1. Introduction
The construction sector represents 26.1% of the final national energy consumption for energy uses, according to the Energy Saving and Efficiency Action Plan in Spain 2011-2020[1]. For this reason, it is a strategic sector provided with important public and private resources, as there is still a high potential for energy savings. In the year 2020, that Plan in the building equipment sector establishes a goal of final energy saving of 33.3 TWh/year, which represents 16.1% of the total savings planned for that year.
The real estate park is currently responsible for 36% of all CO2 emissions from the European Union. The Union has committed to establishing a system of decarbonised energy in the year 2050, for which there are various standards aimed at improving the energy efficiency of the building park, such as Directive 2010/31 / EU[2], on the energy performance of buildings, Directive 2012/27 / EU[3] on energy efficiency or the Directive 2018/844[4] which modifies the previous ones. Likewise, the Paris Agreement of 2015 boosts efforts to decarbonise the real estate park.
The Consideration 17 of Directive 2010/31 / EU states that "Measures are needed to increase the number of buildings which not only fulfil current minimum energy performance requirements, but are also more energy efficient, thereby reducing both energy consumption and carbon dioxide emissions". In Article 9 of the same, it is established that the Member States will ensure that before 12/31/2020 all new buildings are almost zero-energy consumption buildings, and that after 12/31/2018, new buildings that are occupied and owned by public authorities are buildings of almost zero-energy consumption. In addition to the above, Article 5 of Directive 2012/27 / EU establishes the exemplary function of the buildings of public organisms obliging the member states to renew annually 3% of the surface of the buildings occupying the Central Administration.
Because of previously cited directives, the technical knowledge detailing the possibilities of saving energy and incorporating renewable energies in buildings are considered very relevant.
At present, glass façades are also widely used for their aesthetics and adequate use of natural light, although they have low thermal and acoustic insulation. These drawbacks are significantly improved with double skin façades.
This research focuses on analysing the operation of the double-skin façades, glass-glass, used to harness solar energy for heating buildings. The South facing glazed façades (in the northern hemisphere) are a constructive solution that has been historically employed in buildings, by incorporating glazed balconies or attached greenhouses to use solar radiation for air conditioning in winter.
This research has analysed the energy savings using mathematical formulations and computer calculations automated to apply the mathematical model hour by hour throughout the year. At a later stage, measurements are made on a real building during the 2018-2019 heating season to verify the results.
As a starting point, a search of related publications has been carried out using solar energy through glass chambers. The most significant part of the studies carried out to date has been based on computer simulations and/or mathematical methods, identifying only a few based-on experiments or monitoring of real buildings.
After carrying out the meta-analysing data from 27 publications related to the energy efficiency of double-skin façades, an average potential reduction is determined from the heating load of 33%. One of the conclusions of this study is the dispersion of the energy saving produced, which varies from 90% of savings to an increase in consumption of 30%. According to the same report, this is motivated, by the variability of constructive solutions, the ventilation method of the chamber, the climate zone, and the methodology with which the study is carried out. In this regard, the study highlights the significant difference in the amount of energy saving determined by mathematical methods up to 90%, simulation up to 53%, and experimental as only 30%[5].
A review of publications on double-skin façades also indicates that the energy-saving results obtained with this constructive solution are not consistent since they vary from negative saving to 50%[6].
An investigation comparatively simulated the behavior of a building with a double-skin façade in two locations with very different climates: Rio de Janeiro and London. The best energy saving results obtained in London are 85% and 61% in Rio de Janeiro[7].
Additionally, a publication related to the simulation results of double-skin fronts in an office building in Tehran, with hot and dry weather, concludes that the energy savings of this constructive solution varies between 7.9% and 14.8%[8].
Regarding the publications based on measurements in real dip, an investigation indicates that double-skin façades can reduce the consumption of building energy in summer[9].
Considering the experimentation using a test cell or similar, an investigation indicates that it is important to control the air temperature supplied by the façade in winter, since at least 50% of the time the preheating is negative[10]. Besides, another investigation indicates that double-skin façades built with opaque materials can reduce building energy consumption in regions with hot climates such as Brazil[11].
