INTRODUCTION
Vascular epiphytes, which represent around 10 % of the world's flowering plants (Zotz, 2013), are an important part of ecosystems because they increase biodiversity by intervening in water and nutrients cycles, and by providing important sources of food, water, and shelter for numerous organisms (Díaz et al., 2012; Van Stan and Pypker, 2015; Brandt et al., 2017; Seidl et al., 2020). Given the complexity and quality they provide to their habitats; they are considered secondary foundation species (Angelini and Silliman, 2014).
Epiphytes have developed several adaptations, that allow them to survive in the canopy of trees, characterized by abrupt temperature changes and low availability of water and nutrients (Benzing, 1990; Zotz, 2016). Many of these adaptations are related to the capture, storage, and efficient use of water and nutrients since epiphytes are not in direct contact with the ground, which is the main reservoir of these resources for most plants. Alternatively, epiphytes are limited to the cortical and foliage runoffs of their host trees, rainwater, dew, and fog, as sources of water and nutrients (Benzing, 1990; Cardelús and Mack, 2010; Wu et al., 2018; Mendieta-Leiva et al., 2020). Given this strong correlation between epiphytes and the availability of water and atmospheric nutrients, different authors have pointed out that this life form will be among the most affected by the ongoing climate change (Lugo and Scatena, 1992; Benzing, 1998; Zotz and Bader, 2009).
Since 1990, phenology has been one of the most active disciplines to evaluate the effects of climate change on ecosystems (Piao et al., 2019). It is defined as the study of recurrent events during the life cycle of living beings, along with the causes of their occurrence concerning biotic and abiotic factors (Lieth, 1974). Phenological studies allow us to understand the effects of climate change as well as the availability of resources for pollinators and dispersers (Kebede and Isotalo, 2016; da Silva Freitas et al., 2017), ecosystem productivity (Richardson et al., 2010; Chang et al., 2013), and ecological processes such as competition and herbivory (Ekholm et al., 2019; Hood et al., 2019), among others. Phenology is also important for the development of conservation plans because it allows the development of germplasm collection calendars for both in situ and ex situ conservation. Moreover, phenology also contributes to the development of management plans for ecosystems and agroecosystems, which ensure the continuous availability of resources for pollinators, thereby sustaining pollination ecosystem services, and which make possible the sustainable harvest of non-timber forest products, among other benefits (Morellato et al., 2016; Buisson et al., 2017).
Unfortunately, this area has been poorly studied on vascular epiphytes (Williams-Linera and Meave, 2002; Morellato etal., 2010; Sakai and Kitakima, 2019). This led us to conduct this revision in hope of encouraging the phenological study of this group of plants. Our purpose was to synthesize the existing information and detect gaps in knowledge, suggesting future lines of study with emphasis on the particularities of the epiphytic environment.
MATERIALS AND METHODS
We conducted an online search for indexed articles that included reports on reproductive phenology (flowering and fructification) and vegetative phenology (leaves and pseudobulb production) of vascular epiphytes. We only considered studies made on holoepiphytes (plants that develop their full life cycle on their hosts according to Zotz (2013)).
Our search considered phenological studies published between 1800 and 2020, using the Google Scholar search engine and the ISI Web of Science database. Both resources are updated regularly and offer results that include trustworthy scientific documents that have been cited by numerous authors. To narrow down our search we used the following keywords, both in English and Spanish: vascular epiphytes x phenology, orchids x phenology, bromeliads x phenology, phenology x (holoepiphyte genus) including pteridophytes, community x phenology, and life forms x phenology. Since studies on food availability for pollinators usually describe the phenology of the plants they forage on, we also included the following keywords in our search, related to groups which have been reported as epiphyte pollinators: floral resources x hummingbirds, floral resources x bats, floral resources x moths and floral resources x euglossine bees (Gentry and Dodson, 1987; Ackerman, 1989; Benzing, 1990). Regarding resource availability for dispersers, we only included the following families in our search: Cactaceae, Araceae, Ericaceae, Gesneriaceae, Piperaceae, and Bromeliaceae, this is because the majority of epiphytes are anemochorial (Madison, 1977; Zotz, 2016), and the mentioned families are those with the higher number of animal-dispersed epiphyte species. We used a combination of the name of the families with the words fruit resources as keywords (e.g Cactaceae x fruit resources). Lastly, our search also covered articles about breeding systems since they report phenological information on the evaluated species. To find these studies, we searched for the following keywords: breeding systems x vascular epiphytes, breeding systems x family with epiphytic members.
