Introduction
The black cutworm Agrotis ipsilon (Hufnagel, 1767) (Lepidoptera: Noctuidae) is a polyphagous pest that feeds on nearly all varieties of vegetables and many important grains, attacking mainly Solanaceae, Cruciferae and Curcubitaceae; however, they can also attack other species of different plant families, such as corn and soybeans (Link and Pedrolo 1987; Fernandes et al. 2013). This pest is a nocturnal insect, and when the larvae are touched, they roll up, becoming thread-like in appearance. During the day, the insect remains buried in the ground, which hinders its viewing field and consequently its control (Bento et al. 2007). In corn crop, younger larvae damage the leaves, and from the 3rd and 4th instars, they cut the base of the stem, causing streaks on the leaves, and the appearance of cuts in the seedlings at the base of the stem, resulting in losses of up to 27 % (Salvadori 2011).
The control of the black cutworm becomes difficult due to the insect’s habits. Nevertheless, studies have reported the use of allelochemicals to inhibit the growth of larval stages (Reese and Beck 1976). In relation to the biological control, the presence of parasitoids and predators in the field and the consequent reduction in the insect population was reported by Link and Costa (1984). The use of Bacillus thuringiensis in the laboratory (Menezes et al. 2010) and in the field, by means of transgenic plants (Kullik et al. 2011), as well as the use of Baculovirus (Prater et al. 2006) have also been reported.
Entomopathogenic nematodes (EPNs) are insect parasites, which insecticide activity is given by a symbiosis with bacteria ( Xenorhabdus and Photorhabdus), leading the host to death. This is one of the reasons of the successful microbial control (Grewal et al. 2001; Lewis et al. 2006). Infective juveniles (IJs) invade the body of their hosts and release their bacteria at the hemolymph stage, causing fast death of the insect by septicemia. Afterwards, the EPNs feed digested tissues of the dead insect and of the bacteria and reproduce themselves in several generations. When the food is depleted, the IJs leave the host searching for new hosts (Poinar 1990; Ferraz 1998).
EPNs present great tolerance to variations in environmental conditions. They can be used in combination with other control techniques, including chemical control, and are more recommended for the control of insect pests that present cryptic habitats associated with soil, since the nematodes can move themselves searching for a host (Ferraz 1998; Toledo et al. 2010).
Studies on EPNs are recent in Brazil. In the last few years, some works developed in the laboratory have demonstrated the potential of these agents to control several pests (Machado et al. 2005; Alves et al. 2009; Alves et al. 2012; Bortoluzzi et al. 2013). For instance, Leite et al. (2005), Andaló et al. (2010), and Andaló et al. (2012) studied insects of the order Lepidoptera, but there are no studies with Brazilian isolates aimed the control of A. ipsilon.
Thus, this work aims to evaluate the potential of Brazilian isolates of entomopathogenic nematodes by means of selection of isolates for A. ipsilon; to analyze the susceptibility at different instars of the insect and different concentrations; to investigate the in vivo production of these isolates; and to perform greenhouse tests.
Materials and methods
Entomopathogenic nematodes isolates
The EPNs isolates used in this work were obtained by the Universidade Federal de Lavras (UFLA), MG, Embrapa, Trigo, RS, and the Instituto Biológico de Campinas, SP, Brazil. Isolates were reared in Galleria mellonella (L.) (Lepidoptera: Pyralidae) last-instar larvae, according to the methodology described by Molina and López (2001). Ten G. mellonella larvae were placed in Petri dish (9 cm of diameter), containing two sheets of filter paper and 2 mL of aqueous suspension at approximately 500 Infective Juveniles (IJs)/mL. Petri dishes were kept for three days in climatic chamber at 25 ± 1 °C, with relative humidity (RH) of 70 ± 10 %, and 12 h photoperiod, when dead larvae and those with symptoms of infection by nematodes were transferred to Petri dishes with filter paper and kept in climatic chamber for five more days.
After this period, larvae were transferred to White trap (White 1927), and the suspension was taken daily and stored in a Becker containing distilled water and pump for aeration for at the most one week before being used in the tests.
Selection of entomopathogenic nematodes isolates
The nine isolates used for the selection test (Table 1) had been previously reared by the method described by Molina and López (2001). The experiment was carried out in 100 mL plastic cups containing 7 g of sterile vermiculite, which had previously received two seeds of corn, and were maintained at room temperature for about a week, until seeds germination.
