INTRODUCTION
Plant rhizosphere harbors several microbial groups whose physiological activity significantly influences soil fertility, quality, and health properties (De Ridder-Duine et al., 2005; Sanon et al., 2009; Nie et al., 2011), and stimulates the proliferation and abundance of microorganisms able to detoxify or degrade soil contaminants (Sanon et al., 2009; Sun et al., 2015). This rhizosphere interaction not only benefits the microbial communities but also influences positively both plant growth and adaptation (Walker et al., 2003; Harrier and Watson, 2004; Hayat et al., 2010).
Soil microflora is mainly represented by bacteria and fungi (Dajoz et al., 2002; Weidmann et al., 2004). Bacteria may release organic compounds, some of them may establish symbiosis with plants, and others may inhibit the proliferation of plant pathogens due to secretion of antibiotic compounds (Barea, 1998; Ferrera-Cerrato and Alarcón, 2007; Mitter et al., 2013). Microorganisms play a significant role in nutrient cycling in soil such as biological atmospheric nitrogen fixation or solubilization of inorganic phosphates, whose deficiency typically impairs plant growth and development (Barea, 1998). Also, arbuscular mycorrhizal fungi (AMF) are obligated biotrophic symbionts that colonize cortical cells of roots of most of the extant terrestrial plants, and enhance plant nutrition and growth, as well as plant adaptation against stressful soil conditions, water deficiency, contamination, or pathogens (Linderman, 2000; Jeffries et al., 2003; Liu et al., 2004; Hernández-Ortega et al., 2012). These fungi have important effects during the phytoremediation of soils contaminated with petroleum hydrocarbons (Cabello, 2001) by enhancing plant adaptation, growth, nutrition or by stimulating the proliferation of petroleum-degrading microorganisms in the rhizosphere (Joner and Leyval, 2003; Alarcón et al., 2008; Hernández-Ortega et al., 2012). The later benefits highlight the crucial role of rhizosphere microorganisms by improving physical and chemical properties in the surrounding edaphic environment (Zhang et al., 2006; Bento et al., 2012).
Soil pollution by accidental oil spills is an environmental issue that has received special attention worldwide. These contaminants modify soil properties by forming a layer covering the surface and the pore space, thus affecting oxygen diffusion (Franco et al., 2004; Nageswara-Rao et al., 2007; Sun et al., 2015). Likewise, hydrocarbons decrease water retention due to their hydrophobic properties, and significantly increase the amount of carbon, induce acidification processes, and decrease the cation exchange capacity (Li et al., 2000; Châineau et al., 2003; Rivera-Cruz et al., 2005; Nie et al., 2011).
When hydrocarbons accumulate in the rhizosphere the most affected physiological process in the plant is photosynthesis so that the chlorophyll content decreases in leaves (Adenipekun et al., 2008; Baruah et al., 2014) and the synthesis of proteins, sugars, and lipids are affected, thus, plant development is limited (Nageswara-Rao et al., 2007). These contaminants exert pressures on the floristic composition, favoring the selection of well-adapted plant species. Part of this adaptation consists in their association with soil microorganisms as a mechanism to withstand the adverse conditions caused by contaminants (Franco et al., 2004; Nageswara-Rao et al., 2007). Besides causing toxic effects to many microorganisms, some oil fractions are utilized as a source or carbon and energy for satisfying microbial growth (Franco et al., 2004; Gerdes et al., 2005, Labud et al., 2007; Essien et al., 2013; Dellagnezze et al., 2014). These are evident on culture-dependent microorganisms, by which is possible the characterization and the selection of microorganisms with potential use for bioremediation of soils contaminated with several compounds (Alkorta et al., 2004; Hubalek et al., 2007; Zhuang et al., 2007; Singh, 2008; Chibuike, 2013; Zhou et al., 2013; Dellagnezze et al., 2014; Ullah et al., 2015). Thus, certain physiological/ functional microbial groups have significance relevance under contaminated environments since they may contribute on nutrient cycling such as nitrogen (N), phosphorus (P) or more importantly on promoting plant growth (Ramirez-Elías et al., 2014; Morales-Guzmán et al., 2017; Alejandro-Córdova et al., 2017).
