Rapidly and accurately detecting events in the environment is fundamental for survival. It allows human and non-human animals to identify and locate con specifics, shelter, food sources, threats, and potential sexual partners. This process is supported by sensory receptors for detecting features of the environment and by memory of past experiences, which allow for more adaptive future interactions (Ruetti et al., 2009).
One type of memory, working memory (WM), involves several brain regions that interact to direct attention, maintain the activity of recent or retrieved information, and integrate the information necessary for completing a task (Baddeley, 2003). Rather than a single system, WM can be broken down into separate subsystems. The phonological subsystem, also called auditory working memory (AWM), temporarily stores and rehearses auditory information. The identity and spatial properties of visual objects are stored and rehearse din the visuospatial sketchpad subsystem, commonly referred to as visual working memory (VWM). The hypothesis ed independence between these systems suggests that performance in tasks that engage one system should be less affected by subsequent tasks that engage a different subsystem, since WM is not a unitary resource.
The independence of AWM from VWM has been supported using selective interference paradigms, such as articulatory suppression. In an articulatory suppression procedure, participants perform a secondary task to prevent rehearsal in AWM, such as repeating a word out loud (e.g., “the”), while performing a primary AWM task, such as verbal reasoning. Articulatory suppression has been shown to disrupt tasks utilizing AWM more than those using VWM (Hitch & Baddeley, 1976). Secondary tasks that involve visuospatial judgment shave a greater disruptive effect on other tasks utilizing VWM rather than AWM. Furthermore, it has also been proposed that the visual (e.g., colour, shape, size, etc.)and spatial (e.g., location) properties of objects are processed independently, but there is much less research investigating this separation (Logie, 1995).
Logie and Marchetti (1991) evaluated the independence of visual and spatial WM using two primary and secondary tasks. The colour memory primary task required participants to make a judgement regarding a change in the colour of any of the objects between two sequentially presented arrays of squares. In the spatial sequence primary task, participants were asked to observe a pattern of six squares presented one at a time, at a rate of one per second. Another pattern of six squares was then presented, and participants were asked to identify whether the patterns were the same or different. A 10-s retention interval was included in both primary tasks, during which participants we reinstructed to complete a secondary task. The secondary task consisted of observing a sequence of unrelated images, tapping with their right hand, or doing nothing. Results showed that participants performed worse in the colour memory task when they were exposed to a sequence of irrelevant pictures than when tapping, but performance in the spatial sequence task was disrupted more when they were required to tap with their right hand. These results are consistent with additional evidence supporting some degree of independence between visual (visual cache) and spatial (inner scribe) WM (Jiang et al., 2000).
Selective interference procedures have demonstrated a disruption of memory for one property (e.g.,spatial) more than another (e.g., visual). Other procedures, such as priming, improve WM. Priming occurs when prior experience with a stimulus influences the response to a subsequent presentation of the same or similar stimulus, as a result of the degree of activation of particular associations in memory (Kristjánsson &Campana, 2010). This activation can allow participants to respond faster to the stimulus that has been primed, increase the accuracy of their identification, or both(Wiggs & Martin, 1998). Priming procedures typically include repeated presentations of a target stimulus in the same location (Maljkovic & Nakayama, 1996), or with the same identity properties (e.g., colour; Maljkovic & Nakayama, 1994). Previous evidence has also demonstrated that processing a specific property of an object (e.g., identity or spatial) can be improved by using priming procedures. Participants show faster reaction times (RTs) when identifying the object in that position or with those same identity features around it. Using a task with multiple distractors, Kristjánsson et al. (2002) found that RTs in a visual search task were shorter when the target and distractor identities stayed constant across trials (Kristjánsson & Asgeirsson, 2019).
Another procedure, associative priming, involves presenting a cue before (pre-cueing) or after (retro-cueing)a target. Evidence indicates that retro-cueing improves performance to a similar degree as pre-cueing (Fu et al., 2022). In a seminal study, Griffin and Nobre (2003) shaded different corners of a box presented in the middle of a display to cue which of four objects (position outside of each corner of the box) would be the target for a subsequent same-different judgement. Compared to trials in which all corners were shaded (i.e., nocue), presenting a shaded corner as a pre or retro-cue resulted in shorter reaction times (RTs) and higher recognition accuracy. Cue reliability and visual interference have been shown to influence the effectiveness of cueing (Gunseli et al., 2015; Janczyk & Reuss, 2016).