Finally, given the high energy-saving potential of this construction solution, it is expected to be a highly relevant measure to achieve the objectives of buildings with almost zero consumption established by European Directives, and contribute to the construction of more efficient buildings in other regions of the world such as the Andean Region.
2. Experimentation
The procedure summarized below is based on the determination of the energy flows in the double- skin façade to quantify what part of the incident solar energy can be used for heating the building. By taking advantage of this energy flow, the heating system will have a lower producing consumption, therefore an energy saving. A predictable increase has not been determined in energy consumption for cooling that the same double-skin façade would produce in the summer season, since there are multiple solutions with different efficiencies to produce shade on the façade, and thus, reduce these hot gains.
Figure 1 shows the basic scheme of the double-skin façade to consider, also indicating the main energy flows.
The circulation of air inside the chamber generated between the two glasses is carried out by natural convection, without any type of mechanical ventilation system. The entrance of air from the chamber to the interior of the building has an automatic gate that only opens during the period of heating, providing that the average temperature of the air in the chamber exceeds the interior temperature of the building by a number of preset degrees.
Energy savings for heating are determined by comparing the energy demand of a conventional construction building, with the energy demand of the same building with a double skin façade in one of its enclosures. In order to obtain a valid procedure for the largest number of cases, buildings with different energy demands for heating will be taken into account, and façades with any angle of inclination and azimuth with respect to the south will be considered.
2.1 Study of solar radiation
The starting data are direct solar irradiation and diffuse solar irradiation on a horizontal surface. First of all, it has been determined, from these, the global irradiance received by the exterior glass, which is found with a certain inclination (b) and azimuth (a).
Direct solar irradiation on the exterior glass (PRVDirect) is obtained with Equation (1):
In which:
PRHDirect = Direct solar irradiation on a horizontal surface (W/m²)
k is a factor that is determined with Equation (2)
In which:
θ = Latitude (rad)
α = angle of orientation from the normal to the façade, taking as its origin the south orientation and considering negative angles towards the east and positive towards the west. (rad)
β = slope of the façade (rad)
ω = schedule angle (rad)
δ = solar decline (rad). It can be determined for each hour of the year with Equation (3)
In which x, can be obtained with Equation (4)
Where N and h are the number of the day of the year and time of day, respectively.
The diffused solar irradiance on the exterior glass (PRVDiffuse) is obtained with Equation (5).
The solar radiation reflected on the exterior glass (PRVReflected) is obtained with Equation (6).
The global irradiance on the outer glass (PRG) is the sum of the direct, diffuse, and reflected solar radiation as shown in Equation (7)
Known as absorptivity, transmissivity, and reflectance of the two glass panels, each of the energy flows listed in Figure 2 can be determined. By grouping the energy flows and multiplying the glass surface, the solar net that reaches the interior, the solar irradiance absorbed by each of the glasses, and the irradiance reflected to the outside can be obtained.
2.2 Transmission of heat on the surface of the glasses
When a glass is at a different temperature than its surrounding environment, it will exchange heat by convection and radiation. The heat that is exchanged with the air is transmitted mainly by convection and with the rest of the elements, by radiation.
The convective heat transfer to the air surrounding the glass can be determined with Equation (8).
Where,
Q air = Heat flow transmitted by convection to the air in contact with a vertical surface (W)
S = surface in contact (m2)
h = Coefficient of convection (W/m2 oC). In this case, as it is a flat surface placed in a vertical position or close to it, this coefficient can be obtained with Equation (9).
Tv = Glass temperature (oC)
T a = Air temperature (oC)
The radiation heat exchange of glass can be determined with Equation (10).
In which,
Q rad = Radiant heat flow emitted by the glass surface (W)
( = emissivity of the glass surface (dimensionless)
( = Stefan-Boltzmann constant = 5.669x10-8 (W/m2K4)
S = surface (m2)
T v = Glass temperature (oC)
T a = Air temperature (oC). It is assumed that all elements that exchange heat by radiation with glass are at this same temperature.
