INTRODUCTION
The Bruchinae beetles are major pests worldwide; globally widespread species such as Zabrotes subfasciatus (Boheman, 1833) promote high levels of damages on stored bean grains (Phaseolus vulgaris L.), mainly at warehouses of developing countries in Sub-Saharan Africa and Latin America, where common beans are an important source of proteins (Tuda, 2007; Daglish, 2018; Tripathi, 2018). The larvae of Z. subfasciatus consumes grains internally and promotes high levels of damages on dried beans at warehouses resulting on severe economic losses to farmers (Gonçalves et al., 2017; Tigist et al., 2018). In Southern Ethiopia, for example, it is estimated that 44.6 % of the losses of common bean production occur during storage due to inappropriate infrastructure and the presence of Z. subfasciatus. And most farmers (48 %) apply botanical insecticides to control populations of the Mexican Bean Weevil (Mesele et al., 2019).
Unfortunately, chemical control of insect pests of stored products is practically restricted to phosphine, organophosphates and pyrethroid insecticides and many of these products are not easily accessible and available for farmers in developing countries (Boyer et al., 2012). Moreover, there are many reports of resistant insect-pest populations to synthetic insecticides worldwide (Pimentel et al., 2010; Zettler and Arthur, 2010; Boyer et al., 2012). Therefore, it is important to develop botanical insecticides with new modes of action that not only kill adults of Z. subfasciatus but also prevent its larvae to penetrate bean grains. Studies concerning the use of plant-based insecticides and resistant plant varieties have been performed in order to minimize the damages promoted by Z. subfasciatus (Ribeiro-Costa et al., 2007; Gonçalves et al., 2015; Gonçalves et al., 2017; Lüthi et al., 2018). The use of botanical insecticides can be an efficient and accessible tool to control infestations of Z. subfasciatus at warehouses, mainly for small farmers (Mulungu et al., 2007; Pessoa et al., 2016; Silva et al., 2016; Tamiru et al., 2016; Bayih et al., 2018; Bernardes et al., 2018; Zekarias and Haile, 2018).
Worldwide, many botanical insecticides such as neem oil, pogam oil, rotenone and essential oils have been sprayed on crops, and two major classes of insecticides, pyrethroids and neonicotinoids are based on plant insecticidal chemical compounds (Pavela, 2016; Sparks et al., 2017). Regarding botanical insecticides based on Solanaceae species, there are products such as Hot Pepper Wax (Vitova Insectaries, USA) formulated with Capsicum annum L., and Nico Dust (Nico Orgo Manures, India) based on Nicotiana tabacum L. (Pavela, 2016). Solanaceae is a promissory botanical family to discover new insecticidal molecules. It is widely distributed in both the temperate and tropical zones with about 2,300 species presenting secondary metabolites (flavonoids, alkaloids, whitanolides, capsinoids etc.) (Shonle and Bergelson, 2000; Alves et al., 2003; Martins and Barkman, 2005; Veleiro et al., 2005; Luo et al., 2011; Pinto et al., 2011; Ohyama etal., 2013). These secondary metabolites can promote different effects on insect pests, such as mortality, feeding deterrence and repellence (Chowanski et al., 2016). Recent studies demonstrated the efficacy of botanicals from Datura stramonium L. (Solanaceae) and Capsicum frutescens L. (Solanaceae) to control Z. subfasciatus (Bayih et al., 2018; Zekarias and Haile, 2018). Thereby, in the present study, it was performed toxicological bioassays with ethanolic extracts of Solanaceae species in order to evaluate their bioactivity against Z. subfasciatus. To perform a suitable selection of Solanaceae species it was adopted the following criteria: i) scientific data regarding their chemical diversity and bioactivity, ii) focus on including species from different genera (8 in total), and iii) plants that may be easily available for farmers.
