1 Introduction
The enzymatic method is the most widely used to obtain inverted syrup with invertase (EC.3.2.1.26) from Saccharomyces cerevisiae strains 1. However, the lifetime of invertase is short, and the bioactive species are inestable and less selective. The immobilization of invertase extends its lifetime, and the stability of bioactive species is enhanced due to the protection of the support. These characteristics are useful in bioreactors because the separation of the products is easier, and the enzymatic recovery is efficient 2. So, different ways to immobilize invertase have been developed. Invertase has been immobilized on supports such as montmorillonite micropores 3, polyacrylonitrile composite fibers 4, functionalized silicon pores 5, nylon microspheres 6, chitosan nanoparticles 7, calcium alginate 8)(9 and polyaniline-modified surfaces 10, hydrogel comprising of methacrylic acid (MAAc) and Η-vinyl pyrrolidone (Η-VP) 11, among others. As a result, these studies reported that immobilized invertase is most selective allowing the formation of bioproducts derived from glucose and fructose cyclization with temperatures ranging from 30 to 40 °C. However, in packed-bed reactors when small particles are used for invertase immobilization high pressure drops occur, a disadvantage that can be solved by using magnetic particles 12.
Invertase immobilization by covalent bonding on magnetic particles has been performed using nanocomposites of magnetic polyvinyl alcohol microspheres 12, or chitosan- coated /-Fe2O313, and in magnetic diatomaceous earth nanoparticles 14, Recently,with the aim of increase the enzyme adsorption Bayramoglu et al.,15 studied the co-valent immobilization of invertase by modifying magnetic nanoparticles of Fe3O4@SiO2 with gly-cidyl methacrylate (GMA). Uzun et al. 16 immobilized invertase on polyamidoamine (PAMAM)-magnetite nanoparticles functionalized with amino-propyltrimethoxysilane (APTMS), which were then used for successive step-by-step addition of methy-lacrylate and ethylenediamine to form the dendritic structure of PAMAM on the superparamagnetic nanoparticles.
Others methods involved the use of silica-coated magnetic nanoparticles providing many silanol groups on the surface which can be used in the subsequent surface functionalization with organosilanes. However, lowest retained enzyme is obtained when Fe3O4 nanoparticles are directly modified. In this sense, a step of cross-linking agent is necessary to obtain a better immobilization and to avoid the leaching of the enzyme. Besides, a subsequent activation with glutaraldehyde as cross-linking agent favors enzymatic stability due to the multipoint covalent attachment 17. Fe3O4 covered by SiO2 prevents the loss of the magnetic properties and increase the amount of immobilized enzyme 18. Previous results reported by our group using X-ray photoelectron spectra demonstrated the absence of signals associated with Fe 2 p3/2 and p1/219. This suggested that the Fe3O4 particles were totally covered when the particles were previously covered with SiO2 and again treated with polyethyleneimine (PEI) and glutaralde-hyde. Besides, with Fe3O4-SiO2 a larger amount of aminopropylethoxysilane (APTES) could be anchored increasing the amount of enzyme adsorbed. In this study, we studied magnetic nanoparticles functionalized with APTES and posteriorly modified with a cross-linking agent to improve the invertase retention.
2 Experimental
2.1 Synthesis of Fe3O4-SiO2-NH2magnetic nanoparticles
The magnetic nanoparticles were synthesized by the alkaline co-precipitation method proposed by Kang et al.20 using a molar ratio of Fe(II)/Fe(III) = 0.5; then, these nanoparticles were encapsulated with SiO2 following the Stõber method 21. A ratio 1:1 of magnetic particles and the silica source was employed in the synthesis of Fe3O4 -SiO2. The functionalization these particles with APTES (99%) was performed following the protocol described by Diez et al. 22 using APTES as a source of amino groups. These Fe3O4-NH2 SiO2- particles were used as support for immobilization by physical adsorption.