Cueing the most relevant property of an object in a visual task improves visual processing, however, cueing the wrong property can disrupt visual search. In a letter naming task, Engle et al. (1995) asked participants to read aloud a letter of a given colour, while ignoring an overlapping letter of a different colour. The results showed that when the overlapped letter was presented on a subsequent trial as a probe (letter to be read),the RT slowed significantly. This effect of slowing the processing of a probe stimulus that has been used as a distractor in a previous trial is called negative priming (Egner & Hirsch, 2005; Fanini et al., 2006; Tipper, 1985). Interestingly, it has not been tested whether negative priming can occur when testing different properties of objects within the same trial.
Visual processing is impaired by a distractor stimulus or a secondary task inserted between the on set of the target and the probe. Although cueing and selective interference procedures have evaluated the effect of stimuli presented after the target, it is not clear what effect cueing a distractor might have on WM for the target; particularly, if the cue is signaling that the participant should attend to one property (identity or spatial) of the distractor. Therefore, the goal of the current study was to determine whether inserting a priming stimulus that signals an identity or spatial change before a distractor stimulus would selectively interfere with, or facilitate, WM for the identity or location of the target stimulus in a change detection task (CDT).
Experiment
Objects inserted between the presentation of a target and a probe stimulus can interfere with WM and disrupt change detection performance (Washburn & Astur,1998). However, an object inserted between a target and probe that is trained as a retro-cue can facilitate WM for the target. In the current experiment, participants were trained with different cues (coloured backgrounds) that could occur before (pre-cue) or after(retro-cue) a target stimulus (snowflake). The identity or spatial location of the target must be compared to a probe stimulus to determine the correct response. Attest, the retro-cue was followed by a distractor image (a novel snowflake). Based on a selective interference account, if the cue (e.g., green) that precedes the distract or signalled the importance of the identity property during training, for example, then it should lead to more processing of those properties of the distractor and interfere with WM for the identity properties of the target. Little-to-no interference should occur on a trial in which the distractor is preceded by a cue that signalled the importance of a property (e.g., spatial) not being tested on that trial. If a novel cue preceded the distractor, it would result in partial interference because participants would attend to both the identity and spatial properties of the distractor. In sum, a selective interference account predicts that the distractor image will result in the highest interference when the cue preceding it signals the same property of the target object being tested on that trial (e.g., an identity pre-cue followed by the target, and then presented again before a distractor image), moderate interference when preceded by an untrained cue, and the lowest interference when preceded by a cue associated with the property not being tested on that trial (e.g., spatial location). Lower performance would be represented by longer RTs and lower, or even negative, sensitivity on d’ scores.
In contrast to the interference account, the cue preceding the distractor may only operate as a retro-cue for the target because the cue was presented before the distractor, but after the target stimulus. Thus, there exists the potential for the cue to act solely as a retro-cue for the target to improve WM for it. In fact, one explanation for the source of the retro-cueing effect is that it protects the target stimulus from interference (Brady & Hampton, 2018; Fu et al., 2022). If the cue functions solely as a retro-cue for the target stimulus, then the cue preceding the distractor that signals the same property being tested on that trial (e.g., identity) should enhance the processing of the target (i.e., as a retro-cue).This would result in faster RTs and higher d’ values on these trials relative to trials in which the cue signals the other property (e.g., spatial) or in trials where the cue was a neutral stimulus that did not signal a particular property.
The two accounts (selective interference and retro-cueing) make opposite behavioral predictions for cues presented before the distractor that signal the same, a different, or no relevant property as the one signalled by the pre-cue. In the current task, two coloured cues (one signalling an identity test and the other signalling a spatial test) were included twice as often as pre-cues than as retro-cues, which is likely to result in a bias toward the cue acting as a pre-cue for the distractor. Given the design of the current experiment and robust evidence of selective interference in the extant literature, it was expected that behavior would better match predictions of interference.