To identify omissions in the vegetation types where epiphyte phenology has been studied, we extracted the coordinates reported in each study and superimposed them over the world's biomes layer available at https://ecoregions2017.appspot.com/, using the ArcGis 10.3© software.
RESULTS AND DISCUSSION
We found 107 papers with phenological information on vascular holoepiphytes (Supplement 1). Of these, 92 (88 %) were studies conducted in the Neotropic (Fig. 1), the area with the highest epiphyte diversity in the world; eight (7 %) reported data from Asia and the Pacific which hold the second place in terms of global epiphyte diversity, and four (4 %) were localized in Africa, the continent with the lowest epiphyte diversity (Madison, 1977; Benzing, 1990; Zotz, 2016), and one in Australia (1 %), where vascular epiphyte flora has partially been explored (Wallace, 1981). This totals 105 articles after excluding the studies by Barve et al. (2015) and Hietz (2010), since the first used herbarium data for all the Americas and therefore extends beyond the Neotropical area, and the latter is a report about xeric fern species from all around the world.
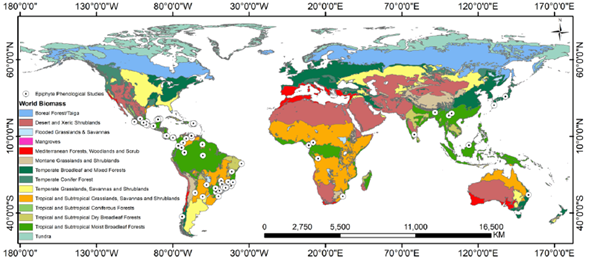
Figure 1 Geographic distribution of phenological studies that include vascular epiphytes and the biomes associated to these studies. Some points overlap because several studies were conducted at the same site.
Most of the studies found in our search were conducted in wet (76 %) or dry tropical and subtropical forests (Fig. 1). These forests hold the highest diversity of vascular epiphytes and cover about 17 % of the world's total area (Zotz, 2016; Dinerstein et al., 2017). While some temperate forests could compare to tropical ones in terms of epiphyte diversity and biomass (Zotz, 2005), we only found four studies done in these kinds of ecosystems (one in Chile and three in Japan). One explanation for the scarcity of studies in these types of forests could be that vascular epiphyte communities in temperate forests are dominated by ferns and similar species, while most phenological studies have focused on the reproductive biology of epiphytes with flowers (Fenner, 1998; Williams-Linera and Meave, 2002), and ferns are rarely included in the extensive vegetative phenology studies that have been done in temperate zones (Polgar and Primack 2011; Müller et al., 2019).
When it comes to taxonomic representation (Fig. 2) following the classification by Zotz (2013) for vascular epiphytes, we can see that only 33 % of the families with epiphytic members (24/73), 16 % of the genera (149/911), and ca. 2 % of the species (418/22 905) have been studied. Most of these studies focused on the Orchidaceae (46 %, 192 spp.) and Bromeliaceae (30 %, 124 spp.), showing an over-representation of these families which has also been reported for demographic studies of vascular epiphytes (Mondragón et al., 2015). Many reasons could explain this bias: a) These two families hold more than 75 % of all vascular epiphyte species (Kress, 1986; Zotz, 2013), b) Since epiphyte members of these families represent an important source of food for both birds and insects they get included in many foraging studies (Sazima et al., 1995; Sazima et al., 1996) and c) Many orchid and bromeliad species are widely collected for various purposes (ornamental, medicinal, etc.) which have caused them to become endangered, favoring their study over other non-endangered species (Bonato and Muraro, 2006; Parthibhan et al., 2015).