2.2 Synthesis of activated Fe3O4-SiO2-NH2 magnetic nanoparticles
For immobilization by covalent bonding, Fe3O4-SiO2-NH2 nanoparticles were activated following a protocol previously established by our group 19. The procedure was based on the suspension of the support in functionalizing agents to provide carboxyl and secondary amino groups. To do this, the particles were suspended in a glutaraldehyde solution and vigorously stirred. Deionized water was used to filter and wash the solution, and once again resuspended in a solution of polyethylenimine (PEI) 5% (w/v) sodium tetraborate buffer. This solution was incubated at 25 °C for 3 h.
Subsequently, the support was resuspended in 15 mL of a solution of 1 % glutaraldehyde in 0.05M acetate buffer for reactivating the functional groups bonded to the substrate; this solution was incubated at 25° C for 15 min. The activated support was filtered and washed with sufficient deionized water to remove the excess of glutaraldehyde. Finally, the support was conditioned with 0.05M acetate buffer at pH 7.0. These particles were denominated activated Fe3O4-SiO2-NH
2.3 Invertase immobilization
Invertase (j6 -D-fructofuranosidase) from Saccha-romyces cerevisiae (Fluka Chemie) was immobilized by physical adsorption on Fe3O4-SiO2-NH2 and co-valent immobilization on activated Fe3O4-SiO2-NH2 supports. The enzyme was dissolved in acetate buffer (0.05 M, pH = 5.5) using an enzyme : support ratio of 1:2, and the solution was mechanically stirred for 24 h at 100 rpm at 4?C. Then, the support impregnated with the enzyme was collected, washed, and resuspended in acetate buffer for further use and stored at 4 ?C for further enzymatic activity ssays. The amount of protein bound to the support was determined by difference between the initial and final amount of protein using the Lowry method using Follin-Ciocalteau reagent at 600 nm 23.
Miller method (DNS) 24 was used to determine the reducing sugars released from the hydrolysis of sucrose by the free and immobilized enzyme. The enzyme was incubated at 50°C in 0.3 M sucrose solution in 0.05M acetate buffer, pH 5.5. For this study an international activity unit (IU) was defined as 1 /¿mol of sucrose hydrolyzed per minute under the test conditions. Specific activity was expressed as IU per mg protein.
The effectiveness factor (Fe) was determined as the retained activity after the immobilization process and calculated from the following equation expressed as:
where Ai is the activity of the immobilized enzyme, Ao is the activity of the free enzyme, and Ae is the enzymatic activity remaining in the solution after immobilization.
The relative activity is the ratio between the enzymatic activity of the immobilized enzyme and the enzymatic activity of the free enzyme, and it was expressed as:
The efficiency factor (η ) determines the effect of diffusional limitations or alterations in mass transfer with respect to the reaction speed: it was calculated from the maximum reaction speed of the immobilized enzyme divided by the reaction speed of the free enzyme:
2.4 Variable optimization
The immobilization time was assessed within a range of 0 to 24 h, and the support-enzyme ratio (0 to 5000 mg enzyme /g support) at pH 5.5. To carry out the optimization of enzyme immobilization conditions, in each experiment only one variable was modified, all other variables kept steady.
2.5 Effect of pH and optimum temperature on the immobilized enzyme
The optimum pH was evaluated in a range from 4.0 to 7.0 at room temperature. The incubation temperature was evaluated in a range from 40 to 70 °C.
2.6 Determination of kinetic parameters (Vmax, km, and ki )
The Vmax and k m kinetic constants were assigned as response variables and determined under optimum conditions for both immobilized and free enzymes. This parameter was calculated from the Lineweaver-Burk plot using different substrate concentrations in a range from 0.025 to 2.0M. The inhibition constant (k i ) was calculated with the substrate Dickensheets model.
2.7 Stability studies and reusability
Stability was evaluated in a batch of immobilized invertase for 90 days at a storage temperature of 4 ° C using an aliquot of 100 /iL of immobilized invertase.