Method
Participants
The minimum required sample size was estimated using an a priori power analysis for a RM-ANOVA on G*Power 3.1 (Faul et al., 2009). Given an expected moderate effect size (f = .25), two (property) by four (interference)by two (response) within-subjects factors, and statistical significance of a = .05, the power analysis revealed a minimum required sample size to achieve95% power of n = 15. A total of 22 young adults (14 females) between the ages of 18-23 (19.8 ± 1.22) were recruited from the University campus through the SONA Psychology Research Participation System. All of them reported normal or corrected to normal vision and hearing in a demographics survey at the beginning of the experimental task. Participants who did not meet those criteria were excluded from the study. The Institutional Review Board (IRB) approved all materials and procedures.
Stimuli
Participants carried out a CDT on a computer HP Elite One, equipped with a 21” monitor at 1080p resolution, an Intel Core i5-8500 processor, 16 GB of RAM, and running Windows 11 Enterprise. Headphones were provided by researchers.
A set of 31 (15 on training, 16 on test) white snowflakes(192 x 192 px) were used as stimuli. Snowflakes were selected given the complex visual features that make them hard to encode verbally. The experimental task was programmed on Psycho Py v2021.2.3 (Peirceet al., 2019). All of the data, data analysis scripts, and experimental code is stored in the following repository: https://osf.io/wa4ej/?view_only=5227b6cee96b48cca4dbdadc802f44d4
Procedure
Participants were welcomed to the experimental room and told to sit comfortably in front of the computer. After being debriefed regarding the study, participants consented and were asked to fill out a background survey. At the beginning of the experimental task, they were provided with information regarding the task, such as the association between the property evaluate din each trial and colour background (e.g., green for identity), the neutral backgrounds, and the auditory feedback for correct and incorrect responses.
Each trial started with the presentation of a coloured background (CB1) for 1,000 ms, followed by the target stimulus (snowflake) for 2,000 ms. A second coloured background (CB2) was presented for 1,000 ms, followed by a crosshair for 2,000 ms. The offset of the crosshair was followed by a 1,000-ms empty interval and then the probe stimulus for 3,000 ms. Participants responded “same” or “different” depending on whether the probe matched the target in the dimension signalled by CB1. Once the participant responded, the background turned white for 2,000 ms, signalling an inter-trial interval (ITI).
All of the stimuli and the crosshair were presented on a black background (Figure 1). Target stimuli were presented 192px away from the centre of the screen, at 0, 45, 90, 135, 180, 225, 270, or 315 degrees. Crosshair and distractor were presented always in the centre of the screen. The CB signalled which stimulus property should be used to evaluate whether the target and probe stimuli matched, such as a green CB to evaluate the identity (Id) or visual property, and a yellow CB to signal a comparison of spatial location (Sp). A grey CB after the target was used as a neutral cue (Nt). Identity changes consisted of different stimuli, while spatial changes consisted of a change of 96px distance relative to the centre of the target stimuli, at 0, 45, 90, 135, 180,225, 270, or 315 degrees.
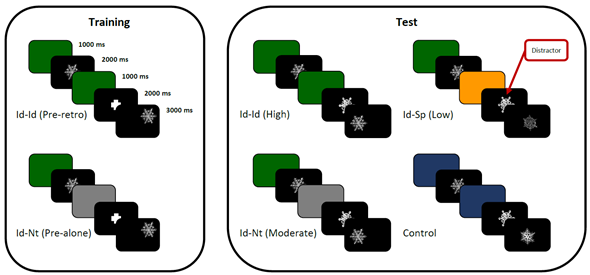
Note. Trials of Id-Id and Id-Nt in Training and Test with a correct response of “same”. The spatial trials had the same configuration, but with a yellow cue instead of green. The test trials for Id-Sp and Control shown have a correct response of “different”.
Figure 1 Schematics of the experimental task for the identity trials
A session included 216 trials split into training and test phases (Table 1). Training consisted of 120 trials presented in sequential blocks, starting with 10 Id-Id,then,10 Sp-Sp, and 20 intermixed of the two. Next, 10 trials of identity-neutral (Id-Nt), followed by 10 spatial-neutral (Sp-Nt), and 20 intermixed of the two. Finally,40 intermixed trials of the four types (10 of each)were presented. Trials where CB1 and CB2 signalled the relevant cue were named pre-retro, whereas trials where CB1 signalled the relevant cue and CB2 was neutral were called pre-alone. The pre-retro trials were included to provide participants with experience before testing of completing the comparison signalled by the pre-cue (e.g., identity) when a cue following the target was the same (pre-retro) or different (i.e., pre-alone). Auditory feedback was delivered following correct and incorrect responses. There was an equal number of same and different trials.