The study by Nevling (1971) was the oldest we found on epiphyte phenology. From then on, there has been a considerable increase in vascular epiphyte research, particularly in the last two decades that concentrate 69 % of all the studies we found. This recent rise matches the period when studies in tropical rain forests flourished, around the beginning of the 21st century (Williams-Linera and Meave, 2002; Piao etal., 2019). However, the incorporation of epiphytes in the mentioned studies has not been easy. For example, in one of the pioneer studies on tropical phenology, conducted at Barro Colorado Island, which included 1181 species of plants -trees, shrubs, herbs, and climbing plants (Croat, 1969), no epiphyte species was considered. It was not until 1975, in his article Phenological behavior of habitat and habitat classes on Barro Colorado Island, where Croat analyzed the phenological patterns of 41 epiphyte species. This resistance to incorporate epiphytes in phenological studies persists to this day, much of it related to the logistical challenge involved in monitoring individuals that grow on trees, often at heights over 40 m off the ground (Sheldon and Nadkarni, 2015).
The studies that present information regarding the phenology of vascular epiphytes can be grouped in the following categories (Supplement 1):
a) Studies about the reproductive biology of one or various species: here we included studies regarding breeding systems, pollination biology, and germination. In this group of studies, the phenology of plants is reported as complementary information. Forty-six of the studies (43 %) we found can be included in this category. Examples of this are Borba and Braga (2003) and Bianchi and Vesprini (2014) with their work on breeding systems; Canela and Sazima (2003) and Aguilar-Rodríguez et al. (2016) on pollination biology; and Duarte et al. (2018) studying germination.
b) Studies about resource availability for pollinators or dispersers: in this kind of study, a yearlong investigation is done on the phenology of species that serve as resources for a specific group of animals. Sixteen of the studies we found were of this kind (15 %). As examples, we have the work by Sazima et al. (1995) and de Araújo et al. (2011) which evaluate resources for hummingbirds.
c) Studies of the phenology of plant communities: these studies follow the phenology of a group of species, generally including different life forms, to establish phenological patterns and then compare between them, evaluating possible factors to explain them. We found 24 studies that included epiphytes (22 %), but four of these, despite mentioning epiphytes in their methods, excluded them from their results and discussion or grouped them with hemiparasites, parasites, and climbers for their analysis. Examples of studies that clearly show variation between phenological patterns of epiphytes and other life forms include Nevling (1971) and Marques et al. (2004).
d) Studies on vascular epiphyte phenology: these track the phenology of one or various epiphyte species, aiming to point out the factors that might be triggering it. We found 21 studies of this nature (20 %), 20 of which evaluate reproductive phenology and only one that gives relevant information about vegetative phenology, focusing on ferns (Hietz, 2010).
A. Vegetative phenology of vascular epiphytes
Phenological plant studies can focus on vegetative or reproductive phenology. The former includes dormancy and events of leaf production and leaf falling, and the latter includes flowering, fructification, and seed dispersal (Williams-Linera and Meave, 2002; Guaraldo et al., 2013; Tang et al., 2016). For vascular epiphytes, we found that most studies (96 % of our search results) are related to the reproductive phenology of these plants. The scarce research done on epiphyte vegetative phenology could be related to the fact that most species are evergreen (Benzing, 1990) with constant production of leaves, thereby impeding the distinction and tracking of the beginning and end of vegetative phenophases (Denny et al., 2014). Even so, we did find five studies that tracked the vegetative phenology of deciduous epiphytes, particularly for orchids and ferns. In orchids, research has been done on the phenology of pseudobulb formation, which is tied directly to the formation of leaves on the lateral or superior side of the pseudobulbs once they reach their final size (Dressler, 1993). Foliar phenology has been monitored in epiphytic ferns where leaves (or frond) production is restricted to a certain part of the year. Fronds are the most conspicuous parts of these plants and are responsible for energy transfer and spore production, both of which contribute to fern growth, survival, and regeneration (Mehltreter and Sharpe, 2013; Lee et al., 2018). Nonetheless, the fact remains that phenological data on epiphyte ferns is very scarce and, up to 2020, we found information for only 14 (Lee et al., 2018) out of 2700 species of epiphytic ferns (Zotz, 2013).
