventional Ag/AgCl electrodes made of silver wires 0.2 mm in diameter coated with AgCl. [7] used custom-made electrodes of silver-coated copper filament diameter less than 0.5 mm of a coaxial cable (2.79 mm diameter). In [75-77] used three stainless steel needle electrodes of 0.35 mm in diameter and 15 mm in length (base, in the middle and on top of the stem), similar to those used in Electromyography. Solid stainless steel has been used in [78] stainless steel 304 SS type 316 rod, 3.18 cm long and 0.2 cm diameter, and in [79] 15mm electrodes wrapped with a cable secured an epoxied cap of thermo-sheath. In other studies, use nonpolarizable reversible Ag/AgCl-electrodes with a diameter of 0.25 mm [20], 0.14 mm [80], and 0.35mm [15].
Also, the aphid technique has been reported. This method consists of using the stylet of an aphid when it pierces the sap in the phloem to attach a microelectrode to it, using a micromanipulator. The stylet works as a salt bridge between the cytoplasm and the microelectrode [48,81-84].
Other techniques often used for acquiring the electrical potentials are:
Patch-clamp recording technique involves sealing glass capillaries to the protoplast membranes' surface and then opening a small hole with a short voltage pulse. The plasma membrane's inner surface then contacts the solution in the glass tube, allowing the movement of ions to be traced using their electronic charges [48]. This technique records the ion channel as Outward rectifying K+ channel [85,86], Inward rectifying K+ channel [87,88], Fast anion channel [89] and Slow anion channel [90]. In [91] prepared the patch pipettes from Kimax-51 glass capillaries and coated with silicone, the pipette solution contained 150 mm CsCl, 1 mm MgCl2, 10 mm EGTA, 1 mm MgATP, and 10 mm HEPES/Tris, pH 7.4.
The non-invasive microelectrode vibrating probe technique measures ionic or molecular activity without invading the cell, using a reference electrode and an ion selectivity microelectrode, with an electrolyte and a liquid ion exchanger. By vibrating the electrode determines the voltage across the ion concentration gradient [48]. With this technique, in [92] investigated in the initial phases of plasma membrane depolarization, which ion act as the depolarizing agent, besides they determined the kinetics of H+, Ca2+, K+, and Cl- fluxes and the changes in their concentrations near bean mesophyll and attached epidermis.
Optical measurements using voltage-sensitive dye (VSD) in this technique are captured with high-resolution cameras. The optical signals emitted by the VSD attached to the plant cells are analyzed. This technique has been applied by [13]. For membrane staining have used different voltage-sensitive dye, VSD bis-(1,3-dibutylbarbituric acid)-trimethine oxonol (DiBAC4(3)) [24], RH-414 [-N-(3-triethylammoniumpropyl)-4-(4-(4-diethylamino)phenyl)butadienyl) pyridinium- dibromide] [93].
5. Applications
The electrical activity in plants not only appears as a reaction to the impact of chemical compounds such as herbicides, air and soil pollution, stimulants of plant growth, salts, water, etc.; but also, with physical factors such as electromagnetic fields, mechanical injuries, changes in temperature, among others. The behavior of electrical activity in plants has been an area of particular interest in recent and ancient studies. The applications that previous researchers have worked on are presented in groups below.
5.1 Monitoring and study systems
Monitoring the plant's response to environmental changes is an active field in plant electrophysiology. Several studies have been conducted that considers temperature, humidity, light, and other factors. Special attention has been paid to the studies of Mimosa pudica (sensitive plant), Drosera (sundew), Dionaea muscipula (flytrap) the significant action potential that generates when stimulated [94,95,4,66].
Studies have found patterns of electrical potentials in plants under water stress, in [96-98,15,55,22] was observed an increase in the electrical signal in non-irrigated fruit plants, which changes when starting irrigation, producing a negative signal slope until reaching stability under normal conditions of irrigation. The roots can feel the soil's drying and send signals to the leaves to coordinate the processes over a long distance, especially in large plants where water transport is slower [99] (Fig. 5).
