Introduction
The flagellate protozoan Trypanosoma cruzi (Trypanosomatida: Trypanosomatidae) is the causative agent of Chagas disease, an endemic zoonosis in 21 countries in Latin America, from the southern United States to northern Argentina and Chile, and is considered the most serious parasitic disease in this hemisphere (INSP, 2021; Rojo-Medina et al., 2018). Vector-borne transmission occurs exclusively in the Americas, with an estimated 6 to 8 million people infected and a mortality of 22,000 people per year (Ceccarelli et al., 2020; de Fuentes-Vicente et al., 2018; Chagas disease in Latin America: An epidemiological update based on 2010 estimates, 2015).
Triatomines are important natural vectors of T. cruzi; they are hematophagous insects of the family Reduviidae, subfamily Triatominae, whose most representative genera are Rhodnius and Triatoma (Estay-Olea et al., 2020; Miles et al., 2003). Out of 31 triatomine species recorded in Mexico, 19 have been found to be naturally infected with T. cruzi (Cruz-Reyes & Pickering-López, 2006). There are three main stages of differentiation in the parasite, i) amastigote, ii) trypomastigote and iii) epimastigote, of which, in the vertebrate host, the trypomastigote and amastigote stages can be found, while in the insect the parasite can be identified mainly as epimastigote and metacyclic trypomastigote (Buekens et al., 2013; De Fuentes-Vicente et al., 2019; Miles, 2004).
Both Triatoma barberi (Usinger) and Triatoma longipennis (Usinger) (Hemiptera: Reduviidae: Triatominae) are considered important vectors of T. cruzi in Mexico, due to their presence in domestic and peridomestic habitats, their wide geographic distribution and their high infection rate (Brenière et al., 2010; Martinez-Hernandez et al., 2021; Rivas et al., 2018; Salazar Schettino et al., 2005). Similar to most reduviids, both species have five nymphal stages in addition to the adult stage, and males and females of all stages can be infected with T. cruzi, and transmit Chagas disease (Velásquez-Ortiz et al., 2022).
Several studies have shown that some biological parameters in the triatomine can be affected by the parasite, deactivating defense mechanisms in the vector to infect its definitive host (Córdoba-Aguilar, 2020; Ramírez-González et al., 2019).
Parasitic infection causes negative effects on the survival of other triatomine species such as Rhodnius prolixus Stål (Hemiptera: Reduviidae: Triatominae), as reported by Peterson et al. (2016), who reported that reduviids had lower survival than the control groups. In terms of development, a difference is also seen between infected and uninfected insects, as mentioned by Elliot et al. (2015), where T. cruzi infection caused a considerable delay in the time to molt. Also, changes in the feeding and defecation patterns of the vectors have also been reported (Botto-Mahan et al., 2006). In this case, T. cruzi infection reduced the time to host infection in Mepraia spinolai (Porter) (Hemiptera: Reduviidae: Triatominae); infected bugs bit about twice more often than uninfected nymphs and defecated 8 min after the last blood meal whereas uninfected bugs needed 11 min (Botto-Mahan et al., 2006).
Another example of the effects of the parasite on defecation patterns was reported in the work of Pereyra et al. (2020), in which insects of the species Triatoma infestans Klug, infected with T. cruzi defecated earlier and in greater quantities compared to uninfected insects.
In addition, T. cruzi is capable of modifying the development and body size of its vector, both in males and females (Antonio-Campos et al., 2019), as Botto-Mahan (2009) reported in her study that infected insects had delayed development, reduced survival and lower weight, compared to non-infected insects, similar to that reported by Cordero-Montoya et al. (2019) who reported that T. cruzi infection affects the same biological parameters in T. pallidipennis (Stål) (Hemiptera: Reduviidae: Triatominae).
In terms of fecundity and reproduction, Botto-Mahan et al. (2017) reported negative effects of T. cruzi infection in M. spinolai, in which uninfected females produced more and heavier eggs when crossed with uninfected than infected males. Uninfected males, in turn, sired more eggs and nymphs when crossed with uninfected than infected females. Another study also evaluated this biological parameter, where T. cruzi infection in R. prolixus increased the e-value in the second reproductive cycle, as well as hatching rates (Fellet et al., 2014).