B. Patterns of flowering phenology in vascular epiphytes
Just as with other life forms, vascular epiphytes present phenological variation at the level of individuals, populations, and communities (Texier et al., 2018). In their paper about phenological patterns, Williams-Linera and Meave (2002) mentioned that these phenological differences might be related to the physiological status or location of the plants in specific micro-habitats, also considering the effect of the genotype (Primack, 1980). The epiphytic environment presents a large variation in terms of possible micro-habitats, derived from a wide range of characteristics such as host tree species and position along the host tree (Johansson, 1974; Wagner et al., 2015; Rasmussen and Rasmussen, 2018). The diversity of microhabitats could favor phenological variation among epiphytic individuals. While this effect has not been measured on phenology itself, it has been proven that different micro-habitats cause variation in the growth, reproductive potential, and flower production of vascular epiphytes (Cervantes et al., 2005; Ticktin et al., 2016; Ramírez-Martínez et al., 2018).
At the population level, epiphytes have non-random flowering patterns (Johansson, 1974; Gardner, 1986; Sahagún-Godínez, 1996; Hietz et al., 2006; Machado and Semir, 2006; Texier et al., 2018). Individuals produce flowers within less than five months per year, and most ofthem flower around the same time, which is why they are considered to flower annually, following the classification by Newstrom et al. (1994), and they mostly have seasonal blooming. Some species bloom during rainy seasons (Sazima et al., 1995; Aragón and Ackerman, 2004; Stevenson et al., 2008), others in dry seasons (del Coro Arizmendi and Ornelas, 1990; de Araújo et al., 2011; Orozco-Ibarrola et al., 2015), while others during both seasons, usually at the end of the dry and beginning of the wet season (Sazima et al., 1996; Buzato et al., 2000; Zimmerman et al., 2007).
There have been reports of variation in the duration or in the starting date of blooming among populations located in different regions. For example, in Machado and Semir's (2006) research about the flowering phenology of ornithophilic bromeliads in a tropical forest, they mentioned that eight of the fourteen monitored species had flowering periods that differed from those reported in other studies done in different areas of the same forest (Araujo et al., 1994; Buzato et al., 2000). This variation could be the result of temporal or micro-spatial differences among study sites. However, Texier et al. (2018) has reported the existence of phenological ecotypes in epiphytic orchids, in which their rhythms are genetically determined and are not influenced by habitat conditions.
At the community level, flowering in epiphytes tends to be continuous (Newstrom et al., 1994), meaning that throughout the whole year, different species can be found flowering, regardless of any possible peaks existing at the community level. For example, Johansson (1974) reported two flowering peaks for orchids during the dry season in eastern Africa, while Sahagún-Godínez (1996) mentioned that epiphytic orchids in western Mexico have a flowering peak at the beginning of the rainy season and another during the dry season. In the case of bromeliads, Machado and Semir (2006) reported they have sequential and continuous flowering with a peak during the rainy season.
When compared to other life forms, epiphytes shared similar flowering patterns with trees, shrubs, vines, and climbers (Croat, 1975; van Dulmen, 2001; Ramírez, 2002; Marques et al., 2004; Liebsch and Mikich, 2009), and in some cases also with terrestrial herbaceous plants (Marques et al., 2004; Cascante-Marín et al., 2017). Epiphytes might show marked flowering seasonality, similar to other life forms, but with shorter duration during the dry season (Croat, 1975; van Dulmen, 2001; Ramírez, 2002; de Freitas et al., 2013). Conversely, other epiphytes have shown low seasonality and extensive flowering, when compared to other life forms, as documented by two studies conducted north of the tropic of Capricorn, where seasonal differences were almost nonexistent (Smith-Ramírez and Armesto, 1994; Marques et al., 2004).
1. Determining factors on flowering phenology of vascular epiphytes
Plant phenology is commonly tied to abiotic (proximate factors) and biotic (ultimate factors) triggers (Lopezaraiza-Mikel et al., 2013). Proximate factors include temporal variation in photoperiods, precipitation and temperature, among others, and ultimate factors are related to phylogenetic relations, biotic interactions such as competition among pollinators, and herbivore evasion (van Schaik et al., 1993; Wright and Calderón, 1995; Lobo et al., 2003).
From the 107 studies, we found only five that explicitly explore the correlation between flowering phenology and proximal factors considering: maximum temperature, minimum temperature, precipitation, relative humidity, and/or solar radiation (Lasso and Ackerman, 2003; Marques et al., 2004; Ramírez and Briceño, 2011; Barve et al., 2015; Cascante-Marín et al., 2017). The possible effects of these correlations are only discussed in the Texier et al. (2018) study, while the effects of pollinators on flowering is only mentioned in the studies by Zimmerman et al. (1989) and Cascante-Marín et al. (2017).
