Introduction
Milk yield in dual-purpose cattle production systems is limited by the low availability and poor quality of pastures, so the use of energy and protein supplements is required to increase production (Garduza-Arias et al., 2013). Protein is the most deficient and expensive nutrient, hence the importance of developing economically feasible technologies to produce foods using local resources. Devilfish (Pterygoplichthys sp.) is a pest with high environmental and social impact in aquaculture production systems (Pérez and Iglesias, 2016). It can be included as a protein source in supplements for lactating cows through anaerobic fermentation using lactic bacteria and carbohydrates (biological silage). However, the resulting fishy taste and smell in milk could limit its use.
Milk is one of the most important foods for humans. Milk quality involves several components (total solids, proteins, fats, vitamins, and minerals), and absence of disease-causing bacteria, chemical residues and inhibitors, especially antibiotics and unpleasant odors and flavors (Park et al., 2013). It is well known that the type of food ingested by dairy cows can affect milk composition. Supplementation with fish-based ingredients may cause changes in milk fatty acids composition and aromatic compounds that could affect milk sensory and physicochemical characteristics. Therefore, the objective of this study was to evaluate the effect of biological silage of fish as a source of protein on the microbiological, sanitary, physicochemical and sensory characteristics of milk.
Materials and methods
Geographic location of the study area
The study was conducted in a cattle operation in the community of Vicente Guerrero, in Jalpa de Méndez, Tabasco, Mexico (18° 25' N and 18° 04' W, at 10 m altitude). The climate is warm and humid, with abundant rains during summer, when average high and low temperatures fluctuate between 30.5 and 22.5 ºC, respectively. Annual rainfall is between 1,500 and 2,000 mm.
Animals, treatments and experimental design
Twelve cross-bred cows (Zebu x Holstein and Zebu x Swiss) were selected, considering the body weight and days in lactation. Liveweight was 457.08 ± 43.61 kg, and the number of days of lactation was 45.08 ± 13.88 d. The cows were randomized in a Latin square design, in such a way that three treatments were tested on all the cows during three periods of time. The columns comprised the cows and the rows were periods.
Each period lasted 20 d (15 d for the adaptation phase, and 5 d for the experimental phase). The treatments (T) evaluated were T1, 0% fish silage (0FS); T2, 10% fish silage (10FS); and T3, 20% fish silage (20FS). The protein source in the control treatment was soybean meal, which was substituted by biological fish silage in the treatments.
Biological fish silage
Fresh fish caught (Pterygoplichthys sp.) were washed, decapitated, and ground in a Rayken RKP2000B C/Motor Husky 6.5 HP grinder (Rayken, Puebla, Mexico). Thereafter, a mixture of 60% fish, 20% molasses, and 20% microbial inoculum was prepared. The fermentation lasted 15 d in a hermetically sealed 200 L plastic tank (Figure 1). The mixture was stirred in the mornings of the first 5 days.
The microbial inoculum was previously obtained by fermentation in a liquid state medium consisting of 4% soybean meal, 4% rice bran, 15% molasses, 0.5% mineral salts, 0.5% urea, 0.3% magnesium sulfate, 5% natural yogurt (Yoplait®), and 70.7% water in a 200 L tank. The mixture was stirred four times per day. After three days, microbial inoculum traits were: pH 4.1, 81.8% moisture, 24.9% crude protein, and log10 8.97 colony forming units (CFU) of lactic acid bacteria per mL, and was ready to be used in the preparation of fish silage.
Preparation of supplements (treatments)
The supplements were prepared with the ingredients shown in Table 1. Once the ingredients were mixed, they were packed in 30 kg plastic bags to be preserved anaerobically. Supplements were formulated to be isoproteic (18% CP), and isoenergetic (2.8 Mcal kg-1 DM) using the “Solver” software from Microsoft Excel.