Trypanosoma cruzi can also affect sexual dimorphism, due to hormones that may serve as communication signals (Alavez-Rosas et al., 2023) as reported by May-Concha et al. (2021), who studied the infection of T. cruzi in Triatoma dimidiata Latreille (Hemiptera: Reduviidae: Triatominae), and when measuring the antennae sensilla, they reported that infected insects have a greater perception of contact stimuli and olfactory perception than uninfected insects, which favors vectorial transmission of the parasite.
Although the effects of T. cruzi on the development and survival of different species of triatomine bugs are known, it is not fully understood how the parasite affects many of the species that inhabit Mexico, such as T. barberi, one of the principal species that transmits the parasite (Rivas et al., 2018). Hence, this study aimed to know if T. cruzi affects the survival and development of this species, both males and females, comparing it with T. longipennis, the latter is one of the most abundant in Mexico (Brenière et al., 2010; Martinez-Hernandez et al., 2021).
The results shown here could allow us to better understand the interactions between parasite and vector and even to apply methods to control their transmission and thus avoid infection.
Materials and methods
Origin of insects and parasites
Triatomines were collected manually from two municipalities of Hidalgo State, Mexico. Triatoma barberi from El Ahorcado (municipality of Tecozautla, 20°29′N, 99°28′W, 2,070 m a.s.l.) and T. longipennis from El Pirú (municipality of Metztitlán, 20°31′N, 98°47′W, 1600 m a.s.l.). (Becerril-Flores et al., 2007, 2010). Both, collected T. barberi and T. longipennis were put in plastic vials; specimens were classified according to the keys of Lent and Wygodzinsky (1979). The T. cruzi strain used was isolated from T. barberi collected at Tecozautla, Hidalgo, as described by Becerril-Flores et al. (2007). The strains were maintained by cyclical passages in adult CD-1 mice.
Feeding and maintenance.
The research was carried out at the Microbiology and Parasitology Research Laboratory, Institute of Health Sciences at the Universidad Autónoma del Estado de Hidalgo (UAEH) in Pachuca, Hidalgo, México.
The experiment was carried out with triatomines of both species, T. barberi and T. longipennis. In the case of T. barberi, infected (n = 30) and uninfected (n = 30), five nymphs for each one from the second to fourth instars, five females and five males were used. On the other hand, for T. longipennis, infected (n = 15) and non-infected (n = 15) nymphs were used, five for each one from the second to the fourth instar. Each insect was 5-10 days old since the last molt. It is important to clarify that studies were not carried out with the fifth nymphal and adult stages for T. longipennis because they did not resist infection in the first 10 days and died, therefore they could not be quantified.
The triatomines were kept inside transparent plastic containers with a circular section (4 x 7 cm) within folded cardboard at the bottom and another cardboard folded like a fan that allowed the insect to walk upwards and covered with a mesh to facilitate feeding. Specimens used were maintained for 90 days under the following laboratory conditions: Incubated at temperature of 28 °C and 40 %-60 % humidity (Figure 1).
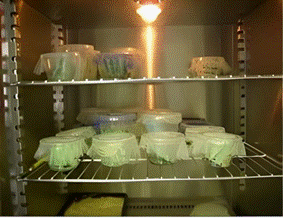
Figure 1 Maintenance of the T. barberi colony with periods with humidity of 40 %-60 %, the temperature of 28 °C, and feeding was performed weekly, with mice as a host.
In order to know whether T. cruzi affects triatomines, the insects were starved seven days before experimentation, then, the open end of the container was placed on the belly of a CD-1 strain mouse with known T. cruzi parasitemia, allowing the insect to access the mouse skin and feed ad libitum.
Insects were weighed before and after feeding with blood from infected mice, in order to determine the amount of blood ingested by each vector. Once the density of the mouse blood was known, the number of parasites that each triatomine ingested could be determined. For this purpose, a cut was made at the tip of the mouse tail in such a way that 10 μl of the blood was collected by weighing it with an analytical balance. This procedure was performed nine times to obtain an average and thus determine the density of the blood; likewise, to determine the parasitemia presented by the mouse, 10 μl of blood were collected and diluted with 40 μl of 0.85 % ammonium chloride and left to stand for five minutes to favor the lysis of the erythrocytes and to be able to make a better observation of the parasite. Then, this suspension was poured into a Neubauer chamber to quantify the parasites.