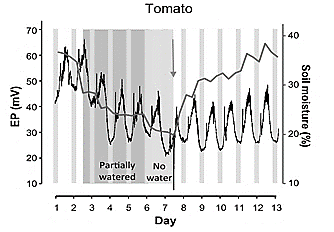
Source: [22].
Figure 5 Changes of electrical potential resulting from irrigating. a) Experiment with tomato plant reported in 2019.
Thermal stress causes changes in the magnitude of the electrical signal; the decrease in electrical signals' magnitude has been evidenced by the reduction of temperature [27]. Furthermore, it has been found that the speed of propagation of action potentials against thermal stress is comparable to that produced by various species of mammals [52].
Light is another environmental factor that induces changes in the electrical potential of plants. Low illumination causes a decrease in the electrical activity of plants [27,100]. Dark light transitions trigger potential membrane changes in the leaf mesophyll, as well as at the root level [101], thereby modulating K+ ion fluxes, Ca2+, Cl- and H+. In the case of roots, the light-induced electrical signal was faster than the hydraulic signal (reduction of xylem pressure), indicating a predominant role of electrical signaling in ion fluxes and nutrient absorption at the root level [101].
The mechanical damage caused by pruning, wind, or insects can be related to the intensity and duration of the said stimulus, as reported by [102,56], who have conducted experiments with Persea Americana (avocado) and Vitis vinifera (grape) plants.
Other reported studies have developed new algorithms to compare with a neural network, deep learning, and SVM to identify and classify action potentials when there is an electrical stimulus [103].
These studies allow to know the plants' behavior, develop instruments for continuous monitoring, and evaluate in real-time the electrical response of the plants in stress situations under field conditions for modifying environmental conditions, maximizing productivity and quality.
5.2 Plant disease development
The existence of variation of the membrane potential upon the attack of herbivores has been analyzed in some studies. Insects cause mechanical damage to plants by chewing them. This action has been studied by [104] finding that plant-insect interactions produce depolarization in the plant; this electrical signal travels rapidly throughout the plant, from the origin of the stimulus, causing a plant response. Plants differentiate mechanical wounding from herbivory through recognition of compounds present in insect oral secretions; a faster depolarization response has been shown to exist when damage is done by insect herbivory [105-107].
Studies have shown the possibility of monitoring the development of virus infections in plants. In [108], a relationship between the progression of tobacco ringspot virus (TRSV) infection in Vigna sinensis plants with the frequency of hyperpolarized and depolarized transmembrane potential was evidenced. The study showed that the response to the metabolic inhibitor sodium azide varied between control plants (H) and cells infected with TRW. Results that can be contrasted with the studies of [109-111].
In the study carried out by [112], it was observed that when causing mechanical damage to a lima bean leaf, a strong depolarization response occurs in the bite area, followed by a transient hyperpolarization and, finally, a constant depolarization in the rest of the sheet.
5.3 Pollution detection
Signals of electrical activity in plants have also been used to monitor environmental conditions and provide information related to pollution. In [113] the authors propose a method of segmentation of time-series measurements to analyze electrical signals from ligustrum and buxus plants in the detection of critical levels of ozone (O3) plant's response to air pollution with O3, see in Fig. 6.
These results can be contrasted with those obtained by [114]. The study used a correlation-based approach to analyze electrical signals from Ligustrum texanum and Buxus macrophilla. The behavior of the biosensor was similar, and in the study a decrease in the electrical signal was observed in the face of ozone pollution.
Other contaminants such as sulfuric (H2SO4) and nitric acids (HNO3) can also be sensed using action potentials. These two acids that predominate in acid rain were analyzed by [115,20], in the study, effects were found in variations of action potentials induced by the application of H2SO4 or HNO3 in soybeans by acidification of the soil or spray on the leaves. The duration of action potentials reported, after the application of acid HNO3 and H2SO4, was 0.2 and 0.02 s.