Chemical analysis of the supplements (treatments)
Dry matter (DM), crude protein (CP), neutral detergent fiber (NDF), acid detergent fiber (ADF), and ash were determined following Latimer´s (2016) methods. Degradation of dry matter (DDM) was determined following the methodology described by Naranjo and Cuartas (2011).
Animal handling
The cows were milked twice a day (5:00 h and 16:00 h) using a milking machine. At each milking, each cow was offered the respective supplement (3 kg wet basis) treatment.
After milking, the cows were kept in an enclosure where they had free access to water and sorghum silage, which contained 27.67 ± 1.5% DM, 8.9 ± 0.91% CP, 61.1 ± 3.6% NDF, 35.1 ± 3.09% ADF, and 8 ± 1.0% ash.
Milk sampling
In the experimental phase (day 15-20), three milk samples of different size were taken, according to analysis to determine milk quality. The first, for microbiological and sanitary analyses on the first day, a second for sensory tests on the third day, and a third for physicochemical analyses on the fifth day. All samples were kept refrigerated at 4 °C before analysis. For the microbiological analysis, 100 ml milk was collected by manual milking into Whirl-Pak® bags (Sigma, St Louis, MO, USA). For the sensory tests, the milk samples (from four cows per treatment) were taken by mechanized milking and bottled in 5-L glass jars. For the physicochemical analysis, 100 ml milk samples were taken into Whirl-Pak® bags by individual mechanical milking.
Microbiological, sanitary and physicochemical analysis of milk.
The presence of Salmonella spp was assessed with the Ridascreen® Salmonella kit. (R-Biopharm AG®, Darmstadt, Germany). Aerobic mesophilic bacteria, total coliforms, and Escherichia coli were counted using the kits RIDA® Count total, RIDA® Count coliform, and RIDA® Count E. coli (R-Biopharm AG®, Darmstadt, Germany), respectively. Somatic cells (SC) were counted with DeLaval® cell counter DCC (DeLaval®, International AB, Tumba, Sweden), and the presence of antibiotic residues in milk was determined with the Delvotest® SP-NT kit (DSM® Food Specialties, Delft, Netherlands). Physicochemical composition of milk was determined with the Lactoscan® Milk Analyzer (Milkotronic® LTD, Nova Zagora, Bulgaria).
Sensory evaluation
Sensory evaluation of milk was conducted in the Sensory Evaluation Laboratory (DACA- UJAT) by eight trained panelists. The panelists were trained with samples of freshly milked and pasteurized milk and whole commercial milk. The results of each session subjected to analysis of variance (ANOVA) to detect differences between judges. Once the analysis did not indicate differences between judges, the treatments were evaluated. Prior to sensory evaluation, milk was pasteurized (65 ºC for 30 minutes), and stored at 5 ºC before use.
During each evaluation period, triangle-type discriminatory tests were conducted to evaluate differences among the three treatments (0FS vs 10FS, 0FS vs 20FS and 10FS vs 20 FS). In addition, a quantitative descriptive analysis (QDA) was performed to evaluate the intensity of sensory attributes of the samples of each treatment. The descriptors evaluated were those reported by Citalán et al. (2016), and “fish smell” and “fish taste” as additional descriptors. Descriptors and definitions are shown in Table 2. Those descriptors had been analyzed and discussed in a working session with the judges to clarify doubts and define the terms by consensus, as shown in Table 2.
Preparation and evaluation of samples for the descriptive test
Samples (40 mL) were served in glasses coded with three-digit random numbers. The panelists were instructed to evaluate the samples in the order of the attributes on a 10 cm non-structured linear scale ranging from null to optimal or null to intense. Intensity was indicated by placing a mark on the unstructured 10 cm linear scale, anchored with the terms “poor” and “optimal” or “null” and “intense” at each end. The answers were quantified by measuring the distance in centimeters from the extreme left to the mark indicated by the panelist. All samples were kept at 12 ºC for evaluation. Between samples, the judges drank natural water to eliminate effects from the previous sample.