MATERIALS AND METHODS
Collecting plant material and preparing ethanolic extracts
In the present research, 25 ethanolic extracts from 17 Solanaceae species were studied (Table 1). The selection of Solanaceae species used were based on i) scientific data regarding their chemical diversity and bioactivity, ii) focus on including species from different genera (eight in total), and iii) plants that may be easily available for farmers. The process adopted to obtain ethanolic extracts was cold maceration in ethanol solvent (analytical grade, 99.5 %). All plant parts were dried in an air flow drying oven (40 °C for 48 to 72 h) and separately drilled in a knife mill to produce a powder. These plant powders (200 g) were separately immersed in 1 L of ethanol (1:5 w/v), stirred for ten minutes, stored at 25 °C for 72 hours to finally be filtered with qualitative filter paper (80 g m-2, porosity of 3 Mm). Such process was repeated three times with each plant powder. Afterwards, the remaining solution (ethanol with extracted compounds from plant powders) was placed in a rotary evaporator at 50 °C and -600 mmHg in order to eliminate the ethanol solvent and acquire the plant extract (with paste or oil consistence).
Toxicological bioassays with ethanolic extracts from Solanaceae
The colony of Z. subfasciatus was initiated with individuals acquired from warehouses of Piracicaba municipality, São Paulo, Brazil. They were maintained in the laboratory under controlled conditions (25±2 °C, 60±10 % R.H. and a photoperiod of 14 L: 10 D hours) in glass containers (2.6 L) with P. vulgaris grains cv. 'Bolinha' as substrate for adults to lay their eggs.
It was employed five couples of Z. subfasciatus adults (aging 24 hours) per sample unit (100 insects per treatment) to perform residual contact toxicological bioassays. The treatments consisted of samples of bean grains treated with Solanaceae ethanolic extracts [(Petri dishes dimensions of 6.5 cm diameter x 2 cm high) with 10 g of bean cv. "Bolinha" per sample] in a completely randomized design, with 10 repetitions per treatment.
For each treatment, 100 g of beans were placed inside a plastic bag (2 L) and sprayed with an ethanolic extract using a microatomizer pistol coupled to a pneumatic pump adjusted to provide a spray pressure of 0.5 kgf cm-2 and a volume of 30 L t-1 [3 mL of solution (solvent + Solanaceae extract) per each 100 g of beans]. The solvents methanol and acetone were used to solubilize ethanolic extracts at a ratio of 1:1 (v/v). After applying ethanolic extracts (2,500 mg kg-1), treated beans were softly shaken for one minute to homogeneously distribute Solanaceae extracts on grains surface. Afterwards, they were kept in an airflow chamber during two hours for solvent evaporation before insects (five couples of Z. subfasciatus) were inserted in treated bean samples (10 g of beans). The concentration (2,500 mg kg-1) used to apply ethanolic extracts in toxicological bioassays was defined based on preliminary tests. The preliminary tests were performed adopting the same procedures described above but applying different concentrations (500-3,000 mg kg-1) of the fraction on bean grains.
For each experiment a negative control with acetone:methanol (1:1, v/v) was included. In addition, the botanical insecticide Azamax® 1.2 EC {azadiractin A/B [12 g.L-1 (1.2 % m/m)]} was included in bioassays to compare its activity with the ethanolic extracts. Azamax® 1.2 EC, a botanical insecticide registered in Brazil to control many insect pests, presents limonoids (azadiractin A and 3-tigloylazadirachtol) that can cause phagodeterrence and hormonal disbalance on insects. Azamax® 1.2 EC was applied adopting the same concentration (2,500 mg kg-1) used for spraying ethanolic extracts.
The mortality of adults and number of eggs per sample were assessed five days after infestation (adults were withdrawn from sample units), and insects were considered dead when they did not react to a brush touch after one minute. Moreover, the F1 progeny and damages on grains were assessed after 56 days of infestation. Bean grains were considered damaged when they presented galleries due to the feeding of insect larvae. The percentage of egg-adult viability was calculated based on the formulae: (n° of F1 individuals/n° of eggs) *100, for each repetition.
Data analysis
The data were analyzed with the software "R", version 3.3.1. It was used generalized linear models (GLM) with quasibinomial or quasipoisson family distribution together with a half-normal probability plot with simulation envelope to verify generalized linear models' fit (Nelder and Wedderburn, 1972; Demétrio and Hinde, 1997; Hinde and Demetrio, 1998). In the case of significant differences among treatments, a multiple comparison test (Tukey's test, p <0.05) was performed to identify such differences.
RESULTS
The Solanaceae ethanolic extracts affected Z. subfasciatus mainly by reducing the number of eggs per sample, the F1 progeny and the damages on bean grains (Table 2). The adults of Z. subfasciatus do not feed on bean grains; thereby reducing the number of eggs and inhibiting its F1 progeny are key aspects to avoid infestations at warehouses and damages on grains. Species from different genus promoted statistically significant effects on Z. subfasciatus, but at different intensities. None of the ethanolic plant extracts interfered on sex ratio, but many of them reduced egg-adult viability (Table 2).
