INTRODUCTION
Mexico is one of the few mega-diverse countries on the planet. Despite having more than 23 000 species of vascular plants, few forest species have an industrial interest. Red cedar (Cedrela odorata L.) is the second most important tropical timber species in the forest industry in Mexico, only surpassed by mahogany (Swietenia macrophylla). It has a great economic potential for its excellent features and high commercial timber value (Estrada et al., 2016). Recent evaluations suggest that over 300 000 plant species are found worldwide and that every plant carries at least one endophyte (Smith et al., 2008). Indeed, endophytic microbes have been found in every plant species examined to date; Partida and Heil (2011), report that a plant without endophytes could only occur infrequently. It can be assumed that plants deprived of endophytes would be more vulnerable to environmental stress and pathogenic attacks (Khan et al., 2012; Leitão and Enguita, 2016; Suman et al., 2016; Brader et al., 2017).
Endophytic microorganisms are primarily bacteria and fungi that live within plants for at least part of their life cycle without causing apparent harm to the host. The various seed-borne bacterial endophytes found in plant tissues utilize either direct or indirect mechanisms to improve plant growth, development and enhance tolerance to biotic and abiotic stresses. In many cases, the microbes protect the plant hosts against diseases and insect pests (Santoyo et al., 2016; Shahzad et al., 2017a;b).
The internal environment of the seed changes during maturation, which consequently affects the seed endophytic community. The ability to inhabit a seed and adapt to severe environmental conditions are special characteristics of seed endophytes that are rarely found in endophytes isolated from roots, shoots, or other plant tissues. Seed endophytes can form endospores, thus protecting from changing conditions inside the seed (Compant et al., 2011; Kane, 2011). Seed-borne bacterial endophytes also participate in modulating endogenous phytohormones (Shahzad et al., 2016). Also, some plant growth-promoting bacterial endophytes can lower ethylene levels by synthesizing a catalyst, ACC deaminase (1-aminocyclopropane-1-carboxylate), of an ethylene precursor in higher plants (Shahzad et al., 2018). Verma et al. (2017), reported that Enterobacter asburiae, Pantoea dispersa, and Pseudomonas putida strains are endophytes of rice seeds, can produce indole acetic acid, solubilization of phosphates, and inhibit the growth of Fusarium oxysporum. They influence the growth and development of seedlings. biotechnological characteristics such as the production of indole acetic acid, siderophores, phosphate solubilization, nitrogen fixation, and ACC deaminase activity have also been reported in Bacillus strains isolated from seeds of four commercial varieties Lycopersicum esculentum Mill. (Xu et al., 2014).
Endophytic bacteria degrading polycyclic aromatic hydrocarbon (PAH) have been reported (Liu et al., 2016). The use of endophytes may offer advantages because the host plant provides a stable environment without interference from the native microflora and its ability to promote plant growth and reduce PAH content directly in plant tissues. Zhu et al. (2017) reported that Serratia sp. PW7, an endophyte isolated from Plantago asiatica, could degrade pyrene in vitro and in vivo; similar behavior was reported in Azospirillum sp. strains and the endophyte Pseudomonas stutzer isolated from Hordeum sativum, Zea mays L., and Leymus arenarius, which degraded anthracene, phenanthrene, and pyrene (Gałązka and Gałązka, 2015).
However, there is a lack of studies about the diversity of endophytic bacteria in Cedrela odorata L., seeds, which can promote the growth of plants that are of importance in biotechnology. Therefore, in this study, the objective was to isolate, characterize, and perform growth on aromatic compounds tests, growth promotion, and antagonisms of endophytic isolates of cedar (Cedrela odorata L.) seeds collected from trees in different regions of the state of Chiapas, Mexico.
MATERIALS AND METHODS
Seed collection
Seeds of Cedrela odorata L. were collected In the months of January and February 2019 from trees located in the municipalities of Huehuetán (15°01' N, 92°23' W), Motozintla (5°21' N, 92°14' W), and Pijijiapan (15°41' N, 93°12' W), Chiapas, Mexico.
