1. Introduction
The Waste Cooking Oil (WCO) must be properly disposed after three utilizations in order to avoid health problems [1]. However, the current situation is far from suitable. From one side, there is an informal sector in Colombia, which collects the WCO in order to restore its transparency using unappropriated methods, repackage it and later sell it in low-income areas in big cities. This illegality generates a series of negative impacts on public health, due to reusing cooking oil increases the cholesterol, causes cancer, attacks organ cells, produces intestinal problems, among others [2].
Furthermore, the beneficial components of cooking oil known as CIS (beneficial fats for good cholesterol) change to TRANS (fats considered harmful to cardiovascular health), which are hard to digest and eliminate, but also reducing good cholesterol and increasing bad cholesterol [3]. The prefix "cis" indicates that the functional groups are on the same side of the carbon chain; while in the “trans” prefix, the functional groups are on opposing sides of the carbon chain. On the other hand, the WCO is disposed through the sewage system due to the people’s lack of knowledge or poor environmental awareness. Siphoning WCO generates several negative impacts in the environment such as the contamination of water sources and soil; pipe clogging and extra operational expenses in the wastewater treatment plants.
The success of the WCO-to-biodiesel production systems relies on the WCO collection efficiency, the conversion technology. The biomass supply chain models have been widely studied in the literature. [4] addressed the WCO collection system as a Multi-Depot Vehicle Routing Problem with Mixed Closed and Open Inter-Depot Routes and proposed a mixed integer linear programming (MILP) formulation where capacity and duration constraints are considered. The model’s outputs were the vehicle routes where a reduction of 13% on mileage and 11% on fleet hiring cost were achieved compared with the current company solution. [5] designed a robust WCO-for-biodiesel supply chain under WCO supply and price as well as biodiesel demand and price uncertainties. First, a model without uncertainties was proposed for economic (profit), environmental (carbon emission), and social (uncollected WCO) optimization. Second, a robust model was proposed by describing the main uncertainties as several uncertain scenarios. Finally, a genetic algorithm was applied to solve the robust model by dividing the model into several submodels through horizontal decomposition. As the main conclusion, the study remarks both robustness and sustainability as key factors in the biofuel supply chain design stage. The results suggest a decentralized production: loading was reduced by 0.64% - 31.03% and transport by 8.31% - 42.68%. Better social effects could be reached if government policies are implemented allowing environmental and social cooperation from biorefineries.
Following a systems approach, [6] reviewed the operation mechanisms of third party take-back and biodiesel enterprise take-back modes in three countries and compared them using recycling costs and profits of biodiesel enterprises, subsidies for manufacturers, recycling rates, degree of administrative control, technical support and incentive mechanisms provided for the WCO suppliers. The study shows that the WCO-to-biodiesel conversion efficiency depends on the recycling mode that is being practiced, e.g., the third party take-back mode (practiced in Japan and the US) is superior to the biodiesel enterprise take-back mode due to the subsidies provided for biodiesel enterprises and the implementation of strict regulation policies in place for the restaurants.
In the case of Colombia, there is a regulation stimulating biofuels production but there is not any kind of subsidies of regulation for the WCO management [1].
Moreover, [7] compared two WCO collection systems based on the energy efficiency to produce biodiesel: the results showed that it is feasible to collect WCO and waste lubricating oil according to the cost benefit analysis. [8] proposed two different solutions using Mixed Integer Linear Programming (MILP) formulations (PM: Primary Model and SM: Schedule-based Model). Both formulations were compared in a case study and a partial linear relaxation was used showing better optimal results with the first model. The authors obtained a periodic routing schedule to repeat over an infinite horizon to calculate the amount of oil to collect, the total inventories and the collection total cost minimized. In another study, [9] evaluated two WCO collection systems in Portugal: door to door and using street containers. Depending on the collection system, the environmental effects can change. The street containers system environmental effects are less than 5% and the door to door system was close to 50%, the difference in the results with the application of different allocation methods ranged between 1 to 11 % (absolute value). [10] proposed a WCO-to-biodiesel supply chain design on three levels: sources, distribution centers and factories. They used a MILP model with environmental and economic optimization.
The state-of-the-art evidences that the WCO collection strategies varies in each country or study case. The use of MILP is common to measure economic, environmental or social impact. A collecting strategy for WCO in Cali becomes an alternative not only to reduce the social and health issues but also to know the environmental and economic impacts of biodiesel production.
2. Methodological approach
The Biomass supply chain models’ key variables and characteristic were summarized from the state-of-the-art and process through a conceptual model with the aim of identifying the qualitative and quantitative factors [Figure 1]. The models proposed by [8,5] and [10] were selected after the literature review. The three models were analyzed considering their main objective and the conceptual model proposed. This characterization led to select the methodology proposed by [8] with a decentralized strategy where the WCO is collected door to door (in situ). Although it is possible to get this kind of result through the models proposed by [10], they were not selected because their model took into consideration the whole biodiesel supply chain, i.e., the biorefineries, the WCO collection and the biodiesel distribution system, which are beyond the scope of the current paper. In addition, the MILP formulation and model will be adjusted bearing in mind the Supply Points (SPs), WCO frequency, containers’ features, vehicle capacity and another local characteristic in Santiago de Cali.
2.1 Mathematical formulation
The model parametrization was done taking into consideration the local features around the WCO SP and the sustainable development policy proposed for the study. The objective function is to minimize the total cost (TC) of collecting the WCO necessary to satisfy the plant demand as is shown in the following Equation (1).