Table 1 List of the isolates of entomopathogenic nematodes (genera/species), place of origin, institution and mortality rate caused in Agrotis ipsilon under laboratory conditions.
Isolates | Genus/ species | LOrigin | Institution | Mortality (%) |
---|---|---|---|---|
GL | Heterorhabditis amazonensis | Lavras - MG - Brazil | UFLA | 90 |
RSC05 | Heterorhabditis amazonensis | Benjamin Constant - AM - Brazil | UFLA | 80 |
JPM4 | Heterorhabditis sp. | Lavras - MG - Brazil | UFLA | 80 |
CH3 | Steinernema sp. | Campinas - SP - Brazil | Inst. Biológico | 100 |
IBCB-n02 | Steinernema carpocapsae | Flórida - USA | Inst. Biológico | 90 |
IBCB-n05 | Heterorhabditis indica | Itapetininga - SP - Brazil | Inst. Biológico | 90 |
IBCB-n40 | Heterorhabditis sp. | Taboporã - SP - Brazil | Inst. Biológico | 80 |
IBCB-n44 | Heterorhabditis sp. | Sta. Adélia - SP - Brazil | Inst. Biológico | 80 |
NEPET11 | Heterorhabditis sp. | Palmeira das Missões - RS - Brazil | Embrapa Trigo | 10 |
When plants reached 10 cm, nematodes isolates were quantified and applied over the vermiculite at the concentration of 100IJs/cm2 (the control treatment received only distilled water), standardizing the percentage of humidity of vermiculite to 20 % (1.4 mL/plastic cup). Afterwards, one of 3rd instar larvae of A. ipsilon was put in each cup, totaling 20 larvae per treatment.
Treatments were kept in climatic chamber at 25 ( 1 °C, RH of 70 ( 10, and 12 hours photoperiod, for five days. After that, the number of dead insects was observed. Dead larvae were dissected under a stereoscopic microscope to confirm mortality by nematodes. The percentage of mortality was calculated in each treatment, considering 20 larvae as 100 %.
Tests with immature stages of A. ipsilon
Susceptibility at different stages (2nd, 3rd, 4th, 5th and 6th instars and pupae) of A. ipsilon was evaluated for the three nematode isolates that presented greater pathogenicity in the selection tests of isolates IBCB-n02 (Steinernema carpocapsae), IBCB-n05 (Heterorhabditis indica) and GL (Heterorhabditis amazonensis).
The test was carried out in 100 mL plastic cups containing 7 g of sterile vermiculite, by placing one larva of A. ipsilon on the surface together with a piece of diet (modified of Greene et al. 1976) for feeding. Each treatment was replicated four times, and each plot corresponded to ten cups containing one larvae/cup. The pupae were buried into the vermiculite without diet. Afterward, nematode suspension was applied with pipette on the vermiculite surface, at the concentration of 100IJs/cm2 (only distilled water was used in the control treatment), standardizing humidity to 20 % (1.4 mL/plastic cups).
Cups were kept in climatic chamber at 25 ( 1 °C, RH 70 ( 10, with 12 hours photoperiod, and the test was carried out in a completely randomized design, in a factorial scheme 4 x 5, considering three isolates and the control (4), and five instars (5). All data were subjected to homogeneity (Hartley) and normality (Shapiro-Wilk) tests to verify the assumptions of parametric statistics. Data were subjected to analysis of variance (ANOVA), and means were compared by the Tukey test at 5 % probability, using the software SISVAR 5.4 (Ferreira 2011).
Concentrations test
The three isolates that proved to be more virulent to A. ipsilon, in the selection test of isolates, were selected (IBCB-n02, IBCB-n05 and GL), using the same methodology of the test with immature stages. However, only 3rd larvae instar was considered, with concentrations of 0 (control treatment), 50, 100, 150, and 200 IJs/cm2.
The test was carried out in completely randomized design, and all data were subjected to homogeneity (Hartley) and normality (Shapiro-Wilk) tests to verify the assumptions of parametric statistics. Data were subjected to analysis of variance (ANOVA) and regression analysis, using the statistical software SISVAR 5.4 (Ferreira 2011) to determine the regression curve and the equation of regression for the interval evaluated in the study.