Metacyclogenesis determination.
The presence of T. cruzi trypomastigotes in the feces of each insect called “metacyclogenesis” was carried out as described by Becerril-Flores et al. (2007). Briefly, after seventh day after feeding and every third of fourth day until the 90th day of incubation, parasitic colonization in the midgut was determined by pressing with dissecting forceps on the abdomen to induce defecation and the feces collected on the slide were observed under a light microscope by staining with Giemsa for identification of T. cruzi stages.
The number of dead insects, time to molt, and percentage of triatomines molting were recorded. These groups of reduviids were compared with insects that were not infected with T. cruzi which served as controls throughout the experiment.
Results
Effect of the development of Triatoma barberi and Triatoma longipennis by the presence of Trypanosoma cruzi.
To determine whether T. cruzi affects the development of insects, they were infected at different stages. The results of metacyclogenesis from T. barberi and T. longipennis are shown in Figure 2, where the number of parasites in the feces over 90 days was recorded. All the triatomines studied were infected with T. cruzi in both species. For T. barberi, the number of parasites in the feces reached a maximum peak between 20 and 40 days of infection with 8-12x106 parasites per ml and after this day until day 90 they did not exceed 3x106 per ml; while in T. longipennis the metacyclogenesis began from day 20 in an ascending way until day 90 or their death which allows us to say that the species T. longipennis was more affected by infection than T. barberi.
Presence of the different phases of T. cruzi on the development of triatomines.
The results of the effect of infection by different stages of T. cruzi on T. longipennis at different stages of development are shown in Figure 3. The epimastigote was found in the highest quantity, in about three to four times more than the trypomastigote in all stages of the triatomines studied. In the case of T. longipennis, although epimastigotes were found in greater quantity than trypomastigotes throughout the infection in the three stages, it can be observed in Figure 4 that the quantities were very close to each other, especially in the second and fourth stages, but in the third stage there was a greater difference between these two stages of T. cruzi, around 20 %. These results allow us to say that regardless of the species of triatomine, at least for the two species studied, the elimination of epimastigotes through feces would lead us to think that they are not of much risk since the infecting phase is the trypomastigote; however, this does not mean that there is no risk of infection since in any case the trypomastigotes are also eliminated in both species of triatomine.
Molting time and molting in Triatoma barberi and Triatoma longipennis.
The time to molt is shorter in those triatomines that were not infected with T. cruzi for both species, T. barberi, and T. longipennis; when the insects were infected, time to molt never could be observed in T. longipennis, and for T. barberi was between 28 to 42 days.
The results shown in Table 1 according to ANOVA show statistically significant differences between infected and uninfected triatomines in both T. barberi and T. longipennis.
On the other hand, all the triatomines could molt when they were not infected whereas all of them could not molt when they were infected. For T. barberi the percentages of triatomines that could molt were similar.
Finally, the time that T. barberi died was shorter in insects infected than the non-infected with T. cruzi. In contrast, uninfected T. longipennis, did not die during the experiment, while the infected ones died 68 days post-infection.
Thus, T. cruzi infection affects the development and survival of triatomines in both species, being greater in T. longipennis than T. barberi.