Subject to: Constraint (12) represents the flow balance at each source node and the constraints [3] and [4] their upper bounds following the vehicle capacity and the waste oil quantity collected on each node. Lower bounds are set by the constraints [5]. [6] and [7] are the incoming and outgoing degree balance constraints to couple the binary X ijt and Y it variables; which are complemented by the depot’s degree balance throughout constraint [8]. Constraints [9] ensure that the collection amount at node i in period t must be zero unless it is visited in that period. Constraints [10]-(15) are used to calculate the inventory at the source nodes and the depot. Inequalities (16) and (17) guarantee that the number of vehicles dispatched is enough to carry the collected WCO. (18) to (24) define the binary variables and the non-negative constraints.
Table 1 and Table 2 summarize the model parameters and variables respectively.
The minimum cost and the vehicle routes obtained from the model will be complemented adding environmental and operational indicators with the aim of analyzing the different impacts generated by the system as well as its efficiency.
2.2 Performance indicators
A set of performance indicators are proposed broadening the model scope. Currently, there is not reliable information about the WCO collection costs, the GHG emissions or potential GHG reductions due to the WCO-based biodiesel use. Table 3 summarizes the proposed performance indicators. Where ∁ is the cost per WCO gal collected, TC is the total cost [COP] and 𝜐 is the WCO collected [WCO gal]. 𝐵 is the biodiesel produced from WCO collected, and 𝜆 is the WCO-to-biodiesel production ratio of 0.8 [biodiesel gal/WCO gal]. γ is the CO2 emissions from WCO collection; dT is the total distance traveled [km]; ω is the vehicle performance [gasoline gal/km] and D is the CO2 emissions factor [kg CO2/gasoline gal]. 𝛽 is the potencial reduction of CO2; 𝛿 is the biodiesel produced from WCO [gal]; 𝜌 is the CO2 emissions factor [kg CO2/diesel gallon] and 𝜇 is the CO2 emissions factor [kg CO2/biodiesel gal].
3. Results and discussion
A pilot plant located in Cali was taken as a case study in order to validate the model. The plant has an installed and a fleet capacity of 1100 WCO gal/d (r t ) and 50 gal (Q) respectively. The current system has 12 supply points (I) with an average WCO production of 0,83 gal/d (a it ). The WCO collection will be twice per week (T).
3.1 Current system
The model was run with the 64-bit version of the mathematical modeling and optimization suite GAMS 23.6.5, conducted on a server equipped with Intel Core i5 2.40 GHz y 3 GB RAM. The optimization did not reach any feasible solution as the requirements were higher than the WCO available in the system (33.58 gal). Even though a feasible solution could be obtained if the plant purchases virgin oil; this is against the model’s sustainable development policy. Then, the plant demand was adjusted to the current WCO availability in the system with the aim of validating the model and obtains an optimal. The model results and performance indicators are summarized in Table 4 and Table 5 respectively.
Table 5 Performance indicators for the case study with modified requirements
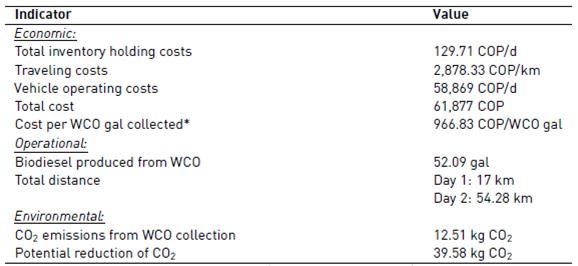
* Crude palm oil in Colombia: 1,968 COP (second semester 2017)
The model’s results scheduled the vehicle routes for the two days programmed. An optimal total cost and WCO collection of 61.877 COP and 966.83 COP/WCO gal was calculated. The environmental indicators allow knowing the carbon footprint generated by the WCO collection, and the potential CO2 reduction if the WCO-based biodiesel is used to replace the same amount of diesel in the market.
3.2 SPs densification (improved scenario)
A different approach to attack the problem is increasing the WCO availability using a SPs densification strategy. The goal is to have a greater WCO collection in order to profit from the plant’s installed capacity. The SPs densification was done adding WCO sources following the existing point’s profiles, i.e., adding others restaurants, casinos, universities within the supply radius. The results obtained with this strategy are shown in Table 6 and Table 7.
The strategy evidences an important effect in the amount of WCO collected and the biodiesel produced. It is possible to satisfy the plant installed capacity if the extra SPs are considered, despite the higher total costs; the cost per WCO gal collected is reduced by 28%. The environmental indicators showed a significant improvement with CO2 reductions five time better than the initial scenario.
Table 8 shows a comparison between the analyzed scenarios (current and improved) together with the pilot plant installed capacity (ideal scenario). Each scenario offers enhanced results, but social strategies of knowledge appropriation must be developed to include the community and changes in WCO collection practices.
4. Conclusions
The study of WCO-to-biodiesel supply chain models has been widely covered by the literature. Nevertheless, there are not local studies with information about the WCO costs or other logistics indicators. A MILP model was proposed to calculate the minimum collection cost and set the vehicles routes. A logistic and environmental assessment was presented to evaluate the feasibility of WCO-based biodiesel production in Cali considering qualitative and quantitative factors. The sustainable WCO-based biodiesel production is possible in Cali if social strategies targeting changes in WCO disposal practices are implemented with the goal of increasing the collection system’s efficiency through the SPs densification.
Index sets
I = 0, 1, . . . , n : the set of n source nodes and the depot 0.
IC = 1, . . . , n : the set of n source nodes only (a subset of I ).
T = 1, . . . , τ : the set of τ periods in the cyclic planning horizon.