Introduction
Cyanobacteria, also known as blue-green algae, are an unique group of photosynthetic prokaryotes with worldwide distribution, characterized by their great morphological diversity, pigmentation and oxygenic photosynthesis (Wehr et al., 2015). The production of cyanobacteria and their cultivation systems have interes-ted researchers given their commercial importance (Hultberg et al., 2013). They have been widely studied because of their enormous biotechnological potential, mainly due to the ability to synthesize different biomolecules for nutritional, pharmaceutical and industrial applications (Nowruzi et al., 2018); including the production of food, fuel and oxygen on Mars (Verseux et al., 2016). Given the versatility of employing cyanobacteria, several studies have focused on their production and economic yield (Barone et al., 2019).
Among cyanobacteria, the most interesting genera, from a biotechnological point of view, are Spirulina, Anabaena and Nostoc (Wehr et al., 2015). Nostoc genus is used for medical applications and human nutrition in countries like China, Japan, India and Colombia (Liao et al., 2015; Guo et al., 2016; Benítez et al., 2018). This cyanophycea produces active antiviral substances against the human immunodeficiency virus (HIV), and also antibiotics that can control cryptococcosis, a secondary fungal infection typical of the acquired immunodeficiency syndrome (AIDS) (Raja et al., 2016; Lotfi et al., 2017). In addition to nutritional and medical applications, Nostoc had too been used as biofertilizer in different crops such as rice, canola, tomato, corn, wheat, peas, and oats. As biofertilizer, Nostoc can contribute to nitrogen fixation, phytohormones synthesis and production of hygroscopic polysaccharides that help plant growth, and, it can also prevent erosion and improve soil properties (Dhar et al., 2015; Ranjan et al., 2016). Moreover, the species Nostoc muscorum synthesizes polymers such as the polyhydroxybutyrate thermoplastic that is used for clinical and industrial applications (Haase et al., 2012; Ansari and Fatma, 2016); and, unsaturated fatty acids of pharmaceutical interest like α-linolenic acid (18:3) (Kim et al., 2015). Additionally, the ultrastructure of N. muscorum can be exploited for the production of based-hydrogen fuel (Shah et al., 2003; Singh et al., 2017).
The cultivation of microalgae is a globally emerging industry with great commercial potential (Hultberg et al., 2013). Traditionally, the main alternatives for cultivating microalgae are raceway pond systems and photobioreactors. However, N. muscorum tends to present a low growth rate and an abnormal growth pattern when in liquid medium without any support; also, the traditional cultivation systems are still expensive and need complex technological developments and technical expertise to be cost efficient and environmentally sustainable (Zhang et al., 2017). The species N. muscorum does not have an optimal growth in liquid medium because the agitation tends to adhere the cells to each other, form biofilms, and migrate towards the part where there is no culture medium; consequently, the cyanophycea undergoes depigmentation and rapid cell death due to the lack of nutrients (Diao and Yang, 2014; Rusydi et al., 2015; Lotfi et al., 2017). Moreover, the mucilaginous envelope of N. muscorum cannot be formed properly, thus, allowing opportunistic bacteria and fungi to degrade it (Rusydi et al., 2015; Cui et al., 2017). Therefore, a successful strategy for increasing the biomass production in cultivation systems of filamentous cyanobacteria that do not have a good adaptation to liquid conditions, e.g. N. muscorum, requires the optimal utilization of a solid substrate (Barone et al., 2019). The use of an inert support allows the adhesion of periphytic microalgae, thus, an optimal growth and biomass production (Vorndran and Lindberg, 2016; Cui et al., 2017). Additionally, for a high growth rate, a culture system that allows the exchange of heat and nutrients is necessary (Alatorre-Cobos et al., 2014).
Hydroponic systems have shown to be cost-effective and environmentally sustainable in previous studies with cyanobacteria Calothrix sp., Anabaena laxa and Anabaena azollae (Alatorre-Cobos et al., 2014). The hydroponic method is mainly used as an alternative for wastewater treatment aimed at a more effective reduction of nutrients (Bawiec et al., 2019). A great support for the purification processes in such systems are microalgae due to their use of inorganic forms of nitrogen and phosphorus, and the ability to uptake heavy metal ions, organic pollutants, and coliform bacteria (Abdel-Raouf et al., 2012; Ye et al., 2016). Together with their space efficiency, low cost and cleanliness, hydroponic systems have become increasingly popular as an ecofriendly method for wastewater treatment; which happens to be an ideal nutrient solution for the development of cyanobacteria used for the production of biofuels (Singh et al., 2017). On the other hand, hydroponic systems have also been studied for co-cultivation of plants and microalgae. Zhang et al., (2017) and Barone et al., (2019) showed that the hydroponic co-cultivation system was beneficial for the growth of both plants and microalgae, resulting in a biostimulant effect for crop biomass provided by algal photosynthesis, while crop root respiration and exudation acted as sources of carbon that increased microalgal biomass. Furthermore, hydroponic systems with cyanobacteria have been used in the process of priming rice and wheat plants (Babu et al., 2015; Bidyarani et al., 2015). This is mainly supported by studies on biofilm formation (Prasanna et al., 2018), and colonization of root tissues (Bidyarani et al., 2015). Bharti et al., (2019) also studied co-culturing as a promising option for seedlings development before planting in pots or field, finding that hydroponic co-cultivation of chrysanthemum with cyanobacteria provided a continuous supply of oxygen gradients, carbohydrates, and nitrogenous compounds, that had an effect on the proliferation of roots and growth of cuttings, and observed a synergistic behaviour of both parts in terms of biomass accumulation.