The reuse of immobilized enzyme was evaluated using the same amount of enzyme immobilized by several cycles while a constant concentration of substrate (sucrose) was maintained. The immobilized enzyme was evaluated until reaching enzymatic activity loss or constant. After each cycle, the excess dye (DNS) was removed washing the support with distilled water.
3 Results and discussion
Effectiveness factor (Fe) obtained for invertase immobilized by physical adsorption is relatively low (Fe = 0.26) compared with that of covalent immobilization (Fe = 0.74). The Fe value relates to the porosity of the support used, and porous supports cause some diffusional effects decreasing this value. In fact, the activated Fe3O4-SiO2-NH2 particles have lower size and pore volume values, as has been described previously 25. Besides, electrostatic interactions are obtained when the enzyme is only immobilized in the magnetic composites modified with APTES. To assure covalent interactions, we used the functionalization of the support to provide carboxyl and secondary amino groups. The PEI assures an amount sufficient of amine groups, and the glutaraldehyde allows to obtain carboxylic groups. This density of -NH2 and C = O groups is necessary to anchor the enzyme and to assure more porosity of the support.
Figure 1a shows the relative activity of invertase immobilized by physical adsorption or immobilized covalently. In the physical adsorption of invertase, when the enzyme concentration increases, the relative activity decreases as a result of leaching processes. On the contrary, this phenomenon is not obvious until an enzyme/support ratio of 1500 mg/g in invertase immobilized covalently. That is, in physical adsorption more enzyme molecules are adsorbed than in covalent immobilization because there are more amino groups available to bind with the enzyme in comparison with groups available in a reticulated support (activated Fe3O4-SiO2-NH2). However, a saturation surface of enzymes promotes diffusional limitations due to the blockage of the active sites by vicinal proteins 6, 7. The optimum enzyme:support ratio is higher for covalent bonding than for physical adsorption, being 1500 and 1000 mg/g of enzyme/support, respectively (Figure 1b).
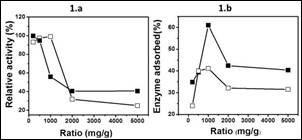
Figure 1 Effect of the support:enzyme ratio on the relative activity (Figure 1.a) and enzyme adsorbed (Figure 1.b) by physical adsorption (■) or covalently bonding (□).
Figure 2a and b displays the optimum time for invertase immobilization in both methods, which occurs at 24 h. Consequently, the protein values obtained are near 60% for physical adsorption and 50% for covalent bonding, i.e., in the physical adsorption a smaller amount of enzyme is immobilized than in covalent bonding, a higher enzyme:support ratio being required in this type of immobilization (Figure 2a) . Interestingly, the enzyme immobilized covalently has a higher activity compared with the enzyme physically retained as can be seen in the Figure 2b). This result is attributed to the fact that the reticulation of the enzyme avoids its anchor on active sites, which is probably occurring in the physical adsorption.
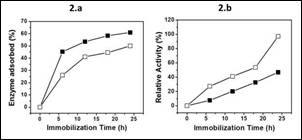
Figure 2 Effect of immobilization time on the enzyme adsorbed (Figure 2a) and relative activity (Figure 2b) by physical adsorption (■) or covalently bonding (□_).
The results of enzymatic activity of the immobilized enzyme at different pH values are shown in Figure 3. It has been reported that depending on the support used, the pH range varies between 4.5 and 5.5 5 6,25. In our study the ideal pH for the free enzyme is 5.5, whereas in the enzyme immobilized by both methods the pH is 5.0. However, it is important to note that the relative activity at pH 7.0 is higher for the invertase immobilized by covalent bonding. The displacement of 0.5 pH units to the acidic region can be explained by secondary interactions between the enzyme and the support. Considering that the support was modified with PEI and glutaraldehyde to increase the interaction of the enzyme on the reticulation process, probably not all the -NH2 groups providing PEI interact with enzyme. This excess of groups can act as buffer in changes of pH in the medium favoring the enzyme functioning in a broad range of pH, i.e. the reticulation not affects the active sites of the enzyme.