Seed endophytes isolation
Endophytic bacteria were isolated from 100 seeds of Cedrela odorata L. as described below: the epiphytic microorganisms were eliminated by superficial disinfection of the seeds, washing with distilled water one min, ethanol 70 % one min, and sodium hypochlorite 3 % (v/v) three min; the excess sodium hypochlorite was removed by rinsing with sterile distilled water. Subsequently, the seeds were crushed and resuspended in 9 mL of a sterile 10 mM magnesium sulfate solution. Finally, serial dilutions of each sample were made and they were seeded in Petri dishes on peptone agar, and yeast extract (PY), with the following composition (g/L): 10, peptone; 5, yeast extract; and 18 agar, which were incubated at 28 °C for three days; the colonies that grew were selected. The effectiveness of disinfection was checked by placing seeds in Petri dishes with PY agar and incubated at 28 °C for five days.
Phosphate solubilization
Bacterial strains were inoculated into the PY liquid medium and incubated at 28 °C for 24 h with shaking at 200 rpm to obtain a pre-inoculum. The bacterial cultures were centrifuged at 10 000 g for five minutes and the optical density was adjusted to 0.2 absorbance units at 600 nm. The biomass collected was seeded in triplicate in NBRIP culture medium with the following composition (%): glucose, 1; Ca3(PO4)2, 0.5; (NH4)2SO4, 0.01; MgSO4.7H2O, 0.025; KCl, 0.02; MgCl2. 6H2O, 0.5; Congo red, 2.5 mg/mL; and agar, 1.8, and incubated at 28 °C for seven days. After this incubation period, the presence of translucent halos around the bacterial colonies was sought (Caballero et al., 2007).
Determination of auxin production
The strains were grown in liquid NFB medium with the following composition (g/L): malic acid, 5; K2HPO4, 0.5; MgSO4.7H2O, 0.2; NaCl, 0.1; CaCl2, 0.02; FeSO4, 0.015; Na2MoO4, 0.0025; MnSO4, 0.01; KOH, 4.8; NH4Cl, 0.2; yeast extract, 0.3; and H3BO4, 0.01; they were incubated for 18 h at 28 °C at 200 rpm. Subsequently, the cultures were adjusted to an optical density of 0.2 units at 600 nm. Finally, 100 μL of the cultures were inoculated in the Jain and Patriquin medium with the following composition (g/L): succinic acid, 2.5; fructose, 2.5; K2HPO4, 6;KH2PO4, 4; NH4Cl, 1; MgSO4, 0.2, NaCl, 0.1; CaCl2, 0.02; FeCl3, 0.01; NaMoO4, 0.002; and KOH, 2.1; with and without tryptophan (0.1 g/L) and incubated at 28 °C for 24 and 48 h at 200 rpm. One mL aliquots of the cultures were centrifuged for 5 minutes at 5000 x g, and 0.5 mL aliquots of the supernatant were taken and mixed with 0.5 mL of Salkowski reagent (Glickmann and Dessaux, 1995). The mixture was incubated in the dark at room temperature for 20 minutes; the absorbance at 530 nm was subsequently measured using a SmartSpec Plus spectrophotometer (Bio-Rad Laboratories Inc., Hercules, CA, USA). The presence of IAA was detected according to the method modified by Rahman et al. (2010). IAA concentrations were determined using a standard IAA curve (Sigma-Aldrich Corp.) from 0 to 50 μg/mL.