Hence, in order to use hydroponic systems for exploiting the biotechnological potential of cyanobacteria, there is a need for monitoring their growth first. The aim of this study was to analyze the growth of N. muscorum in a modified hydroponic system, as an alternative for biomass production.
Materials and methods
Preliminary tests
The biomass production of two Nostoc species was evaluated:
Nostoc ellipsosporum (Göttingen University B1453-79): obtained from the Institute of Plant Biochemistry and Photosynthesis (IBVF-cicCartuja) of the University of Seville (Spain). Filamentous cyanobacterium; with trichomes (chain of cells) that grows by intercalar cell division. Reproduction by formation of hormogons, random breakage of the trichome, and, sometimes by germination of acinets. In the absence of combined nitrogen, trichomes have heterocysts, some also acinets. Cellular division in one plane.
Nostoc muscorum (code number 026): obtained from the collection of freshwater algae in the Botany Department of the Federal University of São Carlos (UFSCar, Brazil). Cells are filamentous, green-brown colored, and can form spores under desiccation conditions. Heterocysts (5-10% of cells) appear when transferred to nitrogen free media. Cells possess a motile stage in its life cycle through the use of hormogones.
Culture medium: The hydroponic solution composition was 0.64 g/l of calcium nitrate (Calcinit®), 0.4 g/l of potassium nitrate (K Crista®), 0.08 g/l of monoammonium phosphate (MAP® Crista), 0.34 g/l of magnesium sulfate, 0.015 g/l of chelated iron in EDDHMA (M48® Rexolin Yara), and 0.02 g/l of micronutrients (Mg, S, B, Cu, Fe, Mo, Mn) (KSC Roullier mix®). This solution was diluted in the following volume/volume percentages: 30% (H30%), 40% (H40%), 50% (H50%), and 60% (H60%).
A test was developed to determine the optimal concentration of hydroponic solution for the N. ellipsosporum culture with the following treatments: BG-11 (UTEX) (Vaara et al., 1979), H30%, H40%, H50% and H60%, each by triplicate in 100 ml Erlenmeyer flasks, with an initial cellular concentration of 3.4 x 105 cells/ml each. Optical density was measured at 650 nm on days: 0, 2, 3, 4, 5 and 8. The controlled laboratory conditions were as follow: 32.4 ± 0.1 µmol.photons/m2/s light intensity, 12 hours of photoperiod, and 23°C temperature.
Lastly, for the selection of the strain with the highest biomass production yield, a comparison was made between N. ellipsosporum and N. muscorum in the H50% culture medium by triplicate in 100 ml Erlenmeyer flasks, with an initial cellular concentration of 2.8 x 104 cells/ml. Optical density data was measured at 650 nm on days: 1, 4, 6 and 7. The controlled laboratory conditions were as follow: 32.4 ± 0.1 µmol.photons/m2/s light intensity, 12 hours of photoperiod, and 23°C temperature.
Nostoc muscorum culture in a modified hydroponic system
N. muscorum inoculum was grown in 1 l Erlenmeyer flasks, with 500 ml of hydroponic solution until cellular density reached 2 x 106 cells/ml. Later, the cell culture was transferred to the hydroponic system inside a greenhouse located at the Algae Biotechnology Laboratory of the Botany Department at the Federal University of São Carlos (UFSCar, Brazil), 21°58’59.60’’ S - 47°52’47.79’’ W, 854 masl. Microalgae grew under the following conditions: 895 ± 18 µs/cm electrical conductivity, 23°C temperature, pH 7, 32.4 ± 0.1 µmol.photons/m2/s light intensity, and 12 hours photoperiod.
Experiments were carried out in an inert material hydroponic system (Figure 1) composed by a feeding tank (10 l), a positive flow pump and a box with inclined support covered by a pore size 5 µm cloth. The system was disinfected with 70% ethanol. 10 l of the hydroponic solution was used to feed the system, which was inoculated with 6.3 ± 3.6 x 104 cells/ml of N. muscorum. The following parameters were monitored on alternate days: pH, temperature, electrical conductivity, and light intensity (measured at 10 am everyday); using a portable pH meter (HI98108 - Hanna, Hungary), conductivity meter (CDC401 - Hach, USA) and a light meter (0713700 - Phywe, Germany). The pH was regulated with the addition of 9.4 x 10-4 M NaHCO3.