Table 2 Bioactivity (mean ± SE) of ethanolic extracts from Solanaceae (tested at 2,500 mg kg-1) against Zabrotes subfasciatus, by residual contact bioassay. Temp.: 25±2 °C; R. H.: 60±10 %; Photoperiod: 14L:10D.
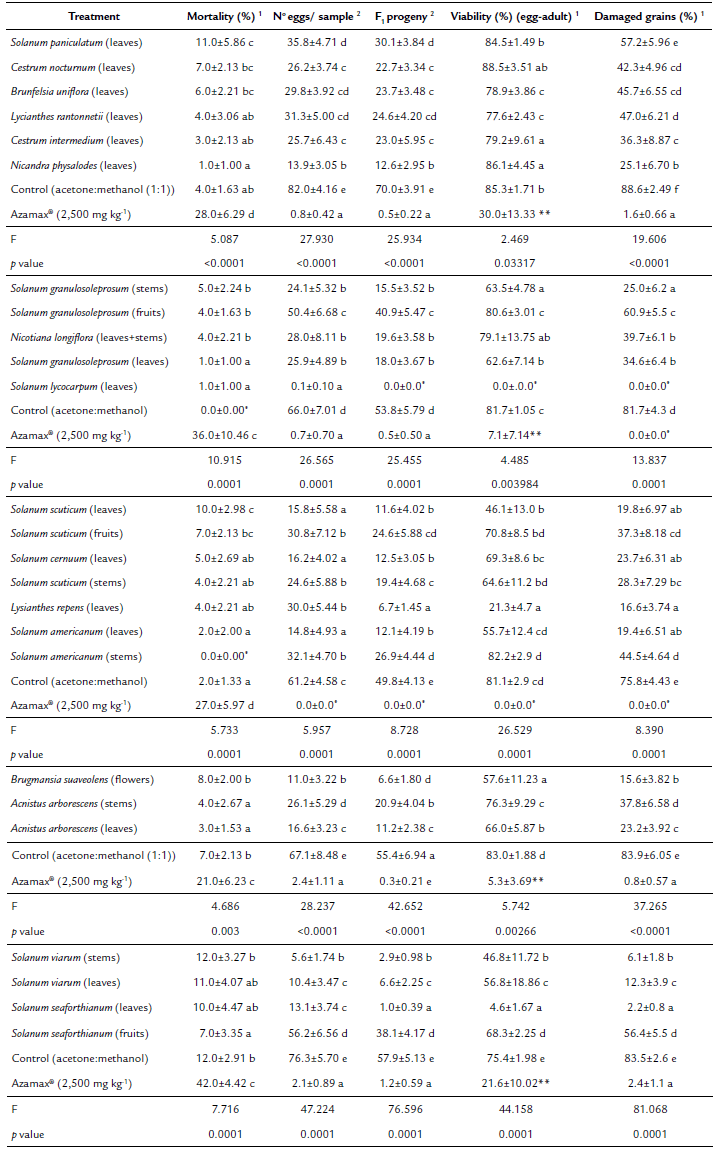
1Means followed by different letters within columns indicate significant differences between treatments (GLM with a quasi-binomial distribution followed by Tukey’s post hoc test, p< 0.05);
2Means followed by different letters within columns indicate significant differences between treatments (GLM with a quasi-Poisson distribution followed by Tukey’s post hoc test, p< 0.05);
* Not included in the analysis (null variance);
** Not analyzed due to small sample unit;
ns Not significant (p>0,05).
The extract of Solanum lycocarpum A. St.-Hil leaves almost reduced the number of eggs per sample to zero and completely protected bean grains against damages resulting from larvae feeding (Table 2). In addition, the extract from flowers of Brugmansia suaveolens (Willd.) Sweet provided a good protection of beans grains against Z. subfasciatus. It promoted an expressive decrease in the number of eggs per sample (11.0), the F1 progeny (6.6), egg-adult viability (57.6 %) and damaged grains (15.6 %) (Table 2). Only the extract of Solanumpaniculatum L. (leaves) promoted significant mortality on Z. subfasciatus adults comparing to the negative control, but it did not expressively reduce oviposition, F1 progeny and damages on grains comparing to other extracts (Table 2). However, some extracts (stems and leaves of Solanum viarum Dunal, and leaves of Solanum seaforthianum Andr., Solanum scuticum M.Nee, Solanum americanum Mill. and Lysianthes repens (Spreng.) Bitter) intensively reduced both the number of eggs per sample and the F1 progeny. They also promoted a moderate toxicity to Z. subfasciatus eggs expressed by the reduction in egg-adult viability and, consequently, by the reduction of damages on grains (Table 2).