The responses of AMF to petroleum hydrocarbons are related to reducing root colonization. However, AMF may stimulate plant survival growing at contaminated conditions, and allow the proliferation of microorganisms able to degrade organic compounds (Binet et al., 2001; Liu et al., 2004; Franco-Ramírez et al., 2007; Labud et al., 2007; Alarcón et al., 2008; García et al., 2013; Kuo et al., 2014; Alejandro-Córdova et al., 2017).
The ability of plants to grow at contaminated media varies from one species to another, and this variation is the key for the remediation of soils contaminated with petroleum hydrocarbons (Akutam et al., 2014). Clitoria ternatea (L.), and Brachiaria brizantha (A. Rich) are plant species of tropical regions, easy to establish, resistant to drought, and tolerant to organic contaminants (Sangabriel et al., 2006; FAO, 2015). However, the rhizosphere microbial populations in both plant species under contaminated soils are not well studied.
Thus, the present study evaluated the effects of crude oil contaminated soil on the culture-dependent population of rhizosphere microorganisms, whose physiological activity is related to the incorporation of atmospheric nitrogen, the solubilization of inorganic phosphates in the soil, and the promotion of growth of two plant species established in mesocosms under greenhouse conditions.
MATERIALS AND METHODS
Soil collection and mesocosms establishment
The soil was collected from the municipality of Rodriguez Clara, Veracruz (Mexico) at coordinates 18°00' N and 95°24' W, 95 m.a.s.l., without the previous problem of petroleum hydrocarbon pollution. The soil sample was collected (20 cm depth), and analyzed to determine cation exchange capacity (CIC), organic matter content (OMC), and content of P, N, and C. The main soil physical and chemical characteristics were: sandy-loam texture (71 % sand, 17 % silt); 5.7 meq CEC, 1.5 % OMC, 0.07 % total N, 6 mg P kg-1 (Olsen), and 0.02 meq K L-1.
Eight kilograms of dry sieved soil (2 mm mesh) were placed in each of the 15 plastic containers (36 x 30 x 14 cm) used as mesocosms. The soil was artificially contaminated with crude oil at the following concentrations: 3000, 6000, 9000, and 12000 mg kg-1, respectively. A treatment without oil pollution was included as a control. The crude oil was dissolved with 300 mL of dichloromethane (Baker®) to reduce oil viscosity and facilitate the soil impregnation. This solvent is quickly volatilized (< 0.002 % of residue after evaporation) and do not exert significant effects on soil microorganisms (Alarcón et al., 2008); thus, there was no need to establish control with the application of this solvent.
One week after contamination, 15 seeds of Clitoria ternatea L. (Fabaceae) and 15 of Brachiaria brizantha (Hochst. ex Rich.) (Gramineae) were planted in combination in each mesocosm evenly distributed in the substrate at one-centimeter depth.
Throughout the experiment (240 days) under greenhouse conditions, the mesocosms were irrigated with tap water as needed. The temperature and relative humidity (maximum and minimum) prevailing during this research were 35.4+5.4 and 13.7+1.6 °C, and 82.9+7 and 28.8+ 11.1 %, respectively (Data logger WatchDog, model 450).
The population of functional groups of culturable bacteria and root colonization of symbiotic microorganisms
24 hours after soil contamination samples were taken to estimate the culturable bacterial populations as described in the following paragraph and to compare them with the two further sampling times (60 and 240 days) described as follow.
Soil sampling was collected at 60 and 240 days. For each mesocosm, a composite soil sample was prepared from five sampled points (300 g rhizosphere soil each). Thus, three composite samples per treatment were obtained. From these composite samples, 10 g of soil were used for determining culturable microorganisms according to serial dilutions and agar plate counting technique (Lorch et al., 1995) using the following growth media: combined carbon (Rennie, 1981) to assess the colony forming units (CFU) of nitrogen-fixing free-living bacteria (NFFLB), and Pikovskaya (Subba-Rao, 1993) to assess the total P-solubilizing bacteria (PSB). For C. ternatea, the number of root nodules formed by native rhizobia was evaluated.