The radiation emitted on each side that has contact with the chamber, both the exterior and interior, has not been evaluated assuming that both are at a very close temperature, and therefore, the heat transmitted by radiation between both is negligible.
Applying the previous Equations 8, 9 and 10 and assumptions, it is possible to obtain:
The heat flow transmitted by convection and radiation inside the building can be calculated with Equation (11).
The heat flow transmitted by convection and radiation to the outside can be obtained with Equation (12).
The heat flow is transmitted by convection to the air inside the chamber can be determined with Equation (13). The rest of the energy absorbed by the exterior glass, which is not transmitted to the exterior, and the rest of the energy absorbed by the interior glass, which is not transmitted to the interior of the building, will be transmitted to the air in the chamber by convection.
2.3 Determination of energy savings
The energy saving for heating is produced by the introduction into the building of three energy flows:
Net solar radiation energy that reaches the interior.
Energy transmitted by convection and radiation from the interior glass to the interior.
Energy transmitted in the form of hot air from the air chamber.
Not all available energy in these flows can be considered useful or usable energy for the heating system. These conditions must be met, so that these flows are considered useful energy:
There is a demand for heating. Only the hours when the outside temperature is below a certain preset value have been considered.
The instantaneous heat provided by the double-skin façade is the same or lower than the heating demand at that moment. In the event that the heat provided by the double-skin façade is superior to the demand, it is considered useful energy for an appropriate time.
The sum of the useful energy flows during a year will be the energy saved annually. The percentage of energy saving is determined with Equation (14)
The procedure used to calculate the heating demand of the building without the double-skin façade is explained in the following section.
2.4. Automation of calculations
The procedure described in the previous section has been programmed in an Excel spreadsheet to perform calculations automatically, for each hour of the year. It is also useful to assess energy savings easily, and assess how modifying the starting data affects it.
The main starting data are:
The hourly data of external dry temperature
Specific humidity of the air
Direct solar irradiance on a horizontal Surface
Diffused solar irradiance on a horizontal Surface
This data has been taken from the climate files, published as documents known to be used in the energy certification of buildings, by the Ministry for the Ecological Transition of the Government of Spain[12]. On the website of this Ministry, free data from the provisional capitals of Spain are available.
In order to evaluate the influence of climatology on energy saving, we have selected ten cities in Spain with decreasing latitudes. In addition, these cities are representative of different climates that occur in the country. Table 1 shows the chosen cities and their latitude, longitude, and altitude. In the same table, the climatic zone corresponding to each city is included, in accordance with the Code building technician (CTE)[13], and the referred heating demand considered for each city as established in Annex III.2 from the recognized document for the energy certification of buildings "Qualification of the energy efficiency of the buildings "[14]. The rest of the starting data are shown in Table 2.
To establish the total heated area of the building, the heated surface ratio is used between an available double-skin façade surface (RT1). Multiplying the available double-skin façade surface by this index, the total heated area of the building is obtained. By varying this relationship, energy savings can be estimated in buildings with different geometric configurations.
The hourly heating demands of the building without the double-skin façade have proportionally determined the thermal jump between inside and outside, from the annual heating consumption, which is obtained through the consumption ratio of final energy for heating (RT2) in kWh/m2 a year. This ratio is often used in the energy certification of buildings, being easy to have it in buildings having this certificate.
3. Results and discussion
Based on the procedure established and using the described simulator as a tool, tests have been carried out to determine the influence of energy-saving variations from each of the starting data. After multiple tests with this model, it has been concluded that the variables that have the most significant influence on the magnitude of savings are:
Location-climatology. Directly related to the latitude and average annual temperature of the building location
Azimuth of the façade with respect to the south orientation
Relation surface of double-skin façade / heated surface (RT1)
Reflectance of the glasses
3.1 Location-climatology
Climatology is one of the factors that has a greater influence on the amount of energy saving for heating. Specifically, the two climatological variables that most influence are the outside temperature, as it is directly related to the heating demand, and the solar irradiation, since it is the source of energy from which the savings are extracted.