In vivo production of isolates Steinernema carpocapsae (IBCB-n02), Heterorhabditis indica (IBCB-n05) and Heterorhabditis amazonensis (GL) in A. ipsilon larvae
Plastic Petri dishes (9 cm of diameter) received two filter papers and 10 A. ipsilon larvae, which had been previously weighed. Subsequently, isolates were applied at the concentration of 50 IJs/cm2 in 2 mL of suspension. Petri dishes were tapped and sealed with PVC film and kept in climatic chamber for 72 hours, at 24 ± 1 °C, RH of 70 ( 10, without photoperiod. After confirmation of mortality, larvae were transferred to new Petri dishes containing only one dry filter paper, and kept for five days under the same conditions. Subsequently, larvae were placed in White traps (one plot per trap). IJs were daily collected in suspension of distilled water and quantified for evaluation of the production. Collection and quantification was repeated until larvae production depletion.
Data were subjected to homogeneity (Hartley) and normality (Shapiro-Wilk) tests to verify the assumptions of parametric statistics. Data were also subjected to analysis of variance and to the Tukey test at 5 % probability, using the software SISVAR 5.4 (Ferreira 2011).
Greenhouse test
For the susceptibility test of A. ipsilon under greenhouse conditions, 8 L cup were prepared with soil, at the ratio of 4:1:0.5 soil, substrate (Plantmax®) and vermiculite, totaling 2 L of the mixture of substrates/cup. Five seeds of corn were sown, using the fungicide treatment Maxim XL (fludioxonil 2.5 % + metalaxyl-M 0.1 %) in each plot, and irrigated with 200 mL water a day.
At 10 days after corn emergency, five 3rd instar A. ipsilon larvae were placed in each plot, which were separated into groups of 15 plots/treatment for the application of nematodes isolates (IBCB-n02, IBCB-n05 and GL) in aqueous suspension (100 IJs/cm2) around the plants, using a pipette. Control treatment received only distilled water.
The test was carried out in randomized experimental design. Evaluation occurred at five days after nematodes inoculation, by collecting the dead larvae. Larvae were disinfested with 70 % alcohol and kept in a dry chamber for another three days, when dissection was performed to confirm the death of the insect by the nematode. All data were subjected to homogeneity (Hartley) and normality (Shapiro-Wilk) tests to verify the assumptions of parametric statistics. Data were subjected to analysis of variance (ANOVA) and the Tukey test at 5 % probability, using the software SISVAR 5.4 (Ferreira 2011).
Results and discussion
Selection of entomopathogenic nematodes isolates
Isolate CH3 (Steinernema sp.) presented the highest mortality rate in the selection test (100 %) (Table 1). However, rearing this isolate in the laboratory is difficult. In other words, the isolate infects and kills G. mellonella larvae, but rearing may not occur, which hinders the production of this isolate to be used in nematode tests. Therefore, isolate CH3 was discarded in subsequent tests.
Other isolates caused between 90 % (IBCB-n02, IBCB-n05, GL) and 80 % of mortality (IBCB-n40, JPM 4, RSC 05 and IBCB-n44), and only the isolate NEPET11 did not cause significant mortality (10 % of mortality) (Table 1).
In relation to susceptibility, Ebssa and Koppenhöfer (2012) tested the isolates Heterorhabditis bacteriophora, H. megidis, Steinernema carpocapsae, S. feltiae, S. kraussei, and S. riobrave in larvae of A. ipsilon, and obtained more than 90 % of mortality, except for S. kraussei, which caused only 60 % of mortality. In this work, the present selection test of isolates also reported that most isolates caused more than 80 % of mortality.
This variation of susceptibility for different isolates was expected, since different isolates coevoluted with different species of host, which make them more or less virulent to a determined host (Almenara et al. 2012).
Tests with immature stages of A. ipsilon
The isolates evaluated (IBCB-n05, IBCB-n02 and GL) caused mortality in immature stages of A. ipsilon (2nd, 3rd, 4th, 5th, 6th instar) with similar values to that observed in the selection test, which was performed just with insects in the 3rd stage (Table 2).
Table 2 Mortality rate of different instars of Agrotis ipsilon after the application of isolates IBCB-n05, GL and IBCB-n02 at the concentration of 100 IJs/cm2 under laboratory conditions. Temperature of 25 ± 2 °C, RH 70 ( 10 %, 12 h photoperiod.