The response of the plant with environmental pollutants as H2SO4, O3 and sodium chloride (NaCl), was also analyzed by [75], in the study the authors used tomato (Solanum lycopersicum) and cucumber (Cucumis sativus) plants; the work focused on the classification of the electrical signal extracted upon the stimuli H2SO4, O3 and NaCl in two different quantities (5 mL and 10 mL), using a machine learning approach, they obtained an average classification of 70% precision. The figure shows the behavior of the plants that the authors obtained with the study. In a similar study reported by [116], a curve fitting approach is shown in extracting signal characteristics to classify H2SO4, O3 and NaCl stimuli. In the study, the researchers obtained a classification accuracy of 98% (Fig. 7).
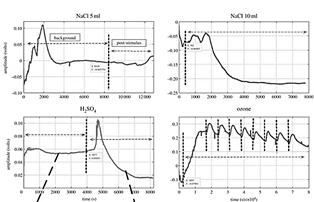
Source: [75].
Figure 7 Plant response upon 3 stimuli, vertical dotted lines mark indicates the application of stimulus.
The electrical response of the plant to pollutant gas was reported by [117], in the study the authors observed a decrease in the electrical activity of the plant, when it is placed in the presence of formaldehyde gas, the electrical change is attributed to the effect of plant's air purification.
On the other hand, uncoupler carbonyl cyanide-p-trifluoromethoxyphenyl hydrazone (FCCP) have been analyzed by several authors, and they have found that the FCCP induces ultra-fast action potentials and decreases the resting potential in a soybean [42,20,118].
The behavior of the electrical activity of plants upon sources of contamination was also studied by [80], they observed that there are fast-acting potentials and decreases the potential for variation to zero in soybeans, when exposed to pentachlorophenol (PCP), which is a contaminant used as an herbicide, insecticide, and fungicide.
5.4 Other applications
In other reported studies, the polarity of the plant tissue has been identified, in order to obtain a sustainable energy source. In the study of [72], they identified polarity based on plant growth and noted that by assembling branch sections in series or parallel arrangements, it is possible to increase the voltage or current depending on the arrangement. The power generation of the plant was also the object of study of [119], in the reported work, a self-powered wireless system was developed, which collects the energy of the plant itself for its operation and transmits the signal generated by the plant with stimuli of electricity and water, the study was carried out on avocado plants.
As reported in [120], electrical activity has potential use in the detection of seismic events, in the experiments of [121] and [122] using silk trees (Albizzia julibrissima) and Ulmus kaeki, respectively, it was possible to detect abnormal bioelectric potentials in trees before earthquakes, the hypothesis that is handled is that plants can perceive underground electromagnetic emissions or geochemical reactions.
6. Conclusion and future research
Plants have pathways for the transmission of electrical signals to respond to environmental stressors, the relationship between biotic and abiotic stimuli and the electrical activity of plants is still under study and takes more strength over the years, since it is an important factor for the development of biosensors that use plants as a detection device. For this reason, researchers have reported various types of sensors to acquire the electrical signal, as well as algorithms capable of recognizing behavior patterns and opening the way to applications in many fields.
The use of computerized methods interconnected with plants using intracellular or extracellular acquisition systems enables the development of biosensors for rapid and real-time monitoring of the environment; in agriculture control factors that influence the harvest; detect contaminants and pesticides; climate change detection; There are many applications that offers the electrical behavior of plants when stimulated.
It is evident that there is great potential for future hybrid applications between biological and artificial organisms, in which the environment will benefit since it will be possible from an optimal and sustainable agricultural production, through the pollution monitoring for improving the quality of life, to obtaining applications in bioelectricity, bioelectronics and biometrics.
The study of the mechanisms of electrical signals in higher plants in response to environmental changes during growth and development is still a subject under construction. Despite the large number of reports describing electrical signals in higher plants, there are few quantitative mechanism models that allow a reliable prediction of the measured shapes of electrical signals in higher plants. It is necessary to develop mathematical models to provide an explanation for electrical phenomena in plants. A more complete understanding of electrogenic ion transport systems involving their density and the corresponding voltage-dependent kinetics is required. New measurement methods (for example, the non-invasive microelectrode vibrating probe technique, the patch clamp technique, as well as modern modeling and stimulation methods) can provide support for the development of an accurate kinetic model of the signal’s plants.