The mycorrhizal colonization in both C. ternatea and B. brizantha (three plants of each species per treatment were harvested at each sampling time) was quantified through the root clearing and dyeing method (Phillips and Hayman, 1970). Once the roots were dyed and mounted on slides, the frequency of AMF structures (hyphae, vesicles, and arbuscules) in each root fragment was estimated using a clear field optical microscope (Reichert, Microstar Model 410) at 40 X magnification, and to calculate the percentage of colonization. The extraction ofAMF spores was performed by the wet sieving and decanting method (Gerdemann and Nicolson, 1963), followed by centrifugation in 70 % sucrose (Castillo et al., 2008). The undamaged spore counting was done under a stereomicroscope (Reichert, StereoStar Zoom), and results were expressed as a number of spores per 100 g dry soil.
Assessment of phototoxicity of oil in Clitoria ternatea and Brachiaria brizantha
At the end of the experiment (240 days), the toxicity of the crude oil to plants was evaluated by quantifying the dry biomass and the total chlorophyll content in leaves of both species. The total dry weight of C. ternatea and B. brizantha was determined by harvesting three individuals per species of each mesocosm and then drying the harvested plant material at 70 °C for three days. The total chlorophyll content was determined by the method described by Dere et al., (1998). One leaf of C. ternatea, and two square centimeters of leaf tissue from B. brizantha were used for pigment extraction, from which the fresh weight was obtained. Leaf samples from each plant were placed in test tubes with 5 mL 80 % acetone and kept at 4 °C for one week. Subsequently, from the obtained solution, absorbance readings were taken, 470, 645, and 662 nm, in a spectrophotometer (Hewlett Packard, model 8453).
Experimental design and statistical analysis
The experiment consisted of five treatments with three replicates each, distributed in a completely randomized experimental design. Data obtained from each sampling (60 and 240 days) were analyzed using analysis of variance and the mean comparison test (LSD, α=0.05) using the SAS version 8 for Windows (SAS Institute, 2002). The percentages of mycorrhizal colonization were transformed to arcsine units, while the values from the quantification of bacterial CFU were transformed to log units for subsequent statistical analysis.
RESULTS
The population of functional groups of culturable bacteria and root colonization of symbiotic microorganisms
In response to crude oil concentrations, the NFFLB and PSB populations decreased significantly (p≤0.001) at the beginning of the experiment (time zero) in comparison to the control (Fig. 1a-b). After 60 days, a significant increase of NFFLB and PSB populations was detected due to the crude oil, in comparison to the control (Fig. 1a-b). At 240 days, the NFFLB population was significantly higher in treatments with 9000 and 12000 mg kg-1 than the control (Fig. 1a), while the PSB population was similar among treatments (Fig. 1b).
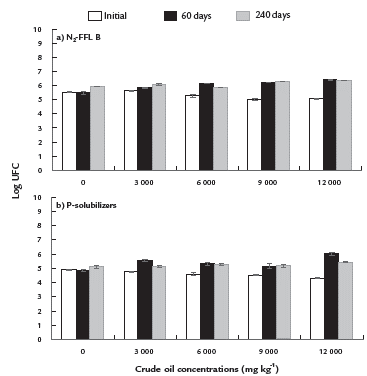
Figure 1 Populations of (a) N-fixing free-living bacteria (N2-FFLB) and (b) P-solubilizing bacteria in the rhizosphere of Clitoria ternatea and Brachiaria brizantha grown in mesocosms contaminated with four concentrations of crude oil, at an initial time (24 h after contamination), 60 and 240 days. Means ± standard error. Mean comparison test (LSD, α=0.05). n=5.
The number of nodules in C. ternatea significantly decreased (p≤0.001) at contaminated soils (Fig. 2a). At 60 days, control plants had in average 25 nodules, whereas at contaminated treatments, plants had in average four nodules (Fig. 2a). At 240 days, control plants showed 27 nodules, while concentrations of 3000 and 6000 mg kg-1 resulted in a low number of nodules (7) (Fig. 2a). In contrast, treatments with 9000 and 12000 mg kg-1, resulted in the lowest number of nodules (Fig. 2a).