Treatments | Mortality rate at different instars | ||||
---|---|---|---|---|---|
2° | 3° | 4° | 5° | 6° | |
IBCB-n05 | 43 ± 8.5 BCa | 77 ± 8.5 Bb | 83 ± 6.3 Bb | 58 ± 7.5 Bab | 60 ± 15.8 Bab |
GL | 48 ± 2.5 Ca | 75 ± 2.9 Ba | 80 ± 5.8 Ba | 60 ± 10.8 Ba | 75 ± 2.9 Ba |
IBCB-n02 | 15 ± 2.9 ABa | 97 ± 2.5 Bd | 83 ± 8.5 Bcd | 50 ± 4.1 Bbc | 45 ± 8.7 Bab |
Control | 0.0 ± 0.0 Aa | 0.0 ± 0.0 Aa | 0.0 ± 0.0 Aa | 0.0 ± 0.0 Aa | 0.0 ± 0.0 Aa |
* Means followed by uppercase letters in the columns and lowercase letter in the rows did not significantly differ by the Tukey test (P ≤ 0.05). C.V. 35.59.
The low mortality in the 2nd instar larvae of A. ipsilon may be due to the low metabolic activity of the larvae of young instars and lower movement, since EPNs located their hosts by the concentration of CO2 around them (Nishimatsu and Jacksn 1998). Thus, the low metabolism may have influenced, since it hinders the contact of the nematode with the larvae, which explains the low mortality.
The 3rd and 4th instars presented the highest mortality rate, reaching 97 % in larvae of the 3rd instar and 83 % in larvae of the 4rd for isolate IBCB-n02 (S. carpocapsae). Ebssa and Koppenhöfer (2012) reported similar results in tests with different instars of A. ipsilon for the species S. carpocapsae, with higher mortality rate for the larvae at the 4th and 5th instars. Conversely, the same authors reported that the 6th instar was less susceptible, differing from the results observed in the present study, in which the lowest mortality rate was obtained in the 2rd instar. Larvae of some Lepidoptera increase susceptibility when they are older; this is because they are bigger, attracting more nematodes, and present more body openings (mouth, anus, and spiracle), making them more susceptible to infective juveniles (Smiths et al. 1994, Shannag et al. 1994). However, in other lepidopteran species, older instars and the pupae are less susceptible (Glazer and Navon 1990; Shannag et al. 1994; Baur et al. 1998). In relation to A. ipsilon, according to Ebssa and Koppenhöfer (2012), the reduced body size of the 2nd instar larvae is the main factor for the low mortality rate, since the small size of the larvae makes it difficult for the IJs to access the larvae’s body.
Pupae mortality rate observed in the test was not significant for any of the isolates evaluated (5 % for IBCB-n02 and 0 % for IBCB-n05 and GL).
Likewise, in the study developed by Chambers et al. (2010) with Cydia pomonella (Lepidoptera: Tortricidae) (apple moth), the pupae were also less susceptible than the larvae, and in the work of Ebssa and Koppenhöfer (2012), mortality rate of A. ipsilon pupae did not surpass 50 %, even for the isolates that caused high mortality rate in the larvae.
Among the alternatives proposed for the low susceptibility of A. ipsilon pupae, it can be suggested that
the lower value of CO2 at this stage may decrease the attraction, or even the displacement the IJ toward the insect (Lewis et al. 1993). In addition, according to Ebssa and Koppenhöfer (2012), A. ipsilon pupae stage occurs in the soil, causing coevolution of this stage together with the EPNs, which could have led to the development of behavioral, physical or physiological defense mechanisms to these entomopathogenic agents.
Concentrations test
All the isolates were pathogenic to A. ipsilon at all the concentrations, with mortality rate between 10 and 100 % (Fig. 1). However, mortality data from the isolate GL did not meet the assumptions of normality and homoscedasticity, and therefore were not subjected to regression analysis. Isolate IBCB-n02 (S. carpocapsae) showed increasing mortality rate of the larvae stage, according to the increase of the concentration used in the test, and CL99 was estimated at a concentration of 140 IJs/cm2. The isolate IBCB-n05 (H. indica) had CL99 estimated at a concentration of 190 IJs/cm2. For both isolates (IBCB-n02 and IBCB-n05), concentrations can be considered as high. This is confirmed with a study developed by Alves et al. (2017), CL99 of the isolate CB40 (Heterorhabditis sp.) for G. mellonella was of 9 IJs/cm2. However, G. mellonella is considered as an insect highly susceptible to most entomopathogenic nematode isolates.