Some ethanolic extracts such as Acnistus arborescens (L.) Schltdl. (leaves), Nicandra physalodes (L.) Gaertn. (leaves), Solanum granulosoleprosum Dunal (stems), Solanum cernuum Vell. (leaves) and Solanum americanum (leaves) promoted a good reduction of damages caused by larvae on bean grains, but less intensively than S. lycocarpum and B. suaveolens (Table 2). Nonetheless, the highest mortality was promoted by the positive control with azadiractin and it also presented grain-protective properties (Table 2).
DISCUSSION
Many toxicological studies with insecticidal plants to control Z. subfasciatus focus on terpenes such as limonoids (azadirachtin) and essential oils that act as fumigants (Weaver et al., 1994; Silva et al., 2008; Zewde et al., 2010; França et al., 2012; Brito et al., 2015; Jairoce et al., 2016; Bernardes et al., 2018). However, an advantage of nonvolatile compounds is that they can protect bean grains for a longer period and avoid new infestations of Z. subfasciatus. In general, Solanaceae ethanolic extracts affected Z. subfasciatus mainly by reducing the number of eggs per sample, the F1 progeny and the damages on bean grains (Table 2). Considering that only the larvae of Z. subfasciatus feed on bean grains, reducing its oviposition and F1 progeny is a strategically aspect to reduce damages on bean grains.
The ethanolic extracts of N. physalodes (leaves), S. scuticum (leaves) and A. arborescens (leaves) reduced oviposition to 13.9, 15.8 and 16.6 eggs.sample-1, respectively (Table 2). Females of Z. subfasciatus may have laid less eggs due to oviposition deterrence or due to active compounds disrupting egg formation. The insecticidal whitanolide nicandrenone produced by N. physalodes and cytotoxic whitanolides produced by A. arborescens may be responsible for the reduced oviposition (Bates and Eckert, 1972; Cordero et al., 2009; Roumy et al., 2010). Whitanolides promote feeding deterrence on insects and can antagonize ecdysteroid hormones (Dinan et al., 1996; Mareggiani et al., 2000). Earle et al. (1970) verified that some ecdysone analogues are able to induce sterility on females of Anthonomusgrandis Boheman (Coleoptera: Curculionidae). Solanaceae ethanolic extracts not only reduced the number of eggs laid on beans' surface but also the egg-adult viability of Z. subfasciatus (Table 2). The highest reduction of egg-adult viability (4.6 %) was performed by the ethanolic extract of S. seaforthianum (leaves) (Table 2). Differently from the above-mentioned species, S. seaforthianum produces glycoalkaloids (solasodine, solasonine and solamargine) and the reduction of egg-adult viability could be related to the contact of eggs with the treated surface of bean grains (Alsherbiny et al., 2018).
The bioactivity of Solanaceae extracts was species-specific and varied according to the plant-structure (leaf, stem, flower and fruit). Bean samples treated with the ethanolic extract from leafs of S. seaforthianum had 2.2 % of damaged grains whereas samples treated with its fruits extract had 56.4 %, for example (Table 2). The ethanolic extract from A. arborescens leafs (16.6 eggs.sample-1) reduced more the oviposition of Z. subfasciatus than its stem extract (26.1 eggs. sample-1) (Table 2). The ethanolic extract from stems (25 % of damaged grains) of S. granulosoleprosum presented better grain-protectant properties than its fruit ethanolic extract (60.9 % of damaged grains) (Table 2). This factor is also reported for Annonaceae species promoting toxicity on Z. subfasciatus. The ethanolic extract from leafs of Annona sylvatica A. St.-Hil. (Annonaceae), applied at 1,500 mg kg-1, did not promote mortality of Z. subfasciatus adults but promoted sublethal effects (11.9 eggs sample-1; 21.11 % of damaged grains) whereas the ethanolic extract from A. sylvatica seeds promoted 100 % mortality and completely inhibited damages on grains (Gonçalves et al., 2015). This species-specific bioactivity is probably due to the specific distribution of secondary metabolites among plant structures and tissues (Berenbaum, 1995). In addition, plants can inductively or constitutively produce secondary metabolites and their production suffers interference from biotic and abiotic factors (Mithõfer and Boland, 2012).