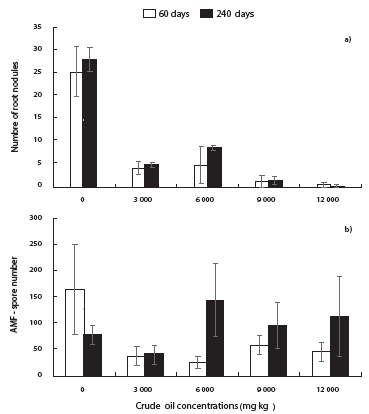
Figure 2 Number of nodules formed by native rhizobia in roots of Clitoria ternatea (a), and number of spores (b) of arbuscular mycorrhizal fungi (AMF) in 100 g of dry soil collected from the rhizosphere of Clitoria ternatea and Brachiaria brizantha grown mesocosms contaminated with four concentrations of crude oil, after 60 and 240 days. Means ± standard error. Mean comparison test (LSD, α=0.05). n = 5.
Mycorrhizal colonization at 60 days in B. brizantha and C. ternatea grew at control treatment was 52.6 and 64 %, respectively, showing significant differences to treatments with crude oil (15 and 18 % colonization in average, for both plat species, respectively) (Fig. 3a-c). At 240 days, B. brizantha at control treatment showed high colonization (63.8 %), which significantly decreased as crude oil concentration increased, especially at 12000 mg kg-1 (Fig. 3b). At 240 days, C. ternatea grown at control treatment showed the highest colonization (53 %), but plants at 12000 mg kg-1 had mycorrhizal colonization as low as 10 % (Fig. 3d).
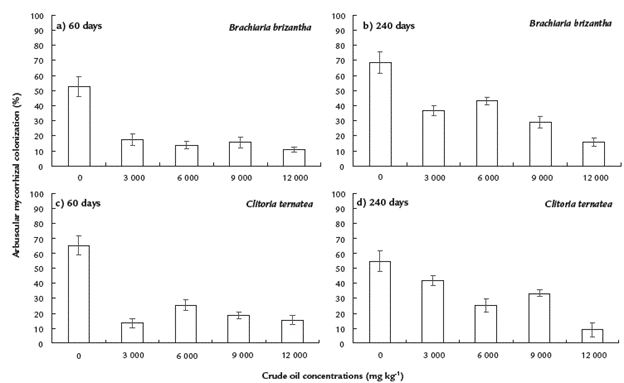
Figure 3 Arbuscular mycorrhizal colonization in roots of Clitoria ternatea and Brachiaria brizantha grown in mesocosms contaminated with four concentrations of crude oil, after 60 (a and c) and 240 (b and d) days. Means ± standard error. Mean comparison test (LSD, α=0.05). n=5.
After 60 days, control treatment had the highest number of AMF spores (165 spores in 100g dry soil), but in contaminated treatments, the number of spores ranged from 55 to 28, without presenting statistical differences among treatments (Fig. 2b). In contrast, the number of spores at 240 days was significantly higher at the concentration of 6000 mg kg-1 (140 spores) than that from 3000 mg kg-1 (45 spores) (Fig. 2b).
Phytotoxic effects of crude oil on Clitoria ternatea and Brachiaria brizantha
The total dry weight of B. brizantha at 60 days was significantly higher in the treatment with 3000 mg kg-1 when compared to the control and the remaining contaminated treatments (Fig. 4a). At 240 days, the highest dry weight was obtained in control plants and the lowest in plants grown under 12000 mg kg-1 (Fig. 4b). For C. ternatea no significant differences were observed among treatments at 60 days (Fig. 4c), but at 240 days, the total plant dry weight decreased significantly (p≤0.001) as the crude oil concentrations increased (Fig. 4d).
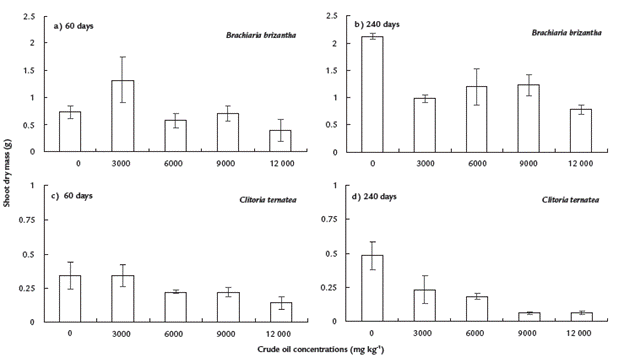
Figure 4 Shoot dry mass of Brachiaria brizantha and Clitoria ternatea grew in mesocosms contaminated with four concentrations of crude oil, after 60 (a and c) and 240 (b and d) days. Means ± standard error. Mean comparison test (LSD, α=0.05). n=5.