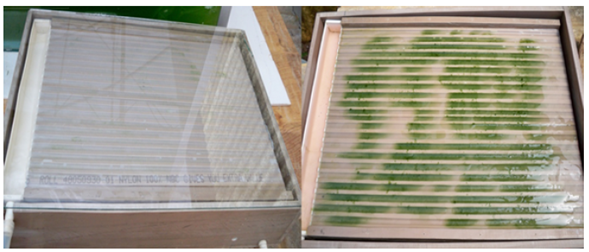
Figure 2 Appearance of N. muscorum growth in a hydroponic system with a solid substrate. Left: Day 1. Right: Day 4.
As days went by, the N. muscorum cells covered the whole cloth, making it necessary to harvest the biomass in order to avoid “self-shading”. The harvest of biomass was done by scraping on the following days: 6, 8, 13, 16, 20, 22 and 23. The algae biomass collected from the hydroponic system was dried in a convection oven (315SE - Fanem, Brazil) at 37°C for 48 to 72 hours, depending on the biomass humidity, and until reaching a constant weight. Dry biomass was weighed in an analytical balance with 0.0001 g resolution (TE214S - Sartorius, Germany). To verify cell adhesion of N. muscorum on the cloth, 2 ml samples of the hydroponic solution were taken during the sampling days. These samples were fixed with one drop of Lugol’s solution, stored in microcentrifuge tubes and analyzed by light microscopy.
Statistical analysis
Mean values of triplicates were calculated using Microsoft Excel 2017 including standard deviation (SD) values as depicted in the error bars of the Figures 3, 4, 5, 6 and 7. Data of the strains growth and biomass production were analyzed using the analysis of variance test, one-way ANOVA, with a confidence level of 95%. Correlations were also calculated in Microsoft Excel 2017 at P value of 5%, using a linear analysis of mixed effects, for the factors: pH, temperature,electrical conductivity and biomass production of N. muscorum.
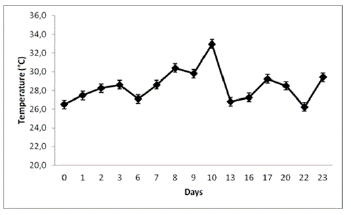
Figure 3 Temperature fluctuations in the greenhouse where biomass production of N. muscorum on a solid substrate within the hydroponic system was helded
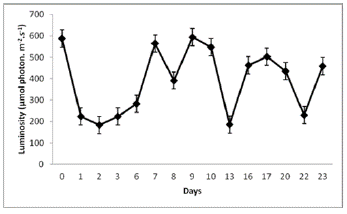
Figure 4 Luminosity fluctuations in the greenhouse where biomass production of N. muscorum on a solid substrate within the hydroponic system was helded
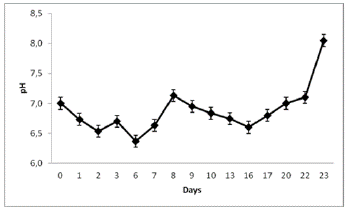
Figure 6 pH fluctuations during the biomass production of N. muscorum on a solid substrate within the hydroponic system.
Results
Preliminary tests
To determine the optimal concentration of hydroponic solution for the Nostoc culture, five treatments (BG-11, H30%, H40%, H50% y H60%) were evaluated, obtaining the following optical density values: BG-11 0.051 ± 0.001 OD650; H30% 0.044 ± 0.001 OD650; H40% 0.045 ± 0.001 OD650; H50% 0.069 ± 0.001 OD650; and H60% 0.054 ± 0.001 OD650. The ANOVA showed no significant differences among the treatments of the diluted hydroponic solution and the BG-11 reference medium (P value 0.784). Nevertheless, the H50% treatment displayed the highest value of optical density during the experiment.
The biomass production of two Nostoc species was evaluated in the H50% treatment according to their optical density values. The ANOVA exhibited significant differences between the strains (P value 0.012), indicating that the strain of N. muscorum had a significant greater growth with an OD650 of 0.087 ± 0.001. On the other hand, N. ellipsosporum reached an OD650 of 0.066 ± 0.001.
Nostoc muscorum culture in a modified hydroponic system
A high growth rate of N. muscorum was observed in the hydroponic system with a porous cloth under semicontrolled environmental conditions (Figure 2). Figures 3 and 4, indicate the temperature and luminosity variations in the greenhouse. Moreover, for further analysis of N. muscorum growth, the dry biomass production (Figure 5), pH (Figure 6) and electrical conductivity (Figure 7) were daily measured. Only a modified hydroponic system was evaluated in the present work.