Based on the percentage of damaged grains, in the present study the most bioactive species tested against Z. subfasciatus was S. lycocarpum (leaves) (Table 2). The ethanolic extract from leafs of S. lycocarpum presented grain-protectant characteristics and completely inhibited damages on grains, despite the fact that it did not kill adults (Table 2). The powder from leafs of Datura stramonium (Solanaceae) (tested at 2,000 mg kg-1) promoted 71.28 % mortality of Z. subfasciatus adults after 5 days, but it did not inhibit F1 progeny (11.33 insects.sample-1) or avoid damages on bean grains (Weevil Perforation Index: 21.06) (Bayih et al., 2018). Nonetheless, in the present study, the ethanolic extract from S. lycocarpum (tested at 2,500 mg kg-1) did not promote significant mortality, but it inhibited F1 progeny and damages on bean grains (Table 2). It demonstrates that killing adults of Z. subfasciatus is not essential to avoid damages on grains and bioinsecticides with sublethal effects can be efficient.
S. lycocarpum naturally occurs in Brazilian savannas and degraded pastures (Bueno et al., 2002) and it produces the steroidal alkaloids solasonine and solamargine, two defensive allelochemicals (Miranda et al., 2012; Al Sinani and Eltayeb, 2017). These were able to block the larval development of Earias insulana (Boisd.) (Lepidoptera: Noctuidae) when incorporated in artificial diet at 0.2 % (Weissenberg et al., 1986); and they reduced the larval growth of Tribolium castaneum (H.) (Coleoptera: Tenebrionidae) at 1 urnol g-1diet (Weissenberg et al., 1998). Solasonine and solamargine present membrane-disrupting properties and can affect phosphatidylcholine/cholesterol liposomes (Roddick et al., 1990). The bioactivity promoted by the ethanolic extract from S. lycocarpum may be due to the presence of such alkaloids. Alkaloids share equal amino acid precursors with insect neurotransmitters enabling them to interact with neurotransmitters' molecular biding sites (Wink and Schimmer, 2018). Other species, such as S. viarum, presents the steroidal alkaloid solasodine (Mola et al., 1997; Shakirov et al., 2012); and B. suaveolens (flowers) produces the alkaloids hyoscyamine, atropine and scopolamine (Andreola et al., 2008). Nonetheless, in the present study it was verified nor high levels of mortality neither symptoms of neurotoxicity on Z. subfasciatus (Table 2). This absence of adult mortality may be due to low concentrations of alkaloids in ethanolic extracts of S. lycocarpum, B. suaveolens and S. viarum.
The present study provides good perspectives for exploring insecticidal compounds in Solanaceae species to produce a botanical insecticide for the management of Z. subfasciatus. Nonetheless, it is necessary to perform bioguided chemical fractionations with Solanaceae ethanolic extracts in order to identify which chemical compounds are responsible for the observed bioactivity against Z. subfasciatus. Moreover, it is also important to perform toxicological studies to verify the presence of toxic compounds to humans and non-target organisms. Many Solanaceae species present alkaloids that are toxic to humans (Smith et al., 2008; Chowanski et al., 2016). These studies are essential to assure safety for both farmers and consumers before recommending the application of a botanical insecticide on stored products. Ethanolic extracts are a simple, cheap and efficient way to extract and apply insecticidal chemical compounds from plants. Therefore, the ethanolic extract from S. lycocarpum might be an accessible solution to the management of Z. subfasciatus to small-scale agriculture.
CONCLUSIONS
Most of the 25 ethanolic extracts from 17 different Solanaceae species promoted significant interference on Z. subfasciatus oviposition, F1 progeny or egg-adult viability resulting in less damaged grains (Tables 1 and 2). The most promissory species was S. lycocarpum that completely inhibited the F1 progeny and damages on bean grains when applied at 2,500 mg kg-1. It demonstrates that Solanaceae species can be a source of botanical insecticides to control Z. subfasciatus.