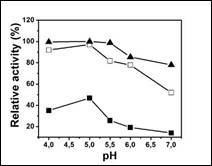
Figure 3 Effect of pH on the relative activity of invertase free (A) and immobilized by physical adsorption (■) or covalently bonding (□).
Figure 4 shows profiles of relative activity of free and immobilized enzymes with respect to temperature. The enzymatic activity of free and immobilized invertase was near 90% and was maintained until 70 °C. At higher temperatures, the activity decreased. However, in the enzyme adsorbed physically the best temperature was 60 °C, but the relative activity was only of 75%, because of a lower binding force between the enzyme and the support 4. It is also remarkable that the immobilization process allowed greater retention of relative activity above the critical temperature (80 °C). While the free enzyme retained only 20% of its relative activity, in covalent and physical immobilization this value was 40% and 30%, respectively.
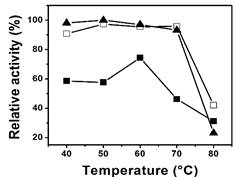
Figure 4 Effect of temperature on the relative activity of invertase free (A) and immobilized by physical adsorption (■) or covalently bonding (□).
Table 1 shows the kinetic parameters determined using the Excel solver software with a nonlinear regression method (Lineal-Newton). The obtained values are consistent with the Michaelis-Menten model and the kinetic equation of substrate inhibition. The Vmax of the enzyme immobilized by physical adsorption is half of that obtained for the free enzyme due the presence of noncompetitive inhibitors, which is evidenced by the higher value for ki (1.21 for free enzyme, 2,95 for physical adsorption). In contrast, the enzyme immobilized by covalent bonding has a higher Vmax value than the free enzyme, which is attributed to possible changes in the structure of the enzyme allowing further decoupling of the product/enzyme complex. Besides, the similar values of ki (1.75) indicate that the covalent immobilization method provides greater stability to the enzyme, preventing its inhibition 5, 13, in agreement with the efficiency factor (η ) obtained.
Table 1. Kinetic parameters for free and immobilized invertase and comparison with other studies on different supports
Support | Method | Vmax (UI/mg enzyme) | km (mM.ml1) | Efficiency factor (η) | Ref |
---|---|---|---|---|---|
Free enzyme | - | 1.86 | 0.0087 | - | This paper |
Fe3O4-SiO2-NH2 | P.A. | 0,96 | 0,053 | 0,51 | This paper |
Activated Fe3O4- SiO2-NH2 | C.I. | 2.70 | 0.054 | 1.45 | This paper |
Montmorillonite micropores | P.A. | 0,000514 | 0,72 | 0,28 | [3] |
Polyacylonitrile composite fibers | P.A. | 0,95 | 0,031 | 0,64 | [4] |
Montmorillonite micropores | C.I. | 0.000657 | 0.39 | 0.36 | [3] |
Functionalized porous silicon | C.I. | 1.243 | 0.037 | 1.31 | [5] |
Magnetic polyvinyl alcohol microspheres | C.I. | 0.97 | 0.55 | 0.80 | [12] |
Nylon-6 microbeads | C.I. | 1.06 | 0.029 | 1.29 | [6] |
Chitosan nanoparticles | C.I. | 1.83 | 0.20 | 1.09 | [7] |
Surface-modified polyaniline | C.I. | 0.052 | 0.67 | 0.71 | [10] |
The values of km were similar in both immobilization methods and 6-fold higher compared to the free enzyme. Conformational changes in the enzyme hinder the access of sucrose molecules to the active sites of the invertase and therefore the formation of the enzyme-substrate complex is more difficult, leading to a decrease in substrate affinity and a high k m value 3 4.
A comparison of kinetic parameters with those of other studies reported in the literature also is observed in the Table 1; however, in the physical adsorption is only an estimate to observe the increase or decrease of the values of Vmax, km and h. A direct comparison was not possible due to the different conditions employed in each study.