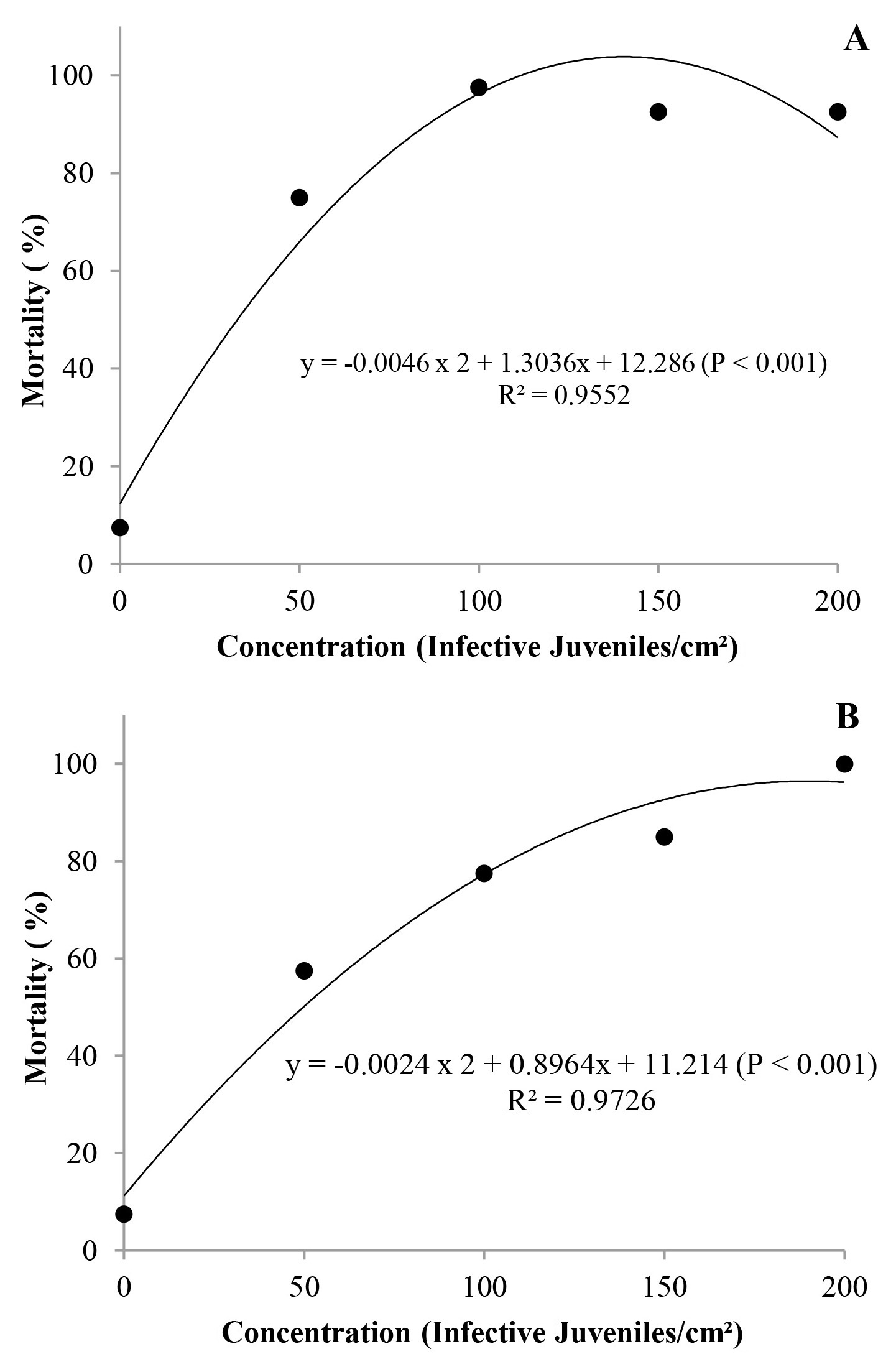
Figure 1 Mortality of Agrotis ipsilon larvae caused by different isolates of entomopathogenic nematodes at different concentrations (IJs/cm2), under laboratory conditions (T = 25 ± 1 °C, RH 90 ± 10 %, and 12 h photoeriod). A. IBCB-n02 Steinernema carpocapsae. B. IBCB-n05 Heterorhabditis indica.