Antifungal activity
The antifungal activity of the endophytic strains was evaluated against the phytopathogenic fungi Fusarium sp., Alternaria solani, and the oomycete Phytophthora capsici. These phytopathogens were grown for seven days at 28 °C in PDA medium with the following composition (%): 0.4, potato extract; 2, dextrose; and 1.5, agar. Subsequently, 7 mm blocks containing mycelia were cut with a sterile scalpel and placed in the middle of a Petri dish with PDA medium. Endophytic cultures were scratched at two ends of the plate and incubated at 28 ± 2 °C for 48-96 h to evaluate the zone of fungal growth inhibition. The control consisted only of placing the 7 mm blocks of the fungus on PDA agar. The diameter of the mycelial growth was measured with a vernier to calculate the percentage of inhibition using the equation of Guo et al. (2006):
Mycelial inhibition (%) = [1 - (Da /Db)] * 100
Where:
Da = Diameter of the mycelial growth zone of treatments (mm)
Db = Diameter of the mycelial growth zone of the control (mm)
Growth on solid medium with aromatic compounds
Bacterial strains were grown in BSE liquid medium for 18 h at 28 °C with shaking 200 rpm. The cultures were centrifuged at 10 000 g, the supernatant was removed and the biomass was adjusted to an optical density of 0.2 to 600 nm. 100 μL of these cultures were inoculated in duplicate in SAAC medium with the following composition (g/L): K2HPO4, 0.4; KH2PO4, 0.4; MgSO4.7H2O, 0.2; CaCl2, 0.02; Na2MoO4, 0.002; FeCl2, 0.01; (NH4)2SO4, 0.5; bromothymol blue, 0.075; agar, 18; as the sole carbon source, 0.1 % phenol, 0.05 % benzene, 0.05 % phenanthrene, or 0.05 % anthracene. The plates were incubated at 28 °C for four days.
Molecular identification of the bacterial strains
Molecular identification was carried out on 26 strains that were selected based on the presence of one or more characteristics of biotechnological interest such as the production of indoles, phosphate solubilization, growth in aromatic compounds, and inhibition of phytopathogens. Genomic DNA of each bacterial strain was extracted using the ZR Fungal/Bacterial DNA Kit ™. The 16S ribosomal gene was amplified using the oligonucleotides rD1 and fD1 and the conditions described by Weisburg et al. (1991). The amplification products were purified from gel using the GeneJET kit (Thermo Scientific) and were sequenced at the Instituto de Biotecnología, Universidad Nacional Autónoma de México. The sequences obtained were deposited in the GenBank of the National Centre for BiotechnologyInformation (NCBI) under accession numbers: MN073815 (Cedo1); MN073829 (Cedo2); MN073809 (Cedo3); MN073825 (Cedo4); MN073826 (Cedo6); MN073818 (Cedo8); MN073806 (Cedo10); MN073807 (Cedo11); MN073827 (Cedo12); MN073830 (Cedo13); MN073824 (Cedo14); MN073817 (Cedo16); MN073823 (Cedo17); MN073810 (Cedo18); MN073811 (Cedo19); MN073820 (Cedo21); MN073822 (Cedo22); MN073813 (Cedo23); MNO73816 (Cedo25); MN073812 (Cedo26), MN073814 (Cedo28), MN073828 (Cedo31); MN073808 (Cedo33); MN073821 (Cedo35); MN075223 (Cedo36); and MN073819 (Cedo37). The 16S rDNA sequences were compared with the 16S rDNA genes in the GenBank database using BlastN and the phylogenetic analysis was performed using the program MEGA 6 (Tamura et al., 2013). The phylogenies were constructed using the neighbor-joining method (Saitou and Nei, 1987) based on 600 nucleotides for 16S rDNA, using the distance matrix of Jukes and Cantor (1969). The trees topology was bootstrapped 1000 times.
RESULTS
Isolation of endophytic bacterial strains of cedar seeds
From the cedar seeds of the selected trees, 62 strains were isolated which were named Cedo1 to Cedo62; they were evaluated to detect activities associated with growth promotion, antagonism against phytopathogenic fungi, and growth in xenobiotic compounds as described below.
Phosphate solubilization activity
Phosphate solubilization activity by microorganisms is very important because this activity can solubilize non-soluble phosphorus in soil. The phosphate solubilization activity of 62 endophytic bacterial strains was evaluated, resulting in 20 strains that could solubilize phosphate with diameter halos of 20-26 mm after seven days of incubation. The greatest diameter halo of phosphates solubilization was obtained with bacterial strain Cedo31 (Table 1).
Quantification of Indoleacetic acid
A direct mechanism that promotes plant growth is the production of auxins, mainly indoleacetic acid. Of the endophytic bacteria isolated from cedar seeds, 24 bacterial strains could produce IAA in a range of 3 to 97.6 μg/mL at 48 hours (Table 1). Bacterial strains Cedo3, (97.6 μg/mL), Cedo11, (96.5 μg/mL), and Cedo 37 (81 μg/mL) had the highest production of this hormone (Table 1).