The following sections were based on all the results obtained from our documental search. These can be broadly divided into studies that evaluate how proximate and ultimate factors might be regulating epiphyte phenology, and all the rest which include epiphytes, either directly in their discussions or indirectly through climographs (25 studies).
Proximate factors
In seasonal tropical environments, precipitation has been widely documented as a trigger for phenological events in numerous life forms (Sakai, 2001; Morellato et al., 2013). Unlike terrestrial plants, epiphytes don't have access to either the water or the nutrients stored in the ground and depend on atmospheric sources to obtain them. Consequently, water availability is considered the most relevant abiotic restriction for epiphyte growth and survival (Benzing, 1990; Zotz and Hietz 2001; Mondragón et al., 2015; Zotz, 2016). Taking this into consideration, one could expect vascular epiphyte phenology to be heavily influenced by precipitation availability, following the climate factor hypothesis (Wright and Calderon, 1995; Boulter et al., 2006) or, in other words, epiphyte phenology might have evolved to coincide with the period with higher water availability, and, thus, increased humidity and nutrient availability (Cascante-Marín et al., 2017).
However, although we find that in seasonal forests, ca. 47 % of epiphyte species flower during the rainy season, ca. 41 % do so in the dry season. This could be the result of various factors: a) According to the biological hypothesis, in which phenology is linked to the activity of pollinators (among other biotic interactions), the species that compete for pollinators tend to shift their flowering periods, thereby minimizing overlap and reducing competition. This would be the case for many epiphytes where, according to Ackerman (1986), one of the strategies adopted to handle the limitations imposed by their environments (individuals being far apart, few resources available to reward pollinators, and small size that limits their detectability) is to shift their flowering periods concerning other life forms, such as trees (Stiles, 1978). b) Phenophases are not disconnected from one another and involve compromises between them. This would mean that flowering during the dry season is more related to the fact that seeds benefit from being dispersed in seasons that favor germination and the establishment of seedlings (Primack, 1987). c) Restrictions due to growth forms. Zimmerman et al. (1989) mention how some species of the genus Mormodes Lindl. can flower during the dry season, when the population density of their pollinators is at its highest because their inflorescence emerges from pseudobulbs produced during the previous growing season. Meanwhile, Catasetum viridiflavum Hook., which shares the same pollinators with the Mormodes spp. mentioned, can't flower in the dry period because their inflorescence emerges from pseudobulbs produced during the same growing season, and this delays their flowering until newer pseudobulbs are formed, which happens around the middle and end of the rainy season.
In temperate environments, the temperature has been considered one of the main triggers for phenological events (Prevéy et al., 2017; Flynn and Wolkovich, 2018; Reed et al., 2019). However, in the tropics, where most epiphytes are found, attention is mostly turned to rain seasonality, ignoring changes in temperature and photoperiods because of their low annual variation (van Schaik et al., 1993; Morellato et al., 2000; Sakai, 2001). One of the few studies that evaluate factors that might affect the flowering phenophase in vascular epiphytes reported that an increase in solar radiation combined with a decrease in minimum temperature, are environmental cues that affect the floral phenology of Werauhia sintenisii (Baker) J.R. Grant (an epiphytic bromeliad growing in a cloud forest). However, the way these two factors influence floral induction remains unknown (Lasso and Ackerman, 2003).
Day duration has been associated with flowering induction in other life forms (Morellato et al., 2000; Rivera and Borchert, 2001) and, although there is little annual variation in photoperiod in the tropics, it could be enough to influence epiphytes, as observed for other phenological events. However, we could not find any studies investigating the potential influence of day duration on epiphyte phenology. Lasso and Ackerman (2003), as well as Cascante-Marín et al. (2017), propose that solar radiation has a positive influence on flowering. This factor could influence the flowering phenology of epiphytes in seasonal forests given that, as pointed out by Sahagún-Godínez (1996), the drought-tolerating adaptations developed by these plants, make them photosynthetically inefficient, so that it is favorable for them to flower in the season with higher solar radiation when they can have higher photosynthetic rates and flower production. However, this hypothesis has yet to be proven.