Statistical analyses
An analysis of variance and Tukey tests of multiple comparisons of means were performed for each of the microbiological composition, sanitary, physicochemical and sensory (QDA) milk variables using SAS® 9.4 (2017). To normalize the data, values of aerobic mesophilic bacteria and somatic cells were transformed to Log10, and the values of total coliforms were transformed to Log10 [Y+1]. The data from the discriminatory tests were analyzed by counting the number of correct answers and comparing them with the minimum number required to establish a significant difference between the samples of the triangular test at a significance level of 0.05 (Roessler et al., 1978).
Results
Chemical analysis of the supplements
Food supplements used in this study (Table 1) were calculated to be isoprotein (18 % PC), and isoenergy (2.8 Mcal kg-1 DM). However, when evaluating the chemical composition, the PC content of the 0FS treatment was higher (p<0.05) compared to treatments 10FS and 20FS (Table 3), the moisture content had a linear increase (p<0.05) in relation to the percentage of fish silage inclusion in the supplement, and treatment 20FS had the highest (p<0.05) percentage of ash relative to 10FS and 20FS; in all treatments, pH was less than 4, with no differences between treatments, due to the addition of 5% of the microbial inoculum in all treatments.
Feed intake
There were significant differences in supplement intake (P<0.05) on dry basis. Intake was highest for cows with 0% fish silage and lowest for cows that consumed the 20% treatment (Table 4). According to the total consumption of supplements on a dry basis, the consumption of devil fish silage was 0.469 and 0.908 kg animal-1 d-1 for the 10FS and 20FS treatments, respectively.
Table 3 Chemical composition, and pH of supplements.

Means within the same column with different superscript letters (a, b) are statistically different (P<0.05).
Milk analysis
Aerobic mesophilic bacteria were less than 100,000 CFU mL-1 for all the treatments. The number of coliforms was also low (Table 5). Neither Salmonella nor E. coli were detected in any sample. Low numbers of somatic cells were found, without significant difference for the three treatments (P<0.05). There were no residues of antibiotics or antimicrobials in milk from the animals studied, according to the Delvotest (Table 5). No significant differences (P>0.05) were found among treatments for contents of fat, protein, lactose, or non-fat solids (Table 5). Milk fat content was ˃31 g L-1 in the three treatments.
Sensory analysis of milk
The triangle sensory test did not reveal significant differences (P>0.05) between milk samples from 0FS and 10FS. However, there was a significant difference (P≤0.05) comparing 0FS vs 20FS; and between the 10FS vs 20FS (Table 6).
Table 5 Indicators of microbiological, sanitary and physicochemical quality of milk from cows supplemented with fish silage.
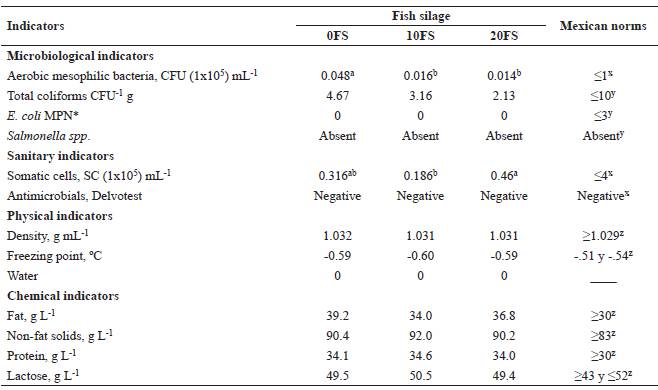
Means within the same column with different superscript letters (a, b) are statistically different (P< 0.05).
*MPN= Most probable number; xNMX-F-700-COFOCALEC-2012; yNOM-243-SSA1-2010; zNOM-155-SCFI-2012.
Table 6 Number of correct answers with the triangle test during evaluation of milk from cows supplemented with biological fish silage.

*(P<0.05) according to Roessler et al. (1978).