Antagonistic activity against different phytopathogens
An indirect mechanism proposed to promote plant growth is the control of phytopathogenic fungi, which affects crop yields or even kills plants. With this as background, the ability of endophytes to inhibit the mycelial growth of phytopathogens, Fusarium sp., Alternaria solani, and the oomycete Phytophthora capsici was evaluated (Fig. 1). Of the 62 bacterial strains evaluated, 24 had antagonistic activity against at least one phytopathogen (Table 2). The strain Cedo13 inhibited the mycelial growth of the three phytopathogens, in 72 % for Alternaria solani, 62 for % Phytophthora capsici, and 54 for % Fusarium sp. This behavior was also presented by the strain Cedo22 inhibiting 75 % of the mycelial growth of Alternaria solani, 68 % of Phytophthora capsici, and 50 % of Fusarium sp.. The bacterial strain with the highest percentage of inhibition of mycelial growth against Alternaria solani and Fusarium sp. was Cedo36, with 76 % and 59 % of inhibition, respectively, while for Phytophthora capsici it was strain Cedo22 with 68 % (Table 2).
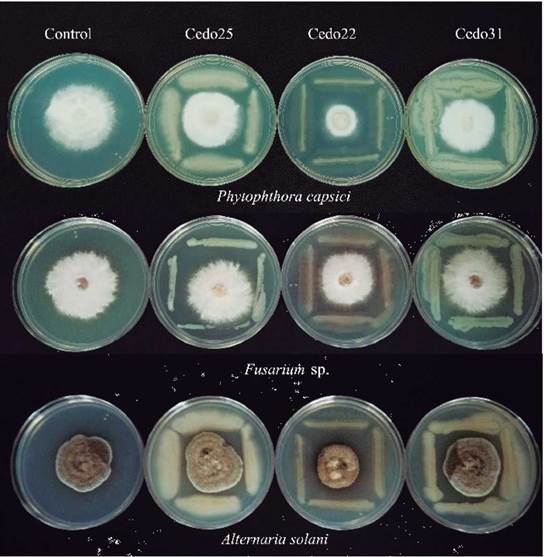
Figure 1 Antagonism ofthree isolates against pathogenic fungi. Control columns means that the fungus was inoculated without a phytopathogen. Row1: Phytophthora capsici; row 2: Fusarium sp.; row 3: Alternaria solani.
Growth in aromatic compounds
In this work, the ability of endophytic strains to grow in culture media containing benzene, phenol, anthracene, or phenanthrene as the only carbon source was evaluated. 26 strains grew in the presence of benzene and phenanthrene; 24 bacterial strains in anthracene and two in phenol, and only strain Cedo22 grew in the presence of the four aromatic compounds (Table 1).
Molecular Identification
The 16S ribosomal gene sequences of the 26 selected strains were analyzed by the Blast algorithm, where all strains were observed to have a high similarity with species of the Bacillus genus (Table 3). The phylogenetic tree was constructed using fragments of the sequences of the 16S ribosomal genes to confirm the identity of the isolates at the genus level. The cladogram shows that strains Cedo1, Cedo2, Cedo3, Cedo4, Cedo6, Cedo11, Cedo12, Cedo14, Cedo18, Cedo23, Cedo26, Cedo28, Cedo31, Cedo33, Cedo35, and Cedo37 are probably related to B. cereus, while strains Cedo8, Cedo10, Cedo13, Cedo16, Cedo17, Cedo19, Cedo21, Cedo22, Cedo25, and Cedo36 are probably a group of new species of Bacillus (Fig. 2).
Table 3 Possible genus of bacteria isolated from Cedrella odorata L seeds using amplified 16S rDNA sequences.