For B. brizantha, total chlorophyll content at 60 days was significantly higher in the treatment with 12000 mg kg-1 when compared to the remaining treatments (a). However, after 240 days, the total chlorophyll content significantly decreased in all treatments, although the significant highest content was obtained in plants exposed to 9000 mg kg-1 (Fig. 5b). For C. ternatea, at 60 days, chlorophyll content decreased significantly as the crude oil concentration increased (Fig. 5c). In contrast, at 240 days, plants grown in treatments with 6000 and 9000 mg kg-1 had significantly higher chlorophyll content than the remaining treatments (Fig. 5d).
DISCUSSION
Increasing concentrations of crude oil caused significant reduction of bacterial populations at initial sampling time, thus, proving the negative effects of this contaminant, which also acts as a selective toxic agent. Chikere et al., (2009) indicated that the reduction of bacterial populations is an adaptive response to petroleum hydrocarbons because of their hydrophobic properties that reduce the enzyme activity and the ability of plants and microorganisms to absorb water and nutrients (Van Hamme et al., 2003; Osuji and Nwoye, 2007; Nie et al., 2011). Also, microorganisms compete for available nutrients and energy sources; thereby the microbial population is restricted (Miranda-Martínez et al., 2007). Nevertheless, after 60 days, the recovery of NFFLB and PSB was observed since their populations were higher than the control. The growth of bacterial populations may be due to the selective effects of crude oil on soil microorganisms, favoring those with the ability to degrade or utilize petroleum hydrocarbons as a source of carbon and energy (Delille et al., 2003; García et al., 2013; Trujillo-Narcia et al., 2014) which prevailed over time. For example, Proteobacteria and Actinobacteria are dominant bacterial groups in contaminated soils and able to metabolize hydrocarbons (Yang et al., 2014). Delille et al., (2003) and Kaplan and Kitts, (2004) mentioned that when an event of oil pollution occurs there is a first microbial process of fast degradation of labile or less toxic fractions of hydrocarbons; as these fractions are consumed, a second degradation phase starts in which the remaining toxic compounds are attacked.
Furthermore, these processes are regulated by both physicochemical properties of hydrocarbons and environmental conditions (Stroud et al., 2007). Although no analyses of hydrocarbon degradation were performed, the described microbial processes explain in part the significant recovery of bacterial populations after 60 days.
Undoubtedly, tap water irrigation may contribute with the addition of some microorganisms to mesocosms, but petroleum hydrocarbons acted as a selective agent from the beginning of experimentation, so at the end time (240 days), this contaminant acted on the recovery and stabilization of well-adapted NFFLB and PSB populations, and on the increased colonization of AMF and nodule-forming rhizobacteria. This phenomenon was described by Liste and Felgentreu, (2006) with legumes and grasses in fields exposed to petroleum hydrocarbons.
Crude oil significantly affected the formation of nodules by native rhizobia in roots of C. ternatea. Few studies have described the negative effects of petroleum hydrocarbons on rhizobial nodulation; overall nodulation decreases in those legumes exposed to petroleum hydrocarbons, either at controlled or natural conditions (Lindström et al., 2003; Rivera-Cruz et al., 2005; De Farias et al., 2009). At 240 days, nodulation in Clitoria plants showed certain recovery at 6000 mg kg-1. In this regard, petroleum hydrocarbons may promote the N-fixation by rhizobial nodules in legumes grown in contaminated boreal soils (Yan et al., 2015); however, further studies are needed to evaluate the effects of hydrocarbons on the nitrogenase activity under petroleum contamination. In this regard, the functionality of nodules in terms of leghemoglobin content (pink coloration) or nitrogenase activity was considered in the present study since the aim of this research was focused on the expression of nodules in roots due to the recovery of native rhizobia in the soil.