In a study developed by Andaló et al. (2010) with Spodoptera frugiperda (Smith, 1797) (Lepidoptera: Noctuidae), the number of IJs to cause maximum mortality was of 223 IJs/larvae for the isolate RSC02 (Heterorhabditis sp.) and of 218 IJs/larvae for S. arenarium, which are higher values than those observed in this work.
Moreover, according to another study on nematodes for biological control of Lepidoptera, specially S. frugiperda, mortality rate of larvae increased with the increase in the concentration (Polanczyk and Alves 2005), corroborating the present results.
In vivo production of isolates Steinernema carpocapsae (IBCB-n02), Heterorhabditis indica (IBCB-n05) and Heterorhabditis amazonensis (GL) in A. ipsilon larvae
At the end of the production test, the isolates IBCB-n02 (S. carpocapsae), IBCB-n05 (H. indica), and GL (H. amazonensis) produced 4.7 x 10-4 IJs/larvae, 2.3 x 10-4 IJs/larvae, and 3.3 x 10-4 IJs/larvae, respectively. Therefore, no difference was reported between treatments (Table 3).
Table 3 In vivo production of entomopathogenic nematodes (IJs/larvae) in Agrotis ipsilon. Temperature of 25 ± 2 °C, RH 70 ( 10 %, and 12 h photoperiod.
Isolates | IJs/larvae | Mean weight of larvae |
---|---|---|
IBCB-n02 | 4.7 x 10-4 ± A* | 0.26 g |
IBCB-n05 | 2.3 x 10-4 A | 0.24 g |
GL | 3.3 x 10-4A | 0.28 g |
* Means followed by the same uppercase letter in the columns did not significantly differ by the Tukey test (P ≤ 0.05). C.V. 30.95.
No production tests of EPNs in A. ipsilon have been reported yet. Nevertheless, when compared with production data obtained with G. mellonella, the values reported in this work are considered low. Guide et al. (2016) observed that the isolates NEPET11 (Heterorhabditis sp.) and RSC05 (Heterorhabditis amazonensis) presented values of 7 x 10-4 IJs/g larvae and 7.2 x 10-4 IJs/g larvae, respectively. In addition, Bortoluzzi et al. (2013) evaluated two isolates in G. mellonella and observed 2.2 x 10-5 IJs/g larvae for CB24 (Heterorhabditis sp.), and 2.2 x 10-5 IJs/g larvae for CB40 (Heterorhabditis sp.)
Galleria mellonella larvae are susceptible to entomopathogenic nematodes and are frequently used for in vivo production of these nematodes (Gaugler and Han 2002). Conversely, larvae that spend part of their life cycle in contact with the soil, as in the case of A. ipsilon, have defense mechanisms against these agents, which can affect the development and reproduction of EPNs in their interior. Thus, Molina et al. (2004) evaluated the production of three nematodes isolates in four different host (G. mellonella, Tenebrio molitor L. (Coleoptera: Tenebrionidae), S. frugiperda and Bombyx mori L. (Lepidoptera: Bombycidae), and observed great difference in production. In addition, Steinernema glaseri did not rear in T. molitor, S. frugiperda and B. mori, but it satisfactorily reared in G. mellonella. All isolates were able to rear in A. ipsilon, suggesting that the insect has mechanisms of resistance that can influence the rearing of the isolates, but cannot prevent EPNs from reproducing inside the larvae.
According to Stuart (2006), there are differences in relation to the virulence of EPNs, which can be attributed to the specificity, morphology, origin, and even the genetic variability of these isolates.
Greenhouse test
The isolate IBCB-n02 reduced the survival rate of the 3rd instar larvae of A. ipsilon in the soil. Only this isolate differed from the control treatment under greenhouse conditions (Fig. 2).