As explained above, the influence of location and climate on energy saving has been assessed through geographic and climatic data from 10 cities in Spain. Figure 3 shows the evolution of the useful energy produced by the double-skin façade, and Figure 4, the evolution of energy saving.
In absolute terms, the annual useful energy provided by the double-skin façade is greater in cities with a lower average outdoor temperature. Specifically, of the cities analysed, León has the highest useful energy and Cádiz the lowest. Both cities correspond to the lowest and highest annual average temperature, respectively. As shown in Figure 5, the annual useful energy can be obtained from the annual average temperature by means of the equation of a line, with a correlation of 0.92.
The percentage of energy saved is extremely variable, and there has not been identified any variable on which it depends predominantly. If it has been detected that it increases when the average solar radiation increases, the correlation is low (0.3). This fact justifies the need for a procedure with certain complexity for its determination as presented in this communication.
Since climatology is a determining factor for energy saving, the analysis carried out in the following sections for the rest of the variables is carried out individually for each of the ten cities.
3.2 Azimuth of the façade with respect to the south orientation
For each of the ten cities, the useful energy produced and the energy saving for different azimuths of the double-skin façade have been determined. Figure 6 shows the variation of energy savings with different azimuths.
With the analysis of these data, it is deduced that there is a very high relation between the energy saving and the azimuth of the façade. On the other hand, contrary to what one might think at first sight, the pure southern orientation is not the one that produces the greatest energy savings in general. As can be seen in Figure 6, the maximum saving values depend on the city (climatology) and are deviated a few degrees towards the east with respect to the pure south orientation. The azimuth with which the maximum saving is obtained deviates more to the east, the higher the annual average temperature. This is due to the fact that the solar contribution is more usable for heating in the morning than in the afternoon, because the building has a greater demand for heating in the morning than in the afternoon, due to the thermal jump between the inside and outside in the mornings.
Figure 7 shows, the azimuth with which the maximum energy saving is obtained based on the average annual outdoor temperature for each city. This azimuth deviates more to the east (more negative), the higher the annual average outside temperature. This relationship can be represented by the equation of a line with a correlation of 0.71.
3.3 Relationship between double-skin façade surface and heated surface
The smaller the relation between the double skin façade surface and the heated surface of the building, that is, the smaller RT1, the energy saving in heating is greater, although it does not present a direct proportionality as can be seen below.
As shown in Figure 8, to obtain savings of percentages higher than 20% in any of the cities studied, RT1 must be less than seven. With RT1 values higher than 14, the energy saving is, in all cities, less than 15%, so the contribution of the double-skin façade may start to be of little relevance from an economic point of view.
In Figure 9, it can be seen that in cities with colder climates, the useful energy increases rapidly with increasing RT1 until it reaches a value equal to 4. From this point, the useful energy continues to increase but with a lower slope. In cities with warmer climates, the curve of increase in useful energy has a more flattened shape.
3.4 Reflectance of the glasses
This variable also greatly influences the energy saving of double-skin façades, since the greater the amount of solar radiation reflected, the less solar energy is available to be introduced into the building. As can be seen in Figure 10, energy savings decrease with increasing reflectance. The decrease is linear, and the slope of the straight line is practically the same in all cities, so we can deduce that it is independent of the weather.
The reflectance of the glass should be taken into account in the design of double-skin façades, since, as can be seen in Figure 10, with reflectance above 30% the energy saving is less than 15% in all cases, so the contribution of the double-skin façade can start to be of little relevance from an economic point of view.
In Figure 11, the evolution of useful anaerobic energy as a function of the reflectance of the glasses is shown. It is appreciated that the useful energy varies according to a straight line, which presents a lower slope, the higher the annual average temperature of the city considered.