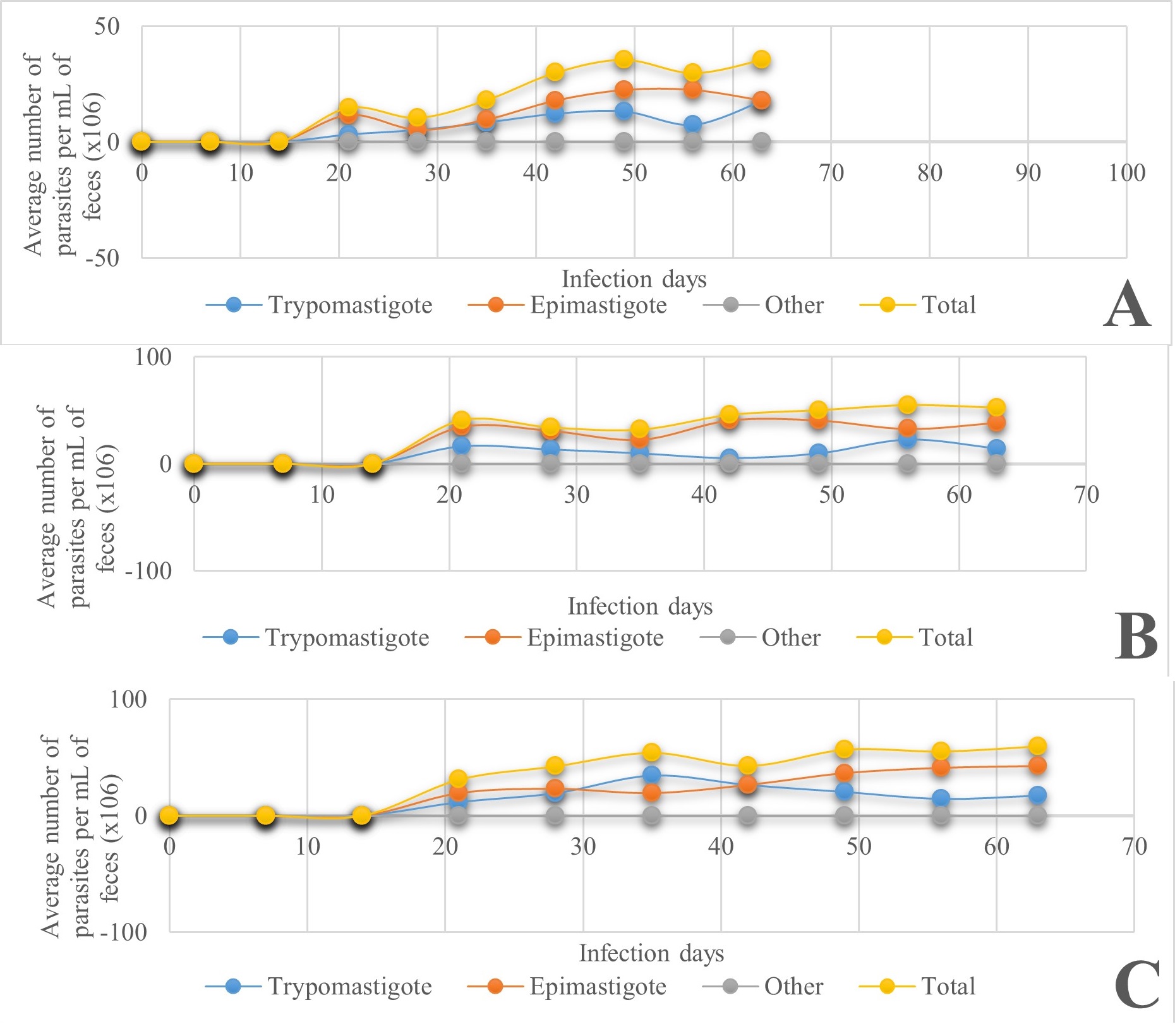
Figure 3 Kinetics of metacyclogenesis in different phases of T. cruzi, present in T. longipennis in A) 2nd-instar nymphs, B) 3rd-instar and C) 4th-instar nymphs.