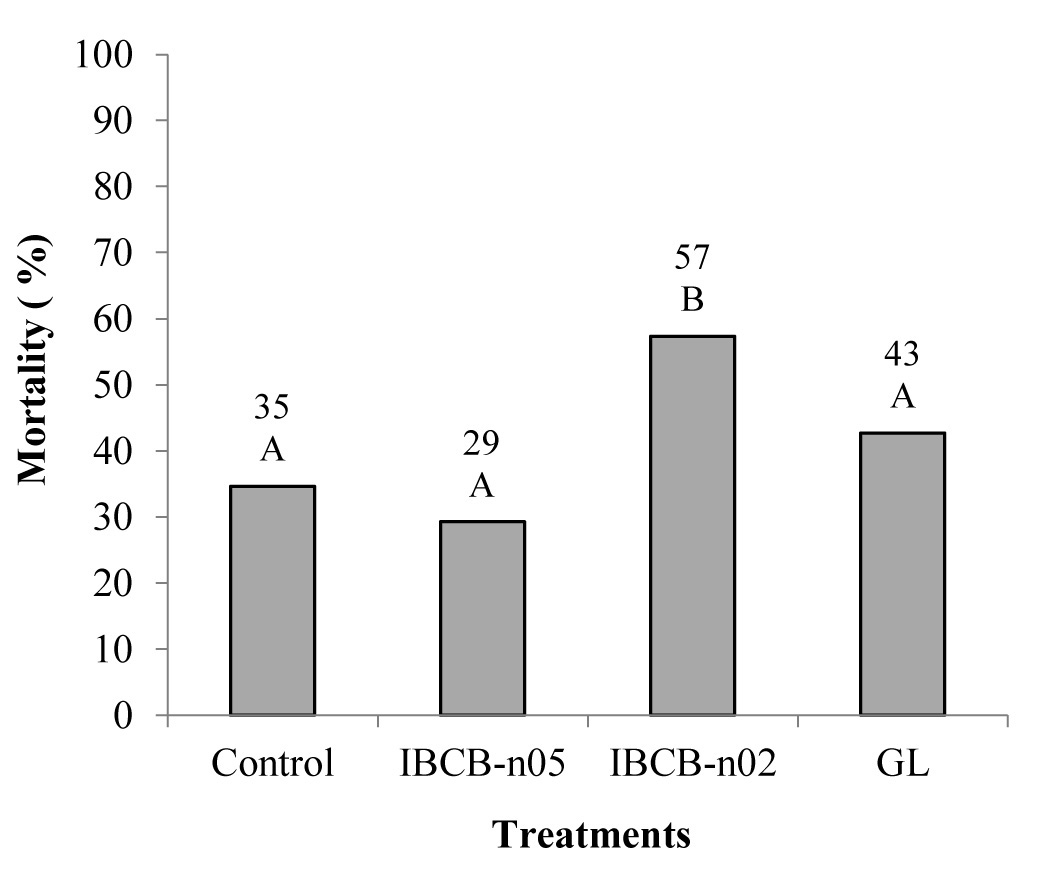
Figure 2 Mortality rate of Agrotis ipsilon larvae caused by different isolates of entomopathogenic nematodes under greenhouse conditions. Columns followed by the same uppercase letter did not significantly differ by the Tukey test (P ≤ 0.05). C.V. 63.24.
During the experiment, larvae caused damage to the corn plants by cutting the stems, at not only in control, but also in the nematodes treatments.
These results can be compared with those obtained by Riga et al. (2001), who tested two isolates of Steinernemaagainst four corn pests, being one of the the noctuidae S. frugiperda, under greenhouse conditions. The authors reported that these isolates decreased the damage against corn plants, when compared with the control treatment (without nematode).
In a study developed by Ebssa and Koppenhöfer (2012), the five isolates (H. megidis, H. bacteriophora, S. carpocapsae, S. riobrave and S. feltiae) caused mortality in A. ipsilon under greenhouse conditions, both in larvae at the 3rd and 5th instars. According to the authors, the highest mortality rates were obtained at concentrations of 200IJs/cm2, which corresponds to twice the concentration used in this work. According to these authors, the time of evaluation was determinant in the mortality rate, and more significant values were observed when the evaluation was carried out at seven days after inoculation.
Factors such as temperature influenced the infectivity of the entomopathogenic nematodes. Moreover, species react differently, i.e., Heterorhabditidae are more sensitive to temperature than Steinernematidae, which might have caused lower mortality rate in the treatment with Heterorhabditis (Flanders et al. 1996).
In the present study, only the isolate IBCB-n02 (v) was able to cause significant mortality in the greenhouse test, which reinforces this statement. Results suggest that this isolate stood out in relation to GL and IBCB-n05, which are of the family Heterorhabditidae.