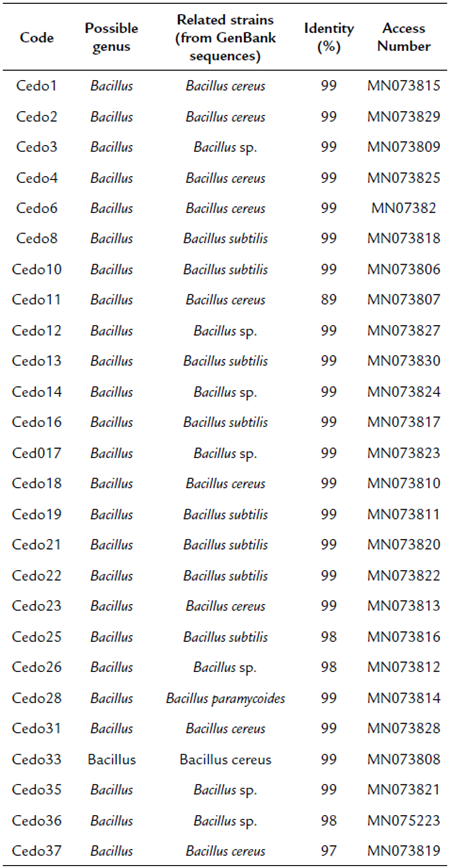
DISCUSSION
The endophytic microorganisms present in seeds are of great importance because they have a primary role in the control of pathogens, and they contribute to conserve and facilitate germination (Shahzad et al., 2018). In this study, 62 endophytic bacterial strains were isolated from Cedrela odorata L. seeds, collected in different geographical regions of the state of Chiapas, Mexico; 26 strains were shown to produce IAA and/or solubilize phosphate, which could promote plant growth, and 24 strains the ability to in vitro biocontrol phytopathogenic fungi. Phylogenetic analysis of the 16S ribosomal gene shows that the 26 strains selected from the 62 isolated in this work are related to the Bacillus genus. It is very interesting in this study that the characteristics of biotechnological interest were only detected in strains identified as belonging to the genus Bacillus. Another factor that could be related to the isolation of species of the genus Bacillus as endophytes of cedar seeds is that the PY culture medium was used, which favored the growth of one group of microorganisms over others, as reported by Lee et al. (2016), authors that used different culture media to selectively isolate microorganisms from the rhizosphere of Solanum lycopersicum. In the cladogram we can see that strains Cedo1, Cedo2, Cedo3, Cedo4, Cedo6, Cedo11, Cedo12, Cedo14, Cedo18, Cedo23, Cedo26, Cedo28, Cedo31, Cedo33, Cedo35, and Cedo37 are related to B. cereus, whereas bacterial strains Cedo8, Cedo10, Cedo13, Cedo16, Cedo17, Cedo19, Cedo21, Cedo22, Cedo25, and Cedo36 are probably a group of new species of Bacillus (Fig. 2). However, it is necessary to use another molecular marker such as the rpoB gene to identify them at the species level (Fazzeli et al., 2012).
According to our review, this is the first report of strains probably related to isolated B. cereus as endophytes of Cedrela odarata seeds. However, isolates of species of the Bacillus genus have been reported as endophytes in seeds of Arachis hypogaea, Phaseolus vulgaris, Lycopersicum esculentum, Zea mays, Cucurbita pepo, Vitis vinifera, Triticum aestivum, and Oryza sativa (Compant et al., 2011; Fürnkranz et al., 2012; Rosenblueth et al., 2012; Sobolev et al., 2013; Shahzad et al., 2016).
A characteristic detected in endophytic strains was the production of indoleacetic acid, the main auxin involved in cell division, while its function in seeds and tubers is to stimulate germination. In this work, the production of this auxin was quantified and it was found that strains Cedo3 (97.6 μg/ mL), Cedo11 (96.5 μg/mL), and Cedo37 (81 μg/mL) had the highest production (Table 1). These IAA concentrations are higher than those reported by Sánchez et al. (2019) in endophytic strains of Mimosa pudica nodules (Spaepen and Vanderleyden, 2011). IAA production has also been reported in endophytes isolated of Oryza sativa, Triticum aestivum, and Phragmites australis (Díaz et al., 2016; Verma et al., 2017; White et al., 2017). Although IAA production is important for seed germination, root growth, and nodulation, in high concentrations it can act as a bioherbicide, as reported by Park et al. (2015) in Enterobacter sp. I-3.