The obtained average biomass productivity was 0.4149 ± 0.0207 g/m2/day, and the maximum daily production was 0.2276 ± 0.0114 g/m2/day. The maximum biomass production of N. muscorum was obtained on day 13 with 0.3185 ± 0.0159 g/m2/day (Figure 5). This result was obtained after seven days of high temperature and luminosity, as shown in Figures 3 and 4. The total biomass produced by the culture system was 0.0053 ± 1.0689 g/m2/day on day 23.
Correlation analysis by mixed effects allowed to identify that there was no dependence between pH (P value 0.3360), temperature (P value 0.7504), electrical conductivity (P value 0.6633), and N. muscorum biomass production factors. Therefore, the algal growth was only influenced by the physiology of the species under the evaluated culture conditions.
Discussion
Nostoc muscorum is a cyanophycea with great biotechnological potential due to the products that can be obtained from its biomass. However, conventional liquid cultures are not efficient for biomass production of N. muscorum, as well as for other filamentous cyanobacteria (Yu, et al., 2009; Cui et al., 2017). Diao and Yang (2014) and Rusydi et al., (2015), found that conventional liquid culture generates disintegration of colonies of Nostoc species, mainly associated with bacteria and fungi propagation. At the same time, periodic exposures to desiccation could cause malformation of the exopolysaccharide layer, because the Nostoc sheath can have its structure modified and become the nutritive base for microbial growth (Rossi and De Philippis, 2015; Widder et al., 2016).
In this way, the viability of biomass production of N. muscorum cultivated in a hydroponic system is demonstrated, presenting several advantages:
It does not require drying periods like conventional cultures implemented by Cui et al., (2017). This is possible because the substrate (cloth) acts as a support for the adherence and development of the algae mucilaginous layer, allowing the continuous flow of nutrients and preventing a dominant growth of opportunistic bacteria and fungi, resulting in a high rate of algal growth (Rossi and De Philippis, 2015).
N. muscorum culture in a hydroponic system maintains the textural elasticity for colony sheath formation, which the algae use to prevent cell disintegration and to generate proteins resistant to drought and UV rays (Rossi and De Philippis, 2015).
The continuous flow of oxygen provided by the pump in the hydroponic system depresses bacterial growth (Widder et al., 2016). In fact, during the active growth of N. muscorum in the hydroponic system, the disintegration of colonial filaments was significantly delayed, suggesting a reduction in the opportunistic microorganism activities.
Furthermore, according to the monitored parameters, the maximum algae growth occurred after several days of high luminosity and temperature (Figures 3 and 4). These results could rely on the fact that N. muscorum adapts very well to environmental conditions with high exposure to UV light, unlike other cyanobacteria (Singh et al., 2011; Rosales-Loaiza et al., 2016). Generally, the temperature change from 15°C to 30°C does not affect morphological development, even in conventional liquid culture conditions. This Nostoc UV light insensitivity is generated by the presence of Mycosporin and Scytonemin type amino acids in the sheath (Ferroni et al., 2010). In this study, N. muscorum tolerated a greater intensity of light (391.8 µmol.photons/m2/s) and temperature (33°C) in the hydroponic culture system, as shown in Figures 1 and 2. The obtained biomass values, 0.4149 ± 0.0207 g/m2/day surpass the results from Cui et al., (2017) in conventional culture systems. Since the water flow in the hydroponic system allowed the release of heat in the form of steam, that was condensed in the same system, water loss and consequent changes in electrical conductivity were minimized (Figure 7). These two features can reduce water and monitoring costs in the maintenance of cyanobacteria growth.
The decrease in the pH value of the hydroponic solution during the cyanobacteria growth could be due to the excretion of organic acids and metabolic products associated with phosphorus solubilization in the environment (Haase et al., 2012). This ability confers an ecological advantage to the species compare to other microalgae because of its possible applications in biofertilizer production (Dhar et al., 2015; Flores et al., 2015).
Furthermore, microscopic analysis of the hydroponic solution showed the absence of cells in the fluid, which indicates that N. muscorum completely adhered to the substrate using its mucilaginous layer of exopolysaccharides (Singh et al., 2011; Singh et al., 2012)
Finally, the hydroponic system with solid support can be manufactured with common elements and requires simple monitoring and harvesting, representing an economical alternative using available technology for cyanobacteria culture. Besides, this hydroponic system is more affordable than other farming systems because it does not need automatic desiccation as in conventional crops. In conclusion, algal biomass production in a hydroponic system with solid support proved to be a viable alternative for the growth of peryphytic filamentous cyanobacteria such as N. muscorum in an indefinite culture medium with nutrients excess (hydroponic solution), compared to the BG-11 medium which is a defined one.