Ultimate factors
Several authors have insisted on the importance of biotic factors as selective triggers, which have molded the periodicity of phenological events for plants in the tropics (Stiles, 1978; Augspurger, 1983; Marquis, 1988).
The interactions that have received the most attention in this regard are herbivory, competition for pollinators, and diaspore dispersion (Fenner, 1998; Williams-Linera and Meave, 2002). In the case of vascular epiphytes, and according to our revision, interactions with pollinators have received the most attention and have been mostly studied in the Orchidaceae and Bromeliaceae families (Carranza-Quiceno and Estévez-Varón, 2008).
It has been hypothesized that competition between species for pollinators can be reduced by avoiding the overlap of their flowering periods ("Hypothesis of the shared pollinator"; Wright and Calderon, 1995), but this has barely been explored when it comes to vascular epiphytes. A study that stands out in this respect is the one by Sheldon and Nadkarni (2015) where they looked at the floral phenology of a community of vascular epiphytes in a tropical forest. Although they did not directly evaluate the competition for pollinators, they found asynchrony in the flowering periods among epiphyte groups that shared a common kind of pollinator. Species pollinated by insects had flowering peaks during the dry season, which is thought to be a mechanism used to maximize the presence of pollinators while reducing the competition for them (Talavera et al., 2001). On the other hand, species pollinated by birds flowered at the end of the rainy season, which correlates with the migratory habits of the birds. Another relevant study is the one by Cascante-Marín et al. (2017) where they reported asynchrony in the flowering of some genera that shared a common pollinator, including Monstera Adans., Peperomia Ruiz & Pav., Tillandsia L. and Werauhia J. R. Grant. The authors emphasize that in order to prove the shared pollinator hypothesis, it is necessary to first establish that the group of species does share the same pollinator, and then evaluate the effect of pollinators on pollen deposition and fruit development.
Other studies show that asynchrony and sequential flowering in species that share pollinators not only reduces competition for them but also allows their pollinators to remain in the community for longer periods of time (Araujo et al., 1994; Machado and Semir, 2006; Marques and Lemos-Filho, 2008). This is the case for three species of Vriesea Lindl. that share the hummingbird Ramphodon naevius Dumont (1818) as their pollinator and bloom sequentially, thereby keeping the pollinator in the area for longer (Araujo et al., 1994).
Alternatively, to the flowering asynchrony mechanism to avoid competition, the flowering phenology of orchid species with pollination by deception (for food), could be conditioned by the flowering phenology of the species they mimic. For example, some epiphytic orchid species do not have floral rewards to attract pollinators, but they benefit from flowering synchronously with other plants that have similar flowers that produce pollinator rewards (Ackerman, 1983; Ackerman, 1986). Another example is Warczewiczella lipscombiae (Rolfe) Fowlie (Orchidaceae), which flowers synchronously with the terrestrial sympatric Neurocarpum javitense Kunth (Fabaceae), whose flowers produce floral rewards (Ackerman, 1983).
Another factor that has been poorly documented or avoided in phenological studies is phylogeny. According to Wright and Calderon (1995), flowering patterns will be influenced and, in some cases, limited by phylogeny, resulting in a tendency for similar flowering dates in taxonomically related species. This approach has only been explored for vascular epiphytes in the Texier et al. (2018) study where they found similar flowering patterns among genera of Orchidaceae. Johansson had already mentioned this in 1974, stating that there is a similarity in the flowering patterns of species of the same genus, setting them apart from the phenological patterns of other genera.
C. Fruiting and seed dispersal phenology of vascular epiphytes
In vascular epiphytes fructification happens mostly during the dry season, contrasting with trees, shrubs, and herbs that fructify mostly in the rainy season (de Freitas et al., 2013). Primack (1987) mentions how fruit maturation is strongly tied to the dispersal syndrome, so that species with fleshy fruits tend to mature once their dispersers are abundant. In the case of epiphytes, which seeds are mostly dispersed by wind (ca. 80 %, Madison, 1977), they tend to liberate them during the dry season.