3.5 Future works
In the winter season of 2018-2019, it is the intention to validate the procedure described above by measuring the energy savings generated in the headquarters of the Regional Energy Agency of Castilla y León (EREN), located in the city of León, and which provides of a double-skin glass-glass façade with a configuration similar to that presented and a glazed surface in the south orientation of 1,032 m2. In this building, probes have already been installed for the measurement of temperature and relative humidity outside, the interior temperature of the chamber at different altitudes, incidental solar radiation on the façade, ventilation airflow of the chamber, etc., as well as a switchboard that will allow the continuous recording of the variables measured by the probes.
The first tests of the measurement system already show interesting data. As an example, the following figures show measurements of the day 09/14/2018. Figure 12 shows the evolution of air temperatures inside the camera and the solar radiation. In Figure 13, the thermal jump (air outlet temperature of the chamber minus inlet temperature) is shown as a function of solar radiation and airflow as a function of solar radiation. In both cases, the belt is very high, so it is considered that the measurement system installed is adequate. These equations will allow to determine the power generated in the form of hot air in the chamber (function of the flow and the thermal jump) in function of the incident solar radiation.
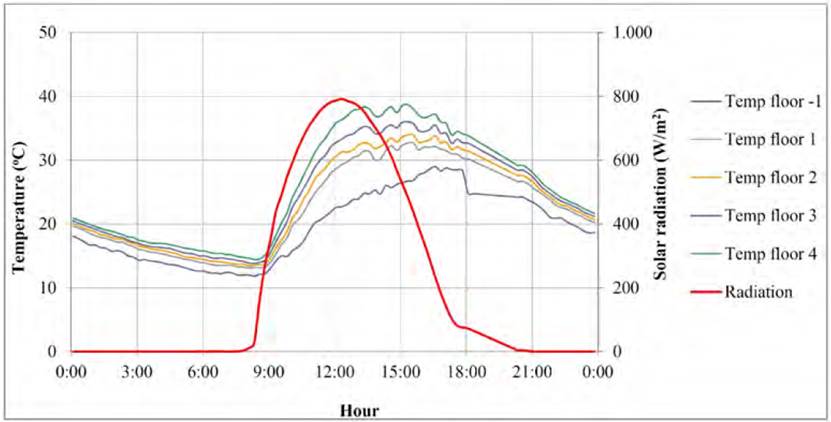
Figure 12 Evolution of air temperatures inside the chamber and incident solar radiation (09/14/2018)
4. Conclusions
The energy savings provided by the double-skin façades studied are highly variable and depend mainly on the location, the climate, the azimuth of the façade, the relationship between the surface of the double-skin façade and the heated surface, and the reflectance of the glass.
Not all the energy that this construction solution can produce is usable for heating. There are time intervals in which the façade's heat production exceeds the heating demand, so not all the energy produced can be used. This fact indicates that the percentages of energy savings could be improved if the system is equipped with elements that store thermal energy.
In absolute terms, the annual useful energy provided by the double skin façade is higher in cities with a lower average annual outdoor temperature. For the ten cities studied, a very high correlation has been found between the average annual temperature and the energy savings provided.
The pure south orientation is not the one that produces greater energy savings in general. The optimal orientation depends on the climatology, and it deviates some degrees towards the east with a purely south orientation. This is due to the fact that the solar contribution is more usable in the mornings than in the afternoons, as the building presents a greater demand for heating in the morning since the thermal jump between the interior and the exterior is greater. The optimal azimuth deviates further east, the higher the annual mean outdoor temperature. This relationship can be represented by the equation of a line with a good correlation.
To obtain savings percentages greater than 20% in climatologies similar to those studied, the ratio between the double-skin façade surface and the total heated surface of the building (RT1) must be less than 7.
The reflectance of the glass must be taken into account when designing this constructive solution since, with reflectances greater than 30%, energy savings are less than 15% in any weather.
Finally, additional work is being developed for the validation of the procedure described in this document with measurements in a real building. After the analysis of the first measurements, the equations that allow determining the thermal jump of the air in the chamber as a function of the solar radiation incident on the double skin façade have been found.