Table 1 Number and type of trials used in each phase of the study
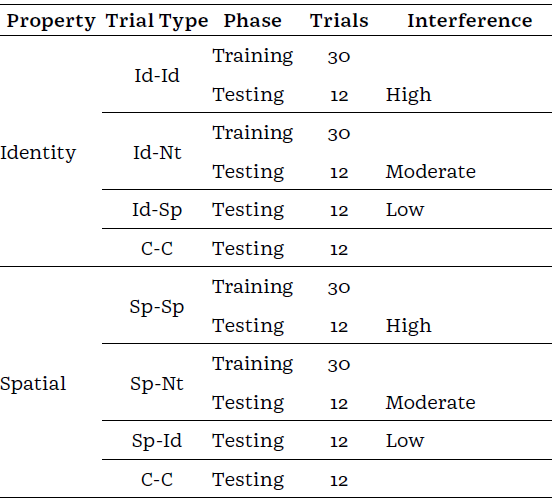
Note. Each pair of letters in the trial column stands for the first and second cue presented respectively, being Id for identity, Sp for spatial, Nt for neutral, and C for control of the property evaluated. Given interference is a Test condition induced by the distractor, Id-Id and Sp-Sp are called pre-retro trials, and, Id-Nt and Sp-Nt, are pre-alone trials.
At test, the crosshair was replaced with a distractor snowflake. The test phase included different combinations of CB1 and CB2 to create different levels of interference (see Table 1). This resulted in the same pre-retro and pre-alone trials from training, along with new trials of Id-Sp, Sp-Id, and novel CB (Figure 1). Four novel distractor snowflakes were used in 96 trials with 12 of each level of interference intermixed. Auditory feedback was not delivered during testing. A resting period of 1 min was included between the two phases. Reminder instructions were presented to the participants before starting the test phase.
Data analysis plan
RT was analysed by calculating the mean of the median of reaction times, excluding error and no-response trials (Brady & Hampton, 2018). d’ scores were calculated using the normalised proportion of hits (responding “different” when the probe stimulus was different to the target) minus the normalised proportions of false alarms (responding “different” when the probe stimulus was the same as the target; Macmillan & Creelman,1992). No-response trials were excluded. A loglinear correction was used to adjust values to infinite when participants exhibited a perfect performance (Hautus,1995). For the training phase, only the last 40 trials were included in the analysis.
All the analyses were performed with R v4.3.1, using the latest available version of the packages Tidyverse (Wickham et al., 2003) and rstatix (Kassambara, 2020).Repeated measure analysis of variance (RM-ANOVA) was conducted on RT and d’. All pair-wise comparisons were performed with Bonferroni’s correction.
Results
Reaction time
A 3-way RM-ANOVA with a 2(Property: Identity vs Spatial),by 2(cueing: pre-alone vs pre-retro), by 2(response: same vs different) factors was conducted on RT on Training. Results did not show any significant main effects or interactions (ps ≥ .274); a trend was found in property, F(1,21) = 3.84, p = .064, n2 p = .155, suggesting a slightly faster average RT in spatial (.90 sec ±.34 sec)compared to identity trials (.95 ±.34 sec).Similarly, a 3-way RM-ANOVA with a 2 (Property: Identity vs Spatial), by 4 (interference: high, moderate, low, control), by 2 (response: same vs different) factors was conducted on test trials, with no significant main effects or interactions (ps ≥ .181), although, a trend was found in a 2-way interaction between property and interference(3,39) = 2.63, p = .064, n2 p = .168. While identity high (.94 ±.28 sec) and moderate (.95 ±.34 sec) interference trials showed similar values, spatial high (.91 ±.31sec) interference trials had RTs that were slightly fasterthan moderate (1.08 ±.40 sec) interference trials.
d’ scores
Correct responses and false alarms were used to calculate the d’ scores. Numbers closer to zero mean participants were responding at chance, while positive values suggest they were sensitive to the change between the target and the distractor presented during the task. Previous studies have used d’ scores because they control for possible response bias participants might have when responding to same/different change detection tasks (Brady & Hampton, 2018; Elmore et al., 2011).