Although, few supports have been reported by the physical adsorption of invertase, our results do not differ of those reported by other authors using supports with high efficiency factors (h) and high value of Vmax. In this work, values of efficiency factor (h), Vmax, and km obtained with invertase immobilized on different supports by covalent bonding were like those obtained by Azodia et al with invertase immobilized on functionalized porous silicon using glutaraldehyde as a functionalization agent. They showed that the lowest values of k m reflect a decreasing of enzyme affinity versus the substrate upon immobilization mainly due to eventual conformational changes. Also, highest values in Vmax were attributed to several phenomena such as favorable change of enzyme immobilized structure and enhanced dissociation of the product from the product/enzyme complex. In this study, we can describe similar reasons in our results. In all case, the affinity of the enzyme decreased upon immobilization (increase of km), the value Vmax increased significantly, and a higher efficiency factor (η ) provided greater stability of the enzyme over time.
The stability of the invertase physical and covalently immobilized was also studied for 90 days; the results are summarized in Figure 5. For the invertase immobilized by physical adsorption a loss of activity of approximately 25% occurred during the first 16 days, mainly due to enzyme denaturation processes. However, in the enzyme immobilized by the covalent method 50% of activity was observed at 30 days, which is similar to the stability obtained by Azodi et al.5 using functionalized silicon pores as support. Glutaraldehyde activated supports have been reported to provide multipoint covalent enzyme immobilization improving enzymatic rigidity, and, thus, enzyme stability17. Because the reticulation maintains an intense multipoint interaction with the enzyme, the relative positions of all groups involved in the immobilization cannot alter the relative positions of the enzyme.
The immobilized enzyme reusability was studied five times (Figure 6) using an external magnetic field. A 50% and 60% loss of activity occurred in the first reuse for the enzyme physically or covalently immobilized. The same behavior was observed in subsequent reuses, mainly due to leaching of the enzyme to the magnetic support, which was verified by monitoring the first residue, showing that the enzymatic activity was maintained. Other authors such as Bayramoglu et al.4 reported a 76% loss of activity due to leaching.
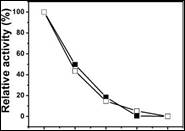
Figure 6 Reuse of invertase immobilized by physical adsorption ((■)_) and covalent bonding (□_) in terms of relative activity (%).
Table 2 summarizes the parameters evaluated in the immobilization of invertase using the physical adsorption and the covalent bonding method. Cova-lent immobilization of invertase on composites of Fe3O4-SiO2-NH2 displays higher efficiency in the immobilization process (Fe), higher catalytic efficiency, less inhibition and twice as much stability. In fact the stability is increased two fold when is used the covalent bonding method. Furthermore, immobilized invertase by physical adsorption or covalent immobilization can be reused for up to four cycles, and using an external magnetic field can be removed from the reaction medium.
Table 2 Parameters evaluated in the immobilization of enzymes in Fe3O4-SiO2-NH2 and activated Fe3O4-SiO2-NH2
Parameters | Invertase immobilized | |
---|---|---|
Fe3 O4-SiO2 -NH2 | Activated Fe3 O4-SiO2-NH2 | |
Effectiveness factor (Fe) | 0.26 | 0.74 |
Efficiency factor (n ) | 0.51 | 1.45 |
Catalytic efficiency | 17.89 | 50 |
Vmax | 0.96 | 2.70 |
k m | 0.053 | 0.054 |
ki | 2.95 | 1.75 |
pH | 5.0 | 5.0 |
Thermostability | 60 ° C | 50 ° C |
Stability | 30 days | 60 days |
Reusability | 4 cycles | 4 cycles |
4 Conclusions
Invertase was immobilized on magnetic composites by physical adsorption or covalent immobilization in magnetic composites. The support activated with glutaraldehyde improved the retention, stability and catalytic efficiency of the invertase, besides the enzyme showed less inhibition. The catalytic activity of the enzyme immobilized by both methods was not affected by different pH and temperatures in comparison with the free enzyme. The enzyme immobilized in the magnetic composites studied can be reused for up to four cycles and can be removed from the reaction medium using an external magnetic field