1. Factors that determine fruiting and seed dispersal phenology in vascular epiphytes
While none of the articles explicitly discusses the factors that might influence these phenophases, it is known that anemochorous plants in seasonal tropical forests tend to disperse their seeds during the dry season (de Lampe et al., 1992; Morellato and Leitão-Filho, 1996; Cortés-Flores et al., 2019) since this dispersal syndrome is closely linked to wind speed and the surrounding vegetation (Augspurger, 1986). Dispersal during the dry season results more effective given the lack of foliage, facilitating the flow of wind currents and allowing the seeds to be carried over longer distances (García-Franco and Rico-Gray, 1991; Mondragón and Calvo-Irabien, 2006; Valverde and Bernal, 2010; Escobedo-Sarti and Mondragón, 2016). As occurs with flowering, fructification, and seed dispersal are influenced by other phenological phases (Primack, 1987) because, for instance, successful regeneration of a species does not only depend on seed dispersal, but also on seeds being dispersed over favorable areas during periods that allow them to germinate and establish as seedlings (Clark et al., 1999). The establishment and germination of epiphytes is a critical phase in their population dynamics (Benzing, 1981; Mondragón et al., 2015) since water availability is one of the main limiting factors for their germination (Benzing, 1978; Castro-Hernández et al., 1999; Toledo-Aceves and Wolf, 2008). This leads to the notion that the best time for dispersal to ensure seed germination and seedling establishment is during the last stretch of the dry season, or during the rainy season.
D. Suggestions for future phenological studies of epiphytes
A) To develop a standardized methodology to measure phenological events, facilitating the recognition of patterns, and interactions, while also enabling the comparison between populations and taxa (Bencke and Morellato, 2002; Miller-Rushing etal., 2010; Denny et al., 2014); B) To increase the taxonomic representation of other families besides orchids and bromeliads; C) To investigate the proximate and ultimate factors that trigger the phenology of this group; D) To assess the temporal and spatial variation of their phenological patterns.
Besides these initial and baseline suggestions, we also consider the following list as relevant to better understand the phenology of vascular epiphytes.
Tracking vegetative phenology
While most epiphytes are evergreen, there is also an important group of species that are not, including ferns and some orchids (Benzing, 1990; Hoeber et al., 2019). We suggest monitoring the production of leaves, pseudobulbs, and offspring in species where the phenophases can be set apart. This must be done considering that each phase needs to have a well-defined beginning and end date (Denny et al., 2014).
Host effect on epiphyte phenology
It is known that epiphytes have an intimate relationship with their host trees (Einzmann et al., 2015; Wagner et al., 2015; Ticktin et al., 2016; Ramírez-Martínez et al., 2018; Rasmussen and Rasmussen, 2018) so it is fundamental to understand how these hosts affect epiphyte phenology. If we consider a population as the group of individuals growing on the same tree (Overton, 1994), the effect of the host tree could be evaluated in two levels: a) interpopulation variation among populations on different hosts, and b) intrapopulation variation between individuals growing on the same tree. The first can be linked to the identity of the host, since each host offers different morphologies (e.g. stability and bark texture and angle and size ofthe branches), chemical environment (e.g. nutritional quality of the foliar and cortical runoffs, as well as the presence of allelopathic substances), and microclimatic conditions (e.g. light, temperature and humidity in the canopy) (Zimmerman and Olmsted, 1992; Valencia-Díaz et al., 2010; Einzmann et al., 2015; Taylor and Burns, 2016). At the intrapopulation level, variation can be caused by micro-climatic differences along the tree related to light and humidity gradients from the base to the crown (Johansson, 1974; Cervantes et al., 2005; Zotz, 2007), as well as substrate characteristics (e.g. tree barks retain more humidity than thin branches, while older branches that grow more horizontally retain more aerial soil) (Marler, 2018; Rasmussen and Rasmussen, 2018). The way human activities are causing changes in the composition and structure of forests influences the availability of host trees for epiphytes, thereby, directly affecting the fate of the epiphyte populations. Thus, evaluating the close relationship of epiphytes with their hosts is very important for the implementation of management practices and conservation plans (Hsu et al., 2012; Wagner et al., 2015).