Either AMF colonization or number of spores decreased significantly as crude oil concentrations increased, which concurs with negative effects of petroleum hydrocarbons on AMF (Cabello, 1997; Gaspar et al., 2002; Franco-Ramírez et al., 2007; Bento et al., 2012; García et al., 2013; Driai et al., 2015). However, our results denote that AMF sporulation showed a recovery at 240 days (Figure 2b), and AMF colonization also increased in roots of Brachiaria when grown in treatments with 6000 to 12000 mg kg-1, at 240 days (Figure 3 b). The sporulation represents an AMF strategy to ensure their progeny under environmentally stressful conditions; moreover, AMF colonization may increase plant resistance to abiotic stresses (Harrier and Watson, 2004).
Phytotoxicity of crude oil resulted in decreased dry biomass of Brachiaria, and more dramatically in Clitoria, which was more susceptible to contamination, excepting at 60 days at 3000 mg kg-1 when its biomass increased, probably due to the nutrient availability provided for the proliferation of PSB, for instance. Excepting this, the results concur to those effects described for several plant species including non-legume or legume species under contaminated soils at greenhouse conditions (Adenipekun et al., 2008; Bento et al., 2012; Baruah et al., 2014; Kuo et al., 2014). Furthermore, legumes have been described as highly sensitive species than grasses to organic contaminants (Spiares et al., 2001; Pilon-Smits, 2005). Moreover, the presence of AMF and rhizobia in roots of legume species allows better tolerance and growth when grown under oil-contaminated soil, showing high AMF colonization and number of nodules. Our results suggest that effects of crude oil on microbial populations in the rhizosphere of grasses and legumes are time depending, because meanwhile the NFFLB, PSB, and AMF showed a stabilization and recovery tendencies along experimentation, but the nodule formation for rhizobia was depressed at 240 days.
Moreover, petroleum hydrocarbons result in positive impacts on symbiotic microorganisms in plants. The beneficial effects of culturable bacteria and symbiotic microorganisms on plant species also resulted in diminishing the content of petroleum hydrocarbons in mesocosms. Overall, petroleum degradation in the mesocosms ranged from 53 % at mesocosms contaminated with 3000 mg kg-1 to ~ 33 % in average for mesocosms with 9000 and 12000 mg kg-1, at the end of experimentation (data not shown).
Conversely, the total chlorophyll content was higher in Clitoria when compared to Brachiaria, denoting that Clitoria despite being highly sensitive to contamination, establishes symbiosis with rhizobia by which both N-assimilation and total chlorophyll content are improved, especially at concentrations below 12000 mg kg-1. Although the biomass of Brachiaria was greater than that achieved for Clitoria, the high content of total chlorophyll in this legume may be in part explained due to the accumulation of this photosynthetic pigment in reduced leaf area, whereas in Brachiaria this accumulation may be diluted in greater leaf area. In this regard, the symbiosis between nitrogen-fixers may promote the growth of legumes in contaminated soils with crude oil, in which the C/N ratio is generally high (Adam and Duncan, 2003). This bacterial benefit was additionally improved by AMF colonization over time, which could contribute on improving N and P plant uptake and consequently on plant growth (Tang et al., 2009; Wang et al., 2017). The chlorophyll content is a critical parameter used as an indicator of plant stress under adverse conditions (Dai et al., 2009). Nevertheless, total chlorophyll content may not be a suitable indicator of plant toxicity, but some reports indicate that organic contaminants may affect the content of photosynthetic pigments in plant species from terrestrial and marine ecosystems (Odjegba and Sadiq, 2002; Catriona et al., 2003; Njoku et al., 2008; Tanee and Akonye, 2009; Naidoo et al., 2010; Bento et al., 2012). However, crude oil concentrations impaired growth of both plant species.
CONCLUSIONS
The crude oil did not modify the populations of NFB and PSB along experimentation until 240 days. In contrast, at the beginning of the experiment, the contaminant significantly decreased the population of both rhizobia and AMF in the rhizosphere of C. ternatea and B. brizantha, but these microorganisms showed significant recovery at 240 days. Also, crude oil induced phytotoxic effects in both plant species, then limiting their growth. All microbial populations assessed in this research tend to increase over time, to show certain resilience to the contaminant, and thus, to sustain plant growth and fitness under these stressful conditions.