A 2-way RM-ANOVA with a 2 (Property: Identity vs Spatial), by 2 (cueing: pre-alone vs pre-retro) factors was conducted on d’. Analysis of the training phase showed a significant main effect of property, F(1,21) = 63.74, p ≤ .001,n2 p = .752, with higher sensitivity on identity (.83 ± .16) compared to spatial trials (.63 ± .15; p ≤ .001; Figure 2a). Also, a significant main effect of cueing was found, F(1,21) = 6.40,p = .020, n2 p = .233, showing a higher sensitivity on prealone(.75 ± .20) compared to pre-retro (.70 ± .16), particularly in spatial (p = .018) trials.
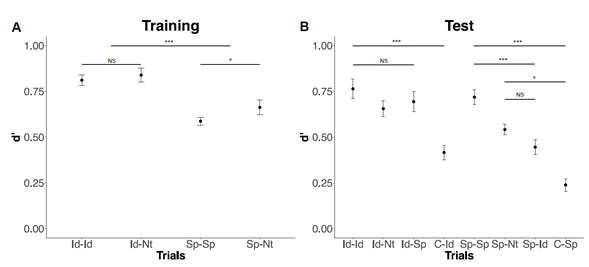
Note. A) On the x-axis, each pair of letters stands for the property signalled by the first and second cue on a trial (Id for identity, Sp for spatial, and Nt for neutral). B) All test trials are shown. The highest interference was expected on Id-Id and Sp-Sp trials, with moderate (Id-Nt and Sp-Nt) and low (Id-Sp and Sp-Id) on the other trials. Error bars represent standard error.
Figure 2 d’ scores for training and test phases. P-values ≤ .001(***), ≤ .01(**), ≤ .05(*), and non-significant (NS) were reported
A 2-way RM-ANOVA with a 2 (Property: Identity vs Spatial), by 4 (interference: High, moderate, low, control) factors on Test trials showed a significant main effect of property, F(1,21) = 36.72, p ≤ .001, n2 p = .636, showing overall higher sensitivity on identity (.63 ± .26) compared to spatial trials (.49 ± .24; p ≤ .001). There was also a significant main effect of interference, F(3,63) = 47.80, p ≤ .001,n2 p = .695, showing a lower sensitivity in control (.33 ±.19) compared to low (.57 ± .26; p ≤ .001), moderate (.60± .18; p ≤ .001), and high (.74 ± .22; p ≤ .001) interference. Participants also were significantly more sensitive during high interference trials compared to moderate and low interference trials (ps ≤ .001). A significant 2-wayinteraction was also found, F(3,63) = 3.28, p = .026, n2 p =.135, with less sensitivity on control compared to low, moderate, and high interference trials when the important dimension was identity (ps ≤ .001) or spatial (ps ≤.012). However, there was reliably higher sensitivity in high interference compared to moderate and low interference(ps ≤ .001) on spatial trials (Figure 2b).
Discussion
Including a distractor between the presentation of a target and probe stimulus in a change detection task can interfere with WM for the target, especially if the new stimulus shares similar features with the target or probe. Priming that distractor should facilitate its recognition and interfere with memory for the target. However, a priming cue before a distractor should cause the most interference when the relevant property of the distractor primed was the same property as the target stimulus that must be remembered. It was hypothesized that participants would exhibit lower performance in the high interference trials, followed by medium and low interference trials. In contrast, if the cue before the distractor acts as a retro-cue for the target stimulus, and protects it from interference by the distractor, then the reverse pattern of results would be predicted. These results of the current experiment support the retro-cue account of the effect of a cue presented after the target stimulus but before the distractor.
Based on the extant priming literature, performance was expected to be the lowest in the high interference trials when the CB priming cue signalled participants to process the property of the distractor stimulus(snowflake) that was congruent with the property of the target snowflake that must be maintained in WM to evaluate the probe stimulus. However, we observed that these conditions resulted in the best performance.