Study of phenological variation among epiphytes with different growth forms
Different adaptations have developed among epiphytes allowing them to face the limitations posed by their habitats. In the case of bromeliad epiphytes, we can differentiate two large groups: tank bromeliads (those that accumulate water and debris between their overlapping leaves) and atmospheric bromeliads without tanks, which are densely covered by peltate trichomes (Benzing and Renfrow, 1974; Benzing, 2000). Both groups have quite different ways of capturing water and nutrients (Reyes-García et al., 2008; Cardelús and Mack, 2010; Wu et al., 2018). For example, bromeliad tanks capture and store water and debris between their leaves, providing a regular supply of resources and shelter for an array of organisms, which contribute nutrients from their debris (Benzing, 1990; Romero et al., 2010). On the other hand, atmospheric bromeliads absorb water and nutrients by pulses from atmospheric sources when they are available (Zotz and Hietz, 2001; Reyes-García et al., 2012). These differences not only influence the vertical distribution of these epiphytes on their hosts but might also produce variation in phenological patterns given their growth forms.
Another option for the further development of these studies could be to consider the Cardelús and Mack (2010) study as a reference. They found variation in the nutritional status of orchids, ferns, and bromeliads associated with differences in the way they acquire nutrients. Ferns and orchids have functional radicular systems and might depend more on nutrients coming from solid depositions and the aerial ground, whereas bromeliads would depend on runoffs and atmospheric sources. These differences might prompt phenological variation between these groups given that phenology is affected by the nutritional status of individuals, according to Williams-Linera and Meave (2002).
Effects of herbivory on the phenology of vascular epiphytes
Herbivory as an ultimate factor for epiphyte phenology has hardly been explored at all. Among epiphytes, herbivory is of low occurrence when compared to terrestrial plants (Benzing, 1990; Zotz, 2016) which could indicate that it does not have an important effect on their phenology. However, we need studies to confirm this idea, since there are reports of herbivore damage on reproductive structures of different orchid and bromeliad species, affecting flowers, peduncles, spigots, bracts, and fruits (Ackerman, 1989; Cascante-Marín et al., 2009; Orozco-Ibarrola et al., 2015; Palacios- Mosquera et al., 2019). This indicates that herbivores can have a direct effect on the loss of epiphyte reproductive tissue and an indirect effect on the behavior of pollinators (Canela and Sazima, 2003; McCall and Irwin, 2006; Cascante-Marín et al., 2009). Insects are the main consumers of reproductive structures (florivores) in vascular epiphytes (Canela and Sazima, 2003; Cascante-Marín et al., 2009; Orozco-Ibarrola et al., 2015; Nunes et al., 2016). This could lead one to expect that species affected by these florivores would delay or advance their phenology to avoid matching seasons with higher insect abundance.
In the case of ferns, where reproductive phenology is thought to be limited by proximate rather than ultimate factors (Rathcke and Lacey, 1985; van Schaik et al., 1993; Sharpe and Mehltreter, 2010; Müller et al., 2019), it is relevant to evaluate the effect of herbivory on reproductive phenology, given that their reproductive structures are found on their fronds (Ranker and Haufler, 2008). There is no available information on the effect of herbivory on the foliar phenology of epiphytic ferns, but on terrestrial ferns, with different growth forms, it has been observed that herbivory can determine the phenophase of leaf senescence (Mehltreter and García- Franco, 2008; Mehltreter and Sharpe, 2013).
Effect of climate change on epiphyte phenology
Given the strong correlation between epiphytes and both water and atmospheric nutrient availability, several authors have pointed out that these will be one of the most affected life forms by the ongoing climate change around the world (Lugo and Scatena, 1992; Benzing, 1998; Zotz and Bader, 2009). Climate change has already caused variation in the phenology of different species (Menzel et al., 2006; Mo et al., 2017) so one could expect epiphytes to be similarly affected.
Climate change is also related to current mismatches occurring between pollinators and the flowering of species (Rafferty et al., 2015; Gezon et al., 2016). Most vascular epiphytes have highly specialist pollinators (Madison, 1977; Ackerman, 1986; Zotz, 2016), and evaluating if this mismatch is occurring is important because it could affect the reproductive success and survival of both the epiphytes and their pollinators (Kudo and Ida, 2013).
CONCLUSIONS
The phenology of vascular epiphytes is just beginning to be explored when compared to other life forms. Thus, there is a vast universe of opportunities for research on their phenological patterns. Only by directing efforts to study these patterns will we be able to clearly understand the proximate and ultimate factors that drive them and the potential repercussions of climate change on vascular epiphyte populations.