Phosphate solubilization is a mechanism that microorganisms use to transform phosphorus from insoluble to soluble forms by participating in the biogeochemical cycle processes of this element. This ability to solubilize phosphate has been reported in endophytic bacteria isolated from Oryza sativa, Triticum aestivum, and Phragmites australis (Díaz et al., 2016; Verma et al., 2017; White et al., 2018). In this paper, 20 endophytic bacterial strains isolated from cedar seeds show the ability to solubilize phosphate, so these strains could promote plant growth through this mechanism. The largest solubilization halo (26 mm) was observed in the bacterial strain Cedo31; it was greater than strains of genus Pseudomonas isolated from Jatropha curcas rhizosphere with 4.5-9.6 mm diameter of solubilization halo, reported by Wong et al. (2015) (Table 1).
The ability to contribute to the promotion of plant growth and stress tolerance in host plants from the action of seed endophytes, as well as reducing or preventing damage by fungi, bacteria, viruses, and in some cases even damage caused by insects and nematodes is very important (Shahzad et al., 2018). Of the 62 bacterial strains isolated from cedar seeds that were evaluated against the three phytopathogenic fungi, Alternaria solani, Phytophthora capsici, and Fusarium sp., only 24 strains inhibited at least mycelial growth from one of the phytopathogens evaluated; their inhibition range was between 37 to 87 %. The bacterial strains Cedo13 and Cedo22 inhibited mycelial growth of the three phytopathogens (Table 2). This characteristic of antifungal activity has also been reported in other isolated endophytes of Mimosa pudica, Paullinia cupana, Phragmites australis, Arachis hypogaea, Cucurbita pepo, and Zea mays (Fürnkranz et al., 2012; Sobolev et al., 2013; Santos et al., 2016; Verma et al., 2017; White et al., 2017; Sánchez et al., 2019). It has also been reported that Bacillus cereus B25 has antifungal activity against Fusarium verticillioides in maize plants (Martínez et al., 2016). Banerjee et al. (2017) reported Bacillus cereus IB311 with an antagonistic effect against Pseudomonas syringae and Agrobacterium tumefaciens on plants of Arachis hypogaea var. Koushal G201 and Sesamum indicum var. Kanak.
A relevant feature found in this work is the ability of 26 endophytic strains to grow in the presence of some aromatic compounds such as phenol, benzene, phenanthrene, or anthracene as the only carbon source in the culture medium, while only strain Cedo22 grew in the presence of these four compounds (Table 1). What is very interesting about this strain is that in addition to growing in the presence of these aromatic compounds, it also produces IAA, solubilizes phosphate, and inhibits the growth of phytopathogenic fungi. These results open the possibility of using this strain not only as a promoter of plant growth, but also in bioremediation processes, as reported from some isolated endophytic strains of Elymus dauricus, Populus, Salix, Lupinus luteus L., Plantago asiatica, Hordeum sativum, Zea mays L., and Leymus arenarius, which can degrade toluene, benzene, anthracene, phenanthrene, pyrene, and other volatile organic compounds (Siciliano et al., 1998; Barac et al., 2004; Taghavi et al., 2005; Germaine et al., 2006; Gałązka and Gałązka, 2015; Zhu et al., 2017). It has also been reported that the inoculation of B. cereus strains in Helianthus annuus stimulates the accumulation of Cd and Ni in the leaves of this plant (Khan et al., 2018). This growth characteristic in aromatic compounds has also been reported in Bacillus thuringensis FQ1, which degrades 95 % of phenanthrene when added with Pleurotus cornucopiae in soils contaminated with Cadmium, and Bacillus cereus as a pyrene degrader (Kazunga and Aitken, 2000; Jiang et al., 2015).
CONCLUSIONS
In this research, endophytic bacteria were isolated from Cedrela odorata seeds from different geographic regions of the state of Chiapas, Mexico. This is the first report of isolates of the genus Bacillus on cedar seeds. Approximately 40 % of the isolates could solubilize phosphate, produce indole, grow on xenobiotic compounds, and had antifungal activity. The phylogenetic analysis shows that strains identified by 16S ribosomal gene sequence are probably related to Bacillus cereus species, while a group of these strains are probably new Bacillus species. These strains are good candidates to be evaluated as plant-growth promoters, biological control agents against phytopathogens, and also grown in presence of xenobiotic compounds.