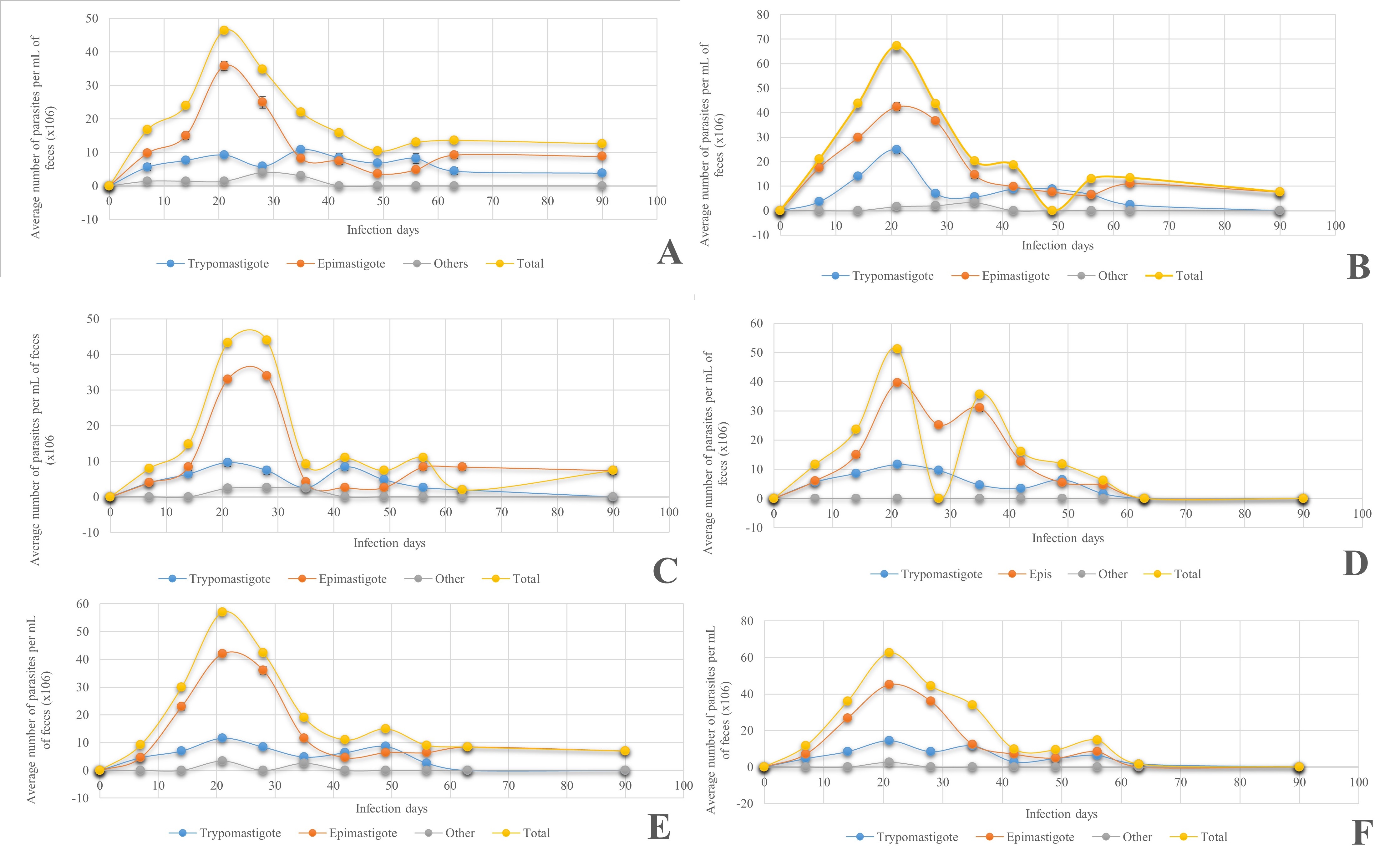
Figure 4 Kinetics of metacyclogenesis in different nymphal stages of T. cruzi, present in T. barberi in A) 2nd-instar nymphs, B) 3rd-instar nymphs, C) 4th-instar nymphs, D) 5th-instar nymphs, E) Females (♀) and F) Males (♂).
Table 1 Molting period of each stage of T. barberi and T. longipennis, infected and uninfected.
Infected | Not infected | ANOVA of molting period data rom infected and not infected triatomines | ||||
---|---|---|---|---|---|---|
Species | Instar | Molting period (days) | Molting percentage (%) | Molting period (days) | Molting percentage (%) | F, p |
T. barberi | 2nd | 35+ 1.2 | 100 | 30+1.32 | 100 | 78.4, p<0.05 |
3rd | 42.4+1.02 | 60 | 35+0.63 | 60 | 64.18, p<0.05 | |
4th | 28.2+0.75 | 80 | 20+1.67 | 100 | 126, p<0.05 | |
5th | 42.4+ 1.02 | 100 | 30.6+ 1.35 | 80 | 197.3, p<0.05 | |
T. longipennis | 2nd | NM | 0 | 60 + 0.75 | 60 | 871.8, p<0.05 |
3rd | NM | 0 | 65+ 1.02 | 100 | 1250, p<0.05 | |
4th | NM | 0 | 65+ 0.75 | 100 | 1443, p<0.05 | |
5th | NM | 0 | 63+ 1.2 | 80 | 2439, p<0.05 |
NM: No molting is considered until 90 days.
Discussion
This work aimed to know whether the protozoan T. cruzi is capable of modifying the biological parameters of triatomines, such as their development and survival, since these, being insect vectors, play an important role in the transmission of the parasite.
We found that mortality in both species of triatomines, T. longipennis and T. barberi, was greater in infected than in uninfected insects, as predicted, and was higher in T. longipennis than in T. barberi. Triatomines of both infected species died throughout the experiment, unlike uninfected ones; in T. longipennis mortality occurred earlier than in T. barberi. In both cases the parasites affected the triatomines, causing death. Triatoma longipennis was more affected, mortality was higher, and it took longer between molts, probably due to a higher infection in this triatomine species than in T. barberi, which had a lower mortality and a shorter time between molts.
This result was also observed by Loza and Noireau (2010), who made a comparison of the vectorial capacity between Triatoma guasayana Wygodzinsky & Abalos and T. infestans, the colonization capacity is different for each stage and each species of triatomine. In another study, Cordero-Montoya et al. (2019), reported that the affectation of triatomines of the species M. pallidipennis is due to infection by T. cruzi strains from Morelos and Chilpancingo, in which infected insects laid fewer eggs than the uninfected control group.
As shown in the results, it can be observed that the fifth-instar stage of T. barberi is the most affected by T. cruzi. In this case, its size is larger compared to the other stages, and this is explained because the midgut is larger in the more advanced stages of this species of triatomine; therefore, during feeding time, they ingest more parasites than other stages since it needs more energy to molt.
A factor that also influences molting time and therefore development time is the fact that the triatomines are infected by T. cruzi. In this work it was observed that infected specimens took more days to molt compared to uninfected ones, this can be explained because trypanosomes may obstruct the epithelium of the intestine of the insects and therefore serve as a barrier that interferes with the absorption of their food, or perhaps the parasites induce a reaction in the insect reducing their feeding, since in these infected bugs their weight and size were reduced, as reported in the works of Cordero-Montoya et al. (2019) and Botto-Mahan (2009), in which infected insects were reported to have delayed development and lower weight.
The effects in terms of survival and development in infected parasites compared to those not infected by T. cruzi can be explained by the "manipulation hypothesis", which, according to Córdoba-Aguilar (2020) and Ramírez-González et al. (2019) explains how the parasite deactivates defense mechanisms in the vector, in order to infect the definitive host.
Trypanosoma cruzi infection in triatomine bugs has implications for vectorial disease transmission, due to its effect on other biological parameters such as feeding and defecation patterns, as reported by Pereyra et al. (2020), where specimens of T. infestans infected with T. cruzi defecated earlier and in greater quantities compared to uninfected specimens. Vectors defecate during or immediately after feeding, suggesting that the greater the defecation, the greater the probability of transmitting the parasite, because if the volume of excrement increases, the insect will release more parasites.
The following questions now arise: Physiopathologically, why are triatomine insects affected in their development by the presence of T. cruzi? Some hypotheses could be the presence of different substances produced by the parasite that affect the physiology of the insect, the large number of parasites that physically obstruct the mechanisms involved in the development of the vectors, the fact that they can mechanically damage some of the metabolically important structures for triatomines, among other conjectures that may arise. This makes it necessary to continue investigating this aspect of the infection inside the vectors, to implement strategies that help prevent the parasitic transmission of T. cruzi and thus Chagas disease.
Conclusions
The results of this work showed that the presence of T. cruzi affects biological parameters such as the development and survival of both, T. barberi and T. longipennis, in their different stages, although this affection is greater for the latter species, which is explained by the higher parasite load observed in it and in most of the affected stages of each species of triatomine. Trypanosoma cruzi also affects the ecdysis or molt of triatomines causing their death. These conditions suggest that the parasite deactivates defense mechanisms in the vector in order to infect its definitive host. Finally, it is necessary to continue with research related to the infection of insects that act as vectors of parasites to generate strategies to reduce disease transmission.