Rather than interfering with WM for the target, it appears as if the second CB protected the target from interference. This effect is consistent with the evidence for retro-cueing benefits (Fu et al., 2022; Griffin & Nobre,2003). Instead of priming WM for the features of the distractor and disrupting WM for the target, the second CB cue worked as a retro-cue for the target, enhancing its processing and causing higher performance in these trials relative to trials in which the second CB signalled the other property, or was a neutral stimulus that did not signal a particular property to remember. These results support the protection from interference hypothesis, which suggests that the presentation of a retro-cue causes attentional resources to be focused on the representation of the recently presented target, protecting it from interference by objects that follow (Matsukura et al., 2007; Souza et al., 2016).
Participant’s RTs did not show any significant main effects during training. Nonetheless, the trend found in the main effect of property (identity vs. spatial) suggests there may be a difference between the trial demands of each. More interesting is the trend in the interaction between property and interference (high vs. Medium vs. low vs. control) found during the test trials, which could indicate that high interference in spatial trials resulted in faster responses than when medium interference was present, but the same result was not found in identity trials. This trend provides weak support for a retro-cueing effect over a distractor priming effect on RTs, however, it’s unclear why the result was only found with spatial trials.
Analysis of d’ in the training phase was consistent with the previous finding with RTs, such that participants were more sensitive to changes in identity than spatial location. Interestingly, there was also a main effect of cueing, such that the presence of the pre and retro-cues resulted in lower sensitivity to the signalled property compared to only the pre-cue trials during training, which is the opposite result of what occurred during testing. These effects were stronger for spatial trials. In the test phase, post-hoc tests of the main effect of interference revealed that performance was better in high interference trials in which the pre and retro-cues were presented than in trials with only a pre-cue or only a retro-cue that signalled a different property. One interpretation is that the retro-cue did not provide a processing advantage during initial training, but it did when interference via the distractor was added at test. The retro-cue was then able to protect the WM representations of the targets.
There was also an interaction between property and interference in d’, which revealed that the effect of the retro-cue was stronger for trials of spatial features. In fact, there is some evidence for negative priming in spatial trials in which the pre-cue signalled that the relevant property was the target’s spatial position, but the retro-cue signalled a test of the identity of the snowflake. This effect may be due, not to the presence of the distractor, but to negative priming by the retro-cue of the target. Further research could evaluate why negative priming was found with spatial, but not identity trials.
One limitation to these findings is that only the low interference trials (Id-Sp and Sp-Id) were novel at test. Given that the low interference trials resulted in the most disruption of performance at test during spatial trials, it is possible that the novelty of the cue sequence(Id before Sp) is responsible for the deficit. However, there is evidence that argues against this explanation. The first is that pre-retro trials did not differ from pre-alone trials during training, but they did during test. Neither of these trial types were novel, and yet a difference was also found at test in the direction predicted by a retro-cue account. Second, participants had experience with the Id and Sp cue in both the pre and retro positions and had experience responding correctly when the colour of the second cue differed from the first in pre-alone trials with each cue. Lastly, performance in pre-alone (Id-Nt) and low interference trials (Id-Sp)did not differ during testing. If novelty were the only source of any difference between trial types, then this finding would not be expected. In contrast to the effect of novelty, these findings are interpreted in terms of the effectiveness of a retro-cue. A retro-cue that signals a test of a property of a target object (e.g., Id) that matches the same property (e.g., Id) signalled by a pre-cue better protects WM from interference by a distractor than retro-cue that signals a test of a different property (e.g.,Sp) of the target object.
Overall, these results support a role for retro-cueing that is greater than pre-cueing the distractor. Though not all measures supported this conclusion, in no measure did we find evidence for greater interference in the high interference condition, whereas, evidence across measures was found for retro-cueing. Future studies should aim to modify the stimulus type, as well as other procedural variables (e.g., interstimulus timing) known to influence retro-cueing vs. selective interference. It is possible that one effect may be more robust to variation sin procedure. Alternatively, different experimental parameters could lead to one or the other effect, which would improve our understanding of the role of these parameters in producing everyday effects on WM performance 1 2.