In the quantitative descriptive analysis, 25 out of 26 of the descriptors did not differ statistically among treatments (Figure 2). The descriptor “creamy milk taste” of the QDA was significantly more intense in the samples from 20FS, relative to the intensity observed in 10FS and 0FS. The grade for typical milk taste was high (≥8) for the 3 treatments. Other descriptors such as “strange odor” or “fish odor” had very low grades (≤1) for all the treatments.
Discussion
Perez (1995) reported that fish silage intake increased milk yield of Holstein cows in Cuba; however, that researcher did not include information on milk quality assessment. Milk of all the treatments in the present study was classified as “grade A” because it had ˂100.000 CFU mL-1 of mesophilic microbes, it had a low number of somatic cells, and no antimicrobials were detected in the Delvotest (Table 5). This quality is generally associated with good dairying farming practices during milking and sampling. Fat in the 3 treatments was higher than 32 g L-1. Avramis et al. (2003) reported that Holstein cows fed on maize and fish meal as feed supplements produced milk that contained 24.3 g L-1 fat, which is lower than that found in our study. Cows fed on diets supplemented with fish meal usually reduce milk fat content and total production of dairy fat (Wright et al., 2003). Spain et al. (1995) indicated that the percentage and yield of milk fat decrease with a higher intake of fish meal. Keady et al. (2000) reported that the higher the quantity of fish oil in the diet, the lower the percentage of fat and protein in the milk. In our study, the percentage of fat and protein in milk were not affected by the inclusion of fish silage. It is important that the supplements used to feed cows are associated with high-fat content in milk because fat it is one of the most economically valued components of dairy products. The price of milk increases with higher fat and protein contents (Wolter et al., 2004).
Protein content in the milk from the three treatments was within the levels specified in CLASS A, because it contained ˃31 g L-1 (NOM-155-SCFI-2012, NMX-F-700- COFOCALEC-2012). Avramis et al. (2003) reported that Holstein cows fed a fish meal- based supplement produced milk with 33.2 g L-1 protein. When fish oil is included in the diet, protein content tends to be lower than that produced with other diets (Fatahnia et al., 2008).
Avramis et al. (2003) stated that one of the main disadvantages of fish-based feed for ruminants is the fishy taste it produces in milk or meat, which is associated with supplements that contain fatty acids from ingredients such as fish oil or meal. However, we demonstrated that supplementation with biological fish silage as the protein source did not generate fish odor or fish taste in milk. Moreover, there were no differences between treatments associated with the other sensory attributes evaluated in the ADC (Figure 2), except for the “creamy milk taste”. Shingfield et al. (2003) reported that fish oil in dairy cow diets contributes to changes in the composition of fatty acids in the milk. These changes in fatty acid composition could be related to a higher intensity of the “creamy milk taste” descriptor perceived by the judges. Avramis et al. (2003) did not find differences in the analyses of quality and taste between the milk from Holstein cows supplemented or un-supplemented with 60% herring (Clupea spp.) meal. The Holstein cows had been supplemented with fish meal as 4.5% of their total dry-matter intake.
Descriptors related to negative effects that supplementation of fish ingredients could have on sensory characteristics of milk, such as fish taste or odor, were included in the QDA. Chilliard et al. (2001) stated that changes in dairy cow diets could change milk taste, color, vitamin content, oxidative stability, proteins, and processing costs. Bragaglio et al. (2015) reported that milk from cows fed fish oil (protected or unprotected) was more susceptible to oxidation, which affects sensory properties. Indeed, oxidation of polyunsaturated fatty acids produces a complex mixture of volatile products of secondary oxidation, some of which produce unpleasant flavors. However, in our study, no increase or significant change in unpleasant or strange odors or flavors was observed; panelists did not perceive fish odor or taste in any of the evaluated samples (Table 6). Otherwise, no difference was observed relative to the consistency or general aspect of the product (Figure 2).
In conclusion, fish silage can be used up to 20% as a source of protein in supplements to produce high-quality milk. The fish silage in the supplement did not affect the microbiological, sanitary, physicochemical or sensory characteristics of the milk.