Introduction
Taro, Colocasia esculenta L. Schott (Araceae) is an annual herbaceous plant that is cultivated in tropical and subtropical areas worldwide, guaranteeing the economy and food security in many countries (Ebert & Waqainabete, 2018; Ubalua et al., 2016). Taro tubers are an excellent source of carbohydrates, as taro contains 70-80 % starch. In addition, due to the small size of its starch granules, taro is highly digestible (Ahmed & Khan, 2013). Unlike sweet potato and yam starches (28.3 and 251 µm), taro, with an average particle size of 1.067-64.19 microns, is more suitable when improved binding and a reduced rupture capacity for pharmaceutical applications are required (Aprianita et al., 2009).
Taro tubers possess a wide range of phytochemical compounds including flavonoids, condensed tannins, β-sitosterol, and vitamins B1, B3, and C, with a long history of medicinal uses in the treatment of asthma, arthritis, diarrhea, internal bleeding, neuronal and skin disorders, in addition to analgesic, anti-inflammatory, anti-cancer and hypolipidemic effects (Prajapati et al., 2011).
In intensive pig farming, weaning is carried out early (21 days of age), and subsequent to this process, important changes occur within the piglet’s gastrointestinal tract (GIT) due to the low digestion capacity of solid diets, with the consequent occurrence of diarrheas in these animals (Flores et al., 2015). Diarrhea is commonly associated with the proliferation of enterotoxigenic Escherichia coli in the pig’s intestine, and colistin, a cationic antibiotic for oral use, is widely used for its treatment. However, despite the effectiveness of this antibiotic in the treatment of diarrhea in pigs, many studies report high rates of E. coli resistant to colistin, and, currently, there are related concerns with the increase of bacterial strains, potentially tolerant to antibiotics (Clark et al., 2012; Rhouma et al., 2017).
Given this situation, intense work has recently been carried out to minimize the use of growthpromoting antibiotics, through the use of functional health feeds from plants containing phenolic compounds (Barszcz et al., 2020; Nguimbou et al., 2014), and, among them, roots and tubers (Chandrasekara & Kumar, 2016). Phenolic compounds constitute a heterogeneous group of natural antioxidants that exert beneficial and protective actions on human and animal health (Baião et al., 2017; Shang et al., 2020). The antioxidant activity of phenolic compounds is especially due to their redox properties, since they allow them to act as reducing agents, hydrogen donors and oxygen extinguishers, potentially chelating metals (Rice-Evans et al., 1995). Ferrous ions are the most effective pro-oxidants in the food system and the high chelating capacities of ferrous ions are beneficial for health (Yamaguchi et al., 1988).
In swine production, several effects are reported: antimicrobial action, maintenance of beneficial intestinal microbiota, antioxidant, anti-inflammatory and growth promoting, stimulation of the secretion of digestive enzymes, improved intestinal health, palatability and taste of the feed (Gheisar & Kim, 2018; Guevarra et al., 2019; Yang et al., 2015).
The objective of this research was to evaluate the effect of inclusion of rejected taro tuber flour (RTTF) on productive performance in commercial hybrid pigs (Landrace × Duroc × Pietrain) during the post-weaning period.
Materials and methods
Location of the experiment
The study was carried out in the pig area of the Caicedo Agricultural Farm, located in the Tarqui area, Pastaza canton, Pastaza province, Ecuador. The animals were kept in a half-closed unit with natural ventilation and photoperiod during the months of September and October 2018. During this period, the maximum average daily temperature was 29 ± 1.6 °C and the minimum temperature 16.8 ± 1.9 °C.
Elaboration of the RTTF
The flour was made following the procedure of the Ecuadorian Technical Standard, Norma Técnica Ecuatoriana (Instituto Ecuatoriano de Normalización [INEN], 2006) numeration NTE INEN 616- 2006, corresponding to the preparation of vegetable flours. The rejected tubers were acquired in the rural area Teniente Hugo Ortiz, Allishungo community, and were conveyed to the premises of the “Granja Agropecuaria Caicedo”. Immediately, the tubers were washed with a 3 % sodium hypochlorite solution in water for 10 minutes, rinsed, and cooked for 20 min in a Memert water bath at 120 °C. Then, the water was drained and the tubers cooled for 30 min and chopped in slices. Subsequently the drying was performed with hot air recirculation at 65 °C for 8 h, and the milling was performed in an industrial mill (Talsa, Model W22, Spain) with a 0.25 mm mesh. Finally, 0.05 g ascorbic acid per kg dry matter was applied, and the tubers were vacuum packed in hermetic packages and stored until their use (Escobar et al., 2016).
Chemical characterization of the RTTF
Three 1 kg flour samples were randomly taken to analyze their chemical composition: dry matter (DM), crude protein (CP), crude fiber (CF), ether extract (EE), ash and nitrogen-free extracts (NFE) according to the procedures of the Association of Official Agricultural Chemists Official Methods of Analysis (AOAC, 2005). The content of neutral detergent fiber (NDF), acid detergent fiber (ADF) and lignin were analyzed according to Van Soest et al. (1991). Hemicellulose was calculated as NDFADF and cellulose as ADF-lignin. Gross energy (GE), digestible energy (DE) and metabolizable energy (ME) were carried out in a Parr adiabatic calorimetric pump (model 1241, USA).
Amino acid composition was carried out by high performance liquid chromatography (HPLC); the samples were processed by the method recommended by Gratzfeld-Huesgen (1998). An internal Norvaline standard at 20 pmol/μL was used to avoid systematic errors in the sample during the hydrolysis process. Tryptophan was determined according to the methodology described by Spies (1967).
The determination of minerals: calcium (Ca), phosphorus (P), potassium (K), sodium (Na), magnesium (Mg), iron (Fe), zinc (Zn), copper (Cu) and manganese (Mn) was carried out using atomic absorption spectrometry (Pérez et al., 2016).
Quantification of total phenols was performed by Folin-Ciocalteu’s spectrophotometric method (Magalhães et al., 2006). While for the total antioxidant capacity, the FRAP (Ferric Reducing Antioxidant Power) (Benzie and Strain 1996) and ABTS (2,2'-Azino-bis (3-ethylbenzothiazoline-6- sulfonic acid)) techniques were used (Re et al., 1999).
Animal handling
Sixty 30-day-old castrated male piglets from the commercial crossbreed (Landrace × Duroc × Pietrain) with an initial average live weight of 9.66 ± 0.29 kg were used. They were randomly housed in individual pens of 0.80 m x 1.0 m (0.80 m2 ) for 28 days. The pen was provided with a hopper-type feeder and a nipple waterer installed in a stable with 1.6 m high walls, a plastic floor and curtains to regulate the temperature of the unit. All procedures were approved by the Code of Ethics for research with domestic animals and wildlife of the Universidad Estatal Amazónica, under protocol 25-01/2017.
Feed handling
An alimentary scale was used according to the nutritional requirements of the pigs in the postweaning stage. The treatments consisted of 0, 10, 20 and 30 % inclusion of RTTF in the diet, respectively. The diets were formulated according to the requirements and stablished by the National Research Council (NRC, 2012) for the post-weaning stage (table 1). All animals had ad libitum access to food and water throughout the experimental period.
Table 1. Dietary composition of the basal diet (dry matter)
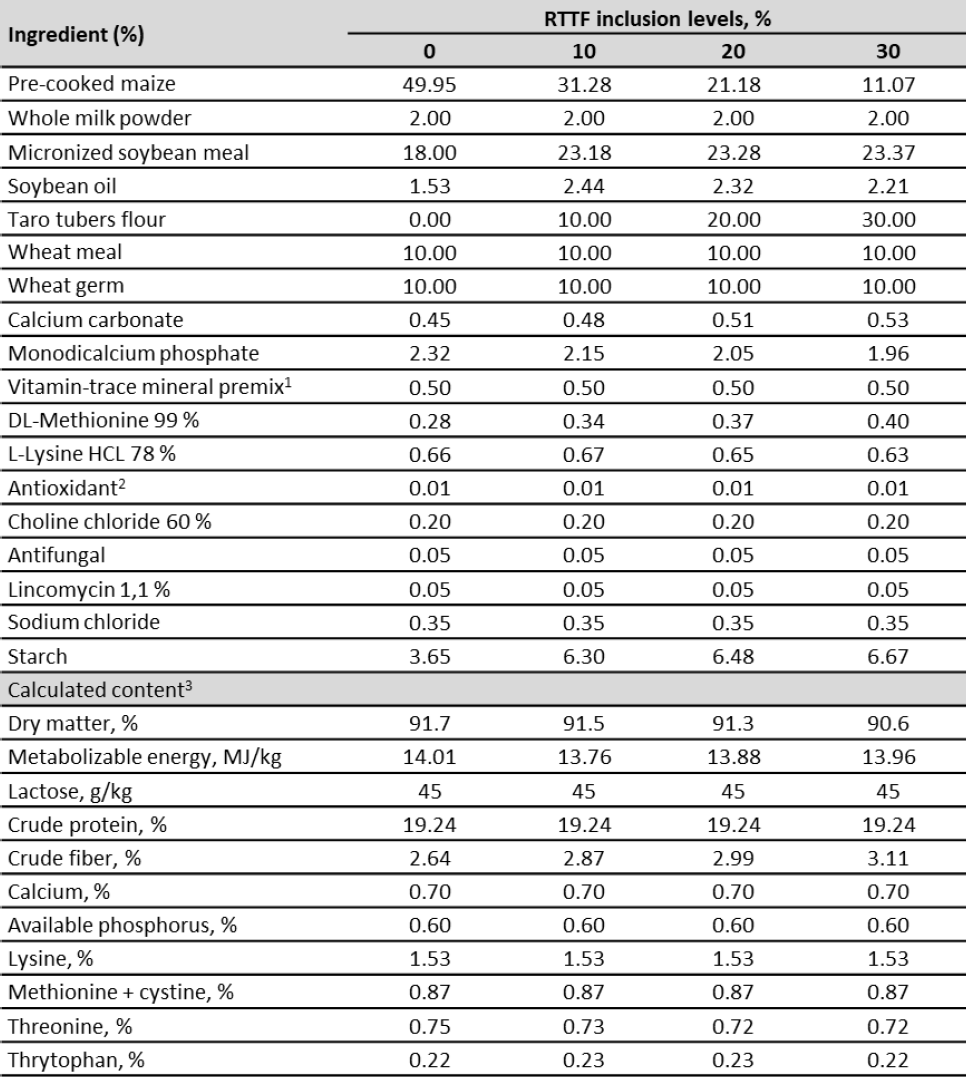
Note:1Supplied per kilogram of complete diet: 2,300,000 IU vitamin A; 4,666.67 IU vitamin D3; 5,000 IU vitamin E; 667 mg vitamin K; 333 mg vitamin B1; 1,000 mg vitamin B2; 400 mg vitamin B6; 4,000 µg vitamin B12; 4,000 mg calcium pantothenate; 67 mg folic acid; 6,660 mg niacin; 17 mg biotin; 183 mg cobalt (cobalt sulfate heptahydrate); 41.67 mg copper (copper sulfate pentahydrate); 267 mg iodine (potassium iodine); 26.67 mg iron (ferrous sulfate); 16.67 mg manganese (oxide manganese); 67 mg selenium (sodium selenite) and 16.67 mg zinc (zinc oxide); 2BHT; 3Calculated according to NRC (2012).
Source: Elaborated by the authors
Evaluation of the productive index and the production cost
The animals were weighed individually every 7 days on a 100 kg capacity Cardinal brand scale. We surveyed their average daily feed intake (ADFI), average daily gain (ADG), feed conversion (FC), body weight (BW), presence of diarrheas and mortality (Halas et al., 2010). To estimate the production cost, we took into account the direct (piglets, feed, materials, sanitary control, labor) and indirect costs (depreciation of the sheds, equipment, tools), administrative expenses (2% of the 10,000 dollars’ investment) and financial expenses (8% of 10,000 dollars in investment) (Brito et al., 2017).
Statistical análisis
The experiment was carried out using a completely randomized design with four treatments, 15 repetitions and an animal that constitutes the experimental unit. The assumptions of normality and variance homogeneity were examined using the Shapiro-Wilk and Levene tests, respectively. The results were statistically analyzed by ANOVA of repeated measures for related samples. For all the data, the linear and quadratic effects of the RTTF inclusion levels were studied using polynomial contrasts. A Chi square test was used for the statistical analysis of the diarrhea and mortality incidence values. The responses were considered significantly different when p < 0.05, and the results presented as mean ± standard error (SD). All statistical analyses were performed using R software (R Core Team, 2019).
Results
Chemical composition
The chemical composition of the RTTF can be seen in table 2. High levels of DM, NFE, GE, DE, ME, and moderate values of CP, CF, EE, ash, NDF, ADF, lignin, cellulose and hemicellulose were obtained. The contents of aspartic acid and glutamic acid were high; there were moderate levels of lysine, threonine, serine, proline, alanine, phenylalanine, glycine, leucine, isoleucine, valine, tyrosine and histidine; and low levels of methionine and tryptophan; there was no presence of cystine. A significant content of potassium, iron, zinc, calcium, phosphorus, magnesium, sodium, manganese and copper was obtained.
Table 2. Analyzed content of rejected taro tubers flour (RTTF) (dry matter)
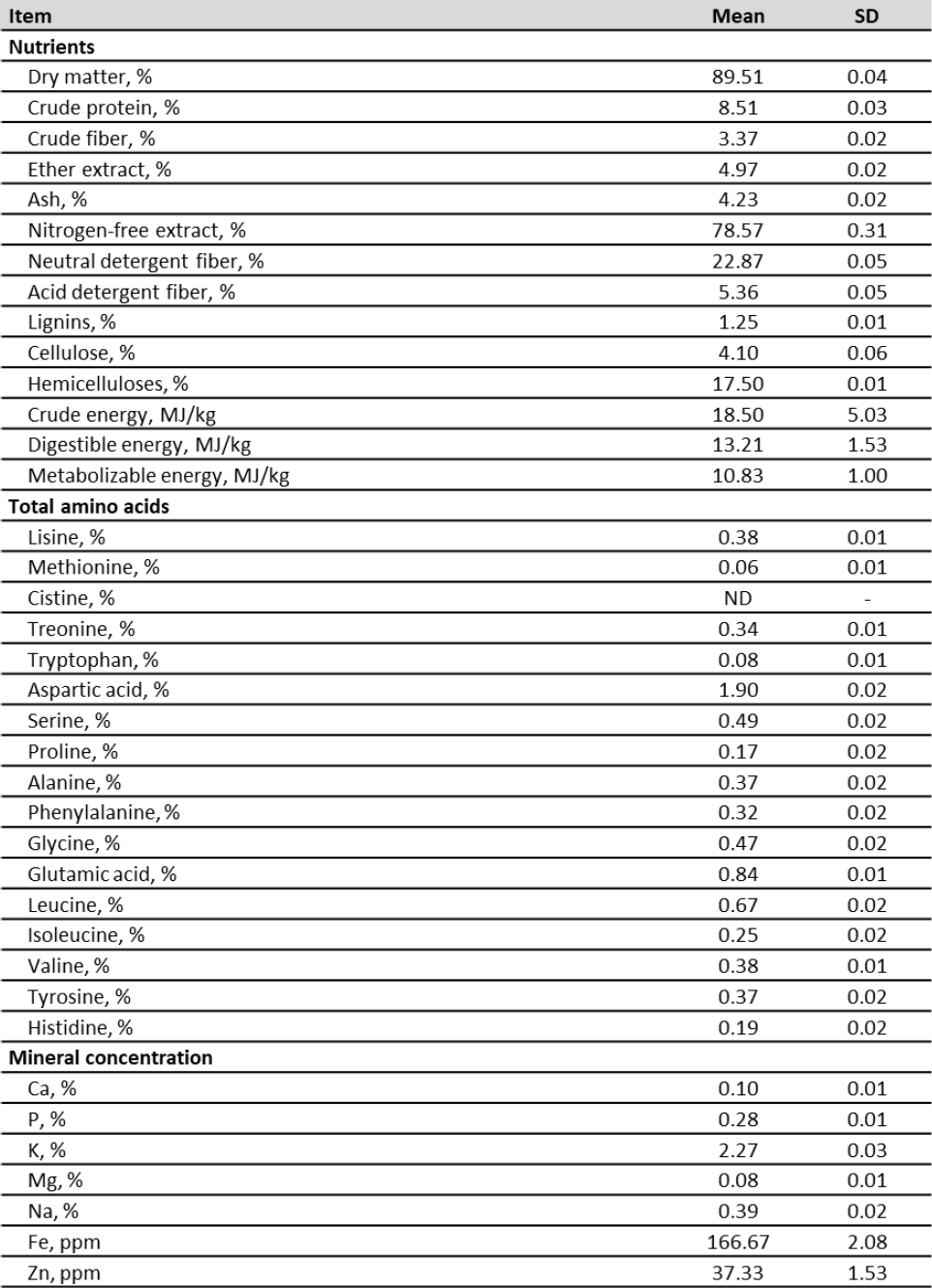
ND:Not detected
Source: Elaborated by the authors
RTTF showed a considerable content of total phenols; a moderate FRAP antioxidant activity; and a low ABTS antioxidant activity (figure 1).
Productive performance
The effects of the inclusion of RTTF on ADFI, ADG, FC, BW and production cost are shown in table 3. There was no presence of diarrhea or mortality during the study.
Table 3. Live body weight (BW), average daily gain (ADG), average daily feed intake (ADFI), feed conversion ratio (FC), diarrhea occurrences, mortality and production cost in post-weaning piglets supplemented with rejected taro tuber flour (RTTF)
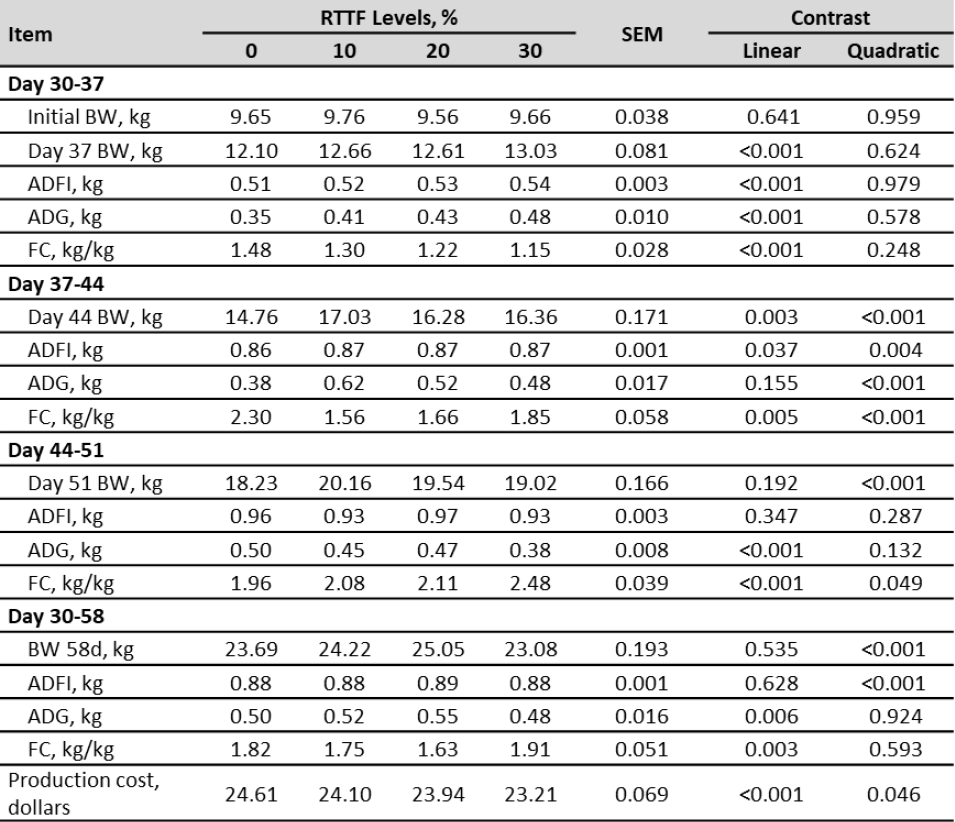
SEM:standard error mean.
Source: Elaborated by the authors
In this study there was no presence of diarrhea or animal deaths during the study. In the first period (30-37 d) the inclusion of RTTF produced a linear effect of the BW (p < 0.001), ADFI (p < 0.001), ADG (p < 0.001) and FC (p < 0.001). For the second period (37-44 d), a quadratic effect of the BW (p < 0.001), ADFI (p = 0.0 04), ADG (p < 0.001) and FC (p < 0.001) was obtained. In the third period (44 -51 d), a quadratic effect was verified for the BW (p < 0.001) and linear effect for ADG (p < 0.001) and FC (p < 0.001), there were no significant differences for the ADFI for which the groups had an mean consumption of 0.96, 0.93, 0.97 and 0.93 kg/d. For the complete period (30-58 d), a quadratic effect was obtained on BW (p < 0.001), ADFI (p < 0.001), and a linear one on ADG (p = 0.006), FC (p = 0.003) and production cost (p < 0.001).
Discussion
Chemical composition
RTTF showed high content of DM (89.56 %), NFE (78.57 %), GE (18.50 MJ/kg), DE (13.21 MJ/kg) and ME (10.83 MJ/kg), as can be seen in table 2. The high DM content is appropriate to preserve the product for prolonged periods (Caicedo et al., 2018). Moreover, levels higher than 12 % could induce moisture damage during storage of the flours, since it can cause growth of putrefactive microorganisms such as molds (Alternaria, Aspergillus, Cladosporium, Fusarium) and bacteria (coliforms, enterococci, E. coli) (Kaur et al., 2013; Salgado & Jiménez, 2012). These microorganisms cause deterioration of the product, and produce changes in its appearance and losses in its nutritional value, due to the degradation of proteins, lipids and carbohydrates; contamination with microbial toxins may also cause damage to human and animal health (Organización Panamericana de la Salud [OPS], 2020).
The high levels of NFE, GE, DE and ME in the RTTF allow us to classify this feed as a good alternative energy source, which can compete with traditional grains such as corn and wheat. For this reason, taro tubers are a staple in many developing countries due to their high energy inputs for food and feeds (Huang et al., 2007; Ogunlakin et al., 2012).
The RTTF showed moderate content of CP (8.51 %), ash (4.23 %), EE (4.97 %), CF (3.37 %), FDN (22.87 %), FDA (5.36 %), lignin (1.25 %), cellulose (4.10 %) and hemicellulose (17.50 %), respectively (table 2). These results are similar to those reported for taro varieties grown in Africa (Aboubakar et al., 2008; Ndabikunze et al., 2011; Nguimbou et al., 2014), Colombia (Púa et al., 2019), and Mexico (Madrigal-Ambriz et al., 2018). Moreover, it has to be noted that taro fiber is considered dietetic and is very advantageous for its active role in regulating the intestinal transit, increasing the diet’s volume and stool consistency due to its ability to absorb water (Temesgen & Ratta, 2015).
Regarding the amino acid composition of the RTTF (table 2), high contents of non-essential amino acids were determined; aspartic acid (1.90 %), glutamic acid (0.84 %) and low levels of sulfur amino acids; methionine (0.06 %) and absence of cystine. In African taro flour varieties, Njintang et al. (2014) and Temesgen et al. (2017) reported high values of nonessential amino acids (aspartic acid and glutamic acid) and low levels of methionine and absence of cystine, results similar to the ones obtained in this study. In this sense, Yeoh and Chew (1997) and Shewry (2003) report that the roots and tubers are deficient in sulfur amino acids.
The RTTF showed a high content of K (2.27 %), Fe (166.67 ppm), Zn (37.33 ppm), Ca (0.10 %), P (0.28 %), Mg (0.08 %), Na (0.39 %), Mn (18 ppm) and Cu (9.33 ppm) (table 2). The results obtained from this research are consistent with other studies that have reported that taro tubers have significant contents of K, P, Mg, Zn, Fe, Cu and Ca (Madrigal-Ambriz et al., 2018; Mergedus et al., 2015). Among the macro-minerals, K and P stand out, while Fe and Zn predominate among the micro-minerals.
The RTTF exhibited a significant content of total phenols (220 mg gallic acid/100 g DM); antioxidant activity moderated by FRAP (25 mg TROLOX/100 g DM); and low antioxidant activity with ABTS (11 mg TROLOX/100 g DM) (figure 1). Regarding phenolic compounds in taro flour, Arıcı et al. (2016) reported 120 mg gallic acid/100 g DM; Madrigal-Ambriz et al. (2018) obtained 113 gallic acid mg/100 g DM; and Purwandari et al. (2018) attained (79 mg gallic acid/100 g DM), lower figures than those found in this research. Regarding antioxidant activity, a better value was obtained with the FRAP technique; this is probably due to the fact that this foodstuff has an appreciable iron content (Mergedus et al., 2015) and therefore the affinity for ferric ions of the FRAP method.
Simsek and El (2015) studied the total phenolic and flavonoid composition of taro corm, and determined that flavonoids were present in about a quarter of the total phenolic content. The main flavonoids found in taro corm were quercetin and antoncyanins (Awa & Eleazu, 2015; Terasawa et al., 2007). In another study with liquid chromatography mass spectrometry, Kumar and Sharma (2017) determined that caffeic acid, gallic acid, chlorogenic acid, catechin, epicatechin 3, 5 DiCQ acid and flavanols were the main compounds present in taro flour extract.
The bioactive compounds of taro have antitumor, antimutagenic, immunomodulatory, antiinflammatory, antioxidant, antihyperglycemic and antihyperlipidemic activities. Ribeiro et al. (2021) reported that the bioactivity of taro tuber is attributed to the combination of tarin, taro polysaccharide-4-I, taro polysaccharides 1 and 2 (TPS-1 and TPS-2), A-1/B-2 α-amylase inhibitors, monogalactosyldiacylglycerols (MGDGs), digalactosyldiacylglycerols (DGDGs), polyphenols and non-phenolic antioxidants and provide anticancer and immunomodulatory benefits.
Productive performance
The literature on the evaluation and use of RTTF in post-weaning pigs’ diets is scarce. This study was carried out in adequate sanitary conditions, and the RTTF inclusion between 0 and 30 % presented high production levels for the BW (23.69-23.08 kg) and ADFI (1.19-1.19 kg/d), although a slight effect in ADG (0.78-0.58 kg/d) and FC (1.53-2.15 kg/kