1. Introduction
The interest in chemical species at the nanoscale is one of the most important scientific and biomedical research fields, mainly due to various new properties and potential applications that can be developed in different areas. At this scale, there are metallic nanoparticles, which present interesting biological, optical, magnetic, electronic, and catalytic properties[1].
Due to the valuable antimicrobial condition of silver nanoparticles (AgNPs), they are significant to the medical and chemical industries. Some studies show that silver nanoparticles have a very strong bactericidal effect in sizes between 1 and 10 nm. There are different ways to synthesize AgNPs using physical and chemical methods, but recent research has focused on alternative methods such as biosynthesis or green synthesis, due to a growing need for environmentally friendly synthesis methods using reducing and finishing agents[2].
There are numerous plant extracts used in the synthesis of AgNPs, such as Aloe vera (aloe vera), Azadirachta indica (Indian lilac), Camellia sinensis (tea plant), Carica papaya (papaya), Capsicum annum (chili bell pepper), Coriandrum sativeem (coriander), natural rubber, Tamarindus indica (tamarind), Musa paradisiaca (banana), which have been successfully tested for the formation of silver nanoparticles[1]. This makes Musa paradisiaca attractive to be implemented in this study, because Colombia is one of the major players in the international banana trade, occupying the fourth place with an equivalent of 9% of the total volume of banana exports worldwide[3]. Furthermore, it is considered that banana peel can be a potential source of antioxidant and antimicrobial substances, as well as phytochemical compounds with activity against free radicals. This is why it is necessary to take advantage of these residues, being banana peels a classic example of natural material available in abundance, are usually discarded[4].
Previous studies demonstrate the effectiveness of Musa paradisiaca peel in the synthesis of gold nanoparticles, being this extract simple, non-toxic, and environmentally friendly. These nanoparticles showed sizes of 300 nm and efficient antimicrobial activity towards most of the tested fungal and bacterial cultures[4].
The composition and concentration of the nanoparticles are determined by Ultraviolet-visible spectroscopy, X-ray diffraction, chromatography, and some other techniques[5]. Its importance lies in the fact that the properties of metallic nanoparticles depend on their size and shape. Therefore, it is necessary to monitor their synthesis, which will be reflected in the characterization[6].
To prepare silver nanoparticles, chemical reduction methods are the most used. Generally, the synthesis of nanoparticles in colloidal suspensions by chemical methods requires the following components: metallic precursors, reducing, and stabilizing agents. The metal precursor is composed of silver salt, from which raw materials can be obtained, and then the nanoparticles are formed, as shown in Figure 1. From the dissociation of the salt in the solvent, ionic silver can be obtained. The next step is to reduce the silver ion to a state of zero valency, which is achieved in the presence of a reducing agent, which can basically yield electrons[7]. This can be expressed by the following chemical Equation (1):
Where:
Red: Form of the unreacted reducing agent.
Re+: Reducing agent form in oxidation state.
Finally, the neutral silver atoms aggregate and form groups. The objective in this phase is that the size of these complexes is a maximum of 100 nm, the limit size to be considered as nanoparticles; in this phasee, a stabilizing agent is used to prevent agglomerations beyond this size[7].
In this work, the synthesis of AgNPs was developed implementing green chemistry, which is based on reduction reactions of metallic silver salts, using a natural solvent as a reducing agent. In order to evidence the composition and concentration of the synthesized nanoparticles, UV-Visible and XRD characterization techniques were implemented. Likewise, to determine the influence of the extract on the formation of AgNPs, the technique of gas chromatography coupled to a mass spectrophotometer was used.
2. Methodology
2.1 Synthesis of silver nanoparticles
Twenty-six Musa Paradisiaca shells (dry weight 1580 g) were collected in order to acquire a natural extract that favors the process of silver nitrate reduction and thus synthesizes the nanoparticles. The pre-treatment for the banana peels consisted of cutting and washing them thoroughly with distilled water at 90 °C for 30 min. Subsequently, the selected (1580 g) banana peels were crushed with mortar and pestle and dissolved in 1600 ml of distilled water, following the 1:1 ratio. Finally, the resulting extract was filtered through a clean cloth and stored in glass containers previously washed with distilled water.
The synthesis of silver nanoparticles was carried out by reducing a precursor salt, silver nitrate (AgNO3), using the previously prepared banana peel extract as a reducing agent, in order to avoid the implementation of organic solvents that produce undesirable products as pollutants.
To carry out this synthesis, a 100 mM silver nitrate stock solution was originally prepared by weighing 1.6614 g of silver nitrate and diluting it in 100 ml of distilled water. In due course, 25 ml of the stock solution were extracted and placed in a 250 ml balloon filled with distilled water (approximately 225 ml), resulting in a 10 mM silver nitrate solution; this procedure was performed in triplicate.
The procedure of the synthesis of the AgNPs consisted of mixing the extract (533 ml) of the banana peels with 250 ml solution of silver nitrate 10 mM; this procedure was performed in triplicate. This reaction was initially performed at room temperature for 10 minutes; therefore, the temperature of the solution was increased to 65 °C for 50 minutes, and the solution was constantly stirred at 500 rpm.
The synthesized nanoparticles were suspended by adding 2M sodium hydroxide until they reached a pH of 10, so that they have good colloidal stability. Finally, the nanoparticles were separated from the reaction medium by centrifugation at 3500 rpm for 10 min. This nanoparticle residue obtained was first washed with ethanol and then with distilled water; in each wash, it was centrifuged at 3500 rpm for 10 minutes. The obtained washed residue was deposited in an oven at 100 °C for 6 hours, to evaporate any presence of water and ethanol, resulting in a thin layer of nanoparticles, as can be seen in Figure 2.
2.2. Characterization of silver nanoparticles
UV-VIS spectroscopy
In order to determine if the silver nanoparticles were formed, a UV-Visible spectroscopy was used with a BK-UV1900 model equipment. In this technique, the samples were prepared by diluting a drop of each solution of extract and silver nanoparticles in different test tubes with 2 ml of distilled water, then the new solutions were shaken in a vortex until obtaining optically transparent solutions, thus concluding the test.
In the case of the analyzed sample of silver nanoparticles, special care was taken because if the nanoparticles were not in suspension, the spectrometer would not read the peak. Once the samples were prepared, a sweep was carried out in a 300-500 nm range, and the absorbance peak was found for each sample.
X-ray diffraction
The diffractometer used for the characterization of the silver nanoparticle sample was an XPert PANalytical Empyrean Series II - Alpha1, Model 2012 branded Malvern Panalytical. To perform the analysis, 2 g of silver powder nanoparticles were placed in the sample holder of the equipment, under the following established conditions of 40 kV and 40 mA, a step size of 0.05 º and a step time of 50 sec. Therefore, a scanning angular speed of 0.06º/min was used. The scanning angle 2θ ranged from 5 to 60º.
Gas-mass chromatography
The composition of the phytochemicals present in Musa Paradisiaca extract was obtained by direct injection of 1 μl of Musa Paradisiaca extract in the mobile phase, using an Agilent Gas Chromatograph with Selective Mass Detector (GC-MS), and with the model 6890N. The selective mass detector is Agilent's brand, model 5973N, and a column DB-1MSInyector: Split/Splitless.
The column was located inside an oven with a temperature program; in the programming of the oven temperature, a starting temperature of 45 °C was used and held for 15 min, then the temperature was increased to 60 °C at a rate of 5 °C/min, and held for 10 min; finally, the temperature was increased at a rate of 2 °C/min to 70 °C and held for 13 min for a total of 38 min run.
3. Results and discussion
3.1. Synthesis of silver nanoparticles
After obtaining the natural solvent from banana peels (Musa paradisiaca), it was used to produce the silver nanoparticles with 10mM concentration. During the synthesis of the nanoparticles, a color change from transparent to yellow-brown was observed, being effective in the formation of silver nanoparticles[8]. This synthesis was influenced by the temperature and reaction time, for which the values of 65°C and 50 min were chosen, based on studies carried out by other researchers[2], showing the effect of the reaction temperature for a range of 30 to 100 °C, and obtaining the best results for temperatures above 60°C, since when this increases, the reagents are consumed quickly, leading to the formation of smaller nanoparticles. With respect to the reaction time, this study evidenced that the intensity of the brown color was directly proportional to the incubation time of the reaction mixture and the constant stirring that guarantees the homogeneity of the solution, so that the reduction of silver ions is completed around 50 min.
3.2. Phytochemical components in the extract of musa paradisiaca
A gas chromatograph connected to a mass spectrometer (GC/MS) was used to determine the phytochemical components of the Musa paradisiaca extract. Some of the compounds that promote the reduction of metal salts during synthesis can be identified by this type of analysis. The chromatogram obtained from this measurement is shown in Figure 3, and Table 1 shows a list of the main components detected.
The chromatographic analysis showed mainly the presence of 1.2 Ethanediol (60.0261%) and 2.3 Butanediol (11.2%), which promote the reduction of silver ions, favoring the formation of nanoparticles[9]. These diols act as a reducing agent for metals, since they behave as a solvent for the metallic precursor and show a higher dielectric constant, which makes them better solvents, presenting a high boiling point[9]. The properties of these compounds allow metallic materials to be transformed into their basic state of electronegativity, which is equivalent to nanoscale and makes free ions attract each other and form nanoparticles. On the other hand, the acetic acid present in 1.7376% favors the stabilization of the AgNPs by acting as a primary surfactant during the nucleation stage of this nanomaterial[10]. This organic acid is essentially found in fruits, and given its structure, it can be coupled to metallic nanoparticles through carboxylic bonds (COO-) and limit the growth of the material's size.
On the other hand, Table 1 also shows compounds that have active organic functional groups (-OH, -NH2, -CHO) of phytochemicals (amino acids, proteins, flavonoids and terpenoids) that were oxidized by reducing the Ag+ ion to AgNPs. AgNPs surfaces were protected by the phytochemicals, making them promising candidates for various applications. Therefore, phytochemicals played a double role as reducing and stabilizing agents. The stretching vibrations of various organic groups (-OH, -NH2, -CHO, and phenolic) of phytochemicals disappeared or changed due to the oxidation of these groups by the Ag + ion[11].
3.3. Characterization of silver nanoparticles
UV-visible spectroscopy
Figure 4 shows the results obtained on the prepared sample of 10 mM at a pH of 10. Soriano et al. determined that the formation of silver nanoparticles is attributed to the appearance of a surface plasmon resonance peak, when these were in a wavelength range of 400 to 450 nm[12]. Therefore, in this image, the appearance of silver nanoparticles is confirmed since a maximum absorption peak was detected when it reached a wavelength of 411 nm.
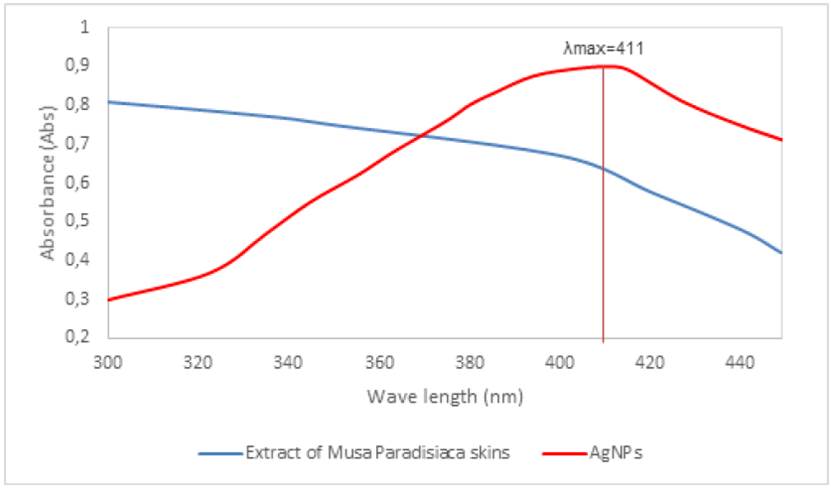
Figure 4 UV-Vis spectroscopy of aqueous suspensions of silver nanoparticles at 10 mM, pH 10 and extract of Musa paradisiaca
In the spectrum, a single band with a maximum absorption peak can be observed, and the formation of silver nanoparticles with sizes between 14 and 35 nm can be estimated. This is stated based on the spectral characteristics of silver nanoparticles reported in Table 2.
The maximum wavelength of absorption in the plasmon of a given solvent can be used to indicate particle size. This study shows silver nanoparticles that produced a wavelength spectrum equal to 400 nm, which is corroborated using transmission electron microscopy (TEM) and size distribution, which showed that the particles become larger as the plasmon peak shifts to longer wavelengths and broadens[13].
X-ray Diffraction (XRD)
In order to determine the crystalline phase corresponding to silver, XRD analyses for silver nanoparticles were performed according to its characteristic face-centered cubic structure[14]. Figure 5 describes the diffraction pattern that indicates the crystalline nature of the silver nanoparticles synthesized by Musa paradisiaca aqueous extract, which corresponds to the face-centered cubic (fcc). The diffractogram shows three different diffraction peaks observed in the 20 range of 30-70° (with values for 20 = 38, 45, 65). This is associated with planes (1 1 1), (2 0 0) and (2 2 0)[15], reflections of the face-centered cubic phase of the metallic silver, consistent with the XRD patterns shown in previous reports on microstructures.
According to the diffractogram, it can be observed that the diffraction peak located in the angular position at 38º presented a higher intensity compared to the peaks at 45º and 65º, indicating that the silver nanoparticles present a greater arrangement of their atoms in 111 planes, and based on what was reported by Pal and collaborators[12], a major reactivity of the silver nanoparticles is expected, since it is favored by planes with a high density of atoms such as the 111 plane.
Particle size calculation
The average crystal size of each sample was determined using the Debye-Scherrer equation, assuming that the crystal lattice is free of deformation and the crystals generally predominate in the sample, by the following equation (Equation (2)][17]:
Where: d is the average crystallite size, k is the shape factor of the crystal, and its value is 0.9, λ is the wavelength of the radiation used (λCu),
is the position of the diffraction peak, and FWHM is the Full Width at Half Maximum of the sample's diffraction peak.
Using the OriginPro 2020b program, the width at the mean height of the highest intensity peak was determined by multiple peak adjustments and obtained in Table 3, from which the mean particle size for the silver nanoparticles was determined.
The value of the FWHM in radians, together with the wavelength of the X-ray emission for Cu-Kα with a value of 1.54178x10-10 m, allows obtaining, as a result, the average particle size calculated for the peak of diffraction with the highest intensity, giving a value of 21.8 nm, by means of the Debye-Scherrer equation.
This result confirms the above; according to the data obtained by UV-Visible spectroscopy, it was inferred using Table 2 that the particle size for an absorbance peak at 411 nm should be between 14 and 35 nm.
From the XRD spectrum, studies have been developed that show the nature of silver nanoparticles. The XRD pattern showed four intense peaks ranging from 20° to 80°, with diffraction peaks in the following values: 38.45, 44.48, 64.69, and 77.62 correspond to the planes for silver (111), (200), (220), and (311), respectively, which illustrates the complete reduction of AgNO3 by the use of an aqueous extract of C. Vulgaris. The Debye-Scherrer formula was used to evaluate the mean particle size, giving a value of 18.94 nm, because, in the XRD pattern, the maximum amplitude is proportional to the mean crystal size of the material. These results were compared by means of TEM analysis, which gave an average value of approximately 20 nm[18].
Other researchers performed X-ray diffraction (XRD) analysis of a sample of silver nanoparticles synthesized using green chemistry[19]. In this study, the XRD pattern was analyzed to determine the maximum intensity, position, and mean height width (FWHM). The FWHM was used in the Debye-Scherrer formula to find the mean particle size, resulting in a size of 16.6 nm. Bragg reflections were also observed corresponding to the set of planes of the network related to the face-centered cubic crystalline structure (fcc). The average size of the silver nanoparticles given in the TEM analysis is approximately 16 nm and with spherical morphology. The above is consistent with the particle size found in the present investigation, and it can also be noted that for these studies, the error between the size found by TEM and the Debye-Scherrer equation does not exceed 6%.
4. Conclusions
Using the extract of Musa paradisiaca peel (banana) as a natural solvent, the synthesis of silver nanoparticles was carried out through an environmentally friendly method, compared to conventional chemical methods. This method is characterized by its easy operation and low cost, contributing to the environmental richness achieved when no toxic compounds are used in the synthesis stage.
The synthesis of the silver nanoparticles was demonstrated using characterization techniques (UV-Vis and XRD). These tests showed the existence of the silver nanoparticles obtained in the synthesis, since by UV-Vis the maximum absorbance peak was observed, of 411 nm, in the range of 400 to 450 nm. By XRD, it was evidenced the face-centered cubic crystalline phase of the silver, with diffraction peaks positions in 2theta of 38°, 45°, and 65°, and it was determined that it has an average particle size of 21.8 nm by means of the Debye-Scherrer equation. These results correspond to those obtained with the silver nanoparticles described in other studies.
This study shows that at room temperature, the phytochemical components present in the extract of Musa paradisiaca's peels can promote the formation of silver nanoparticles; such analysis mainly indicated the presence of these compounds: 1.2 Ethanediol (60.0261 %) and 2.3 Butanediol (11.2 %), which facilitate the reduction of silver ions, contributing to the formation of nanoparticles, and acetic acid (1.7376 %) which helps to stabilize the AgNPs by behaving as a primary surfactant during the nucleation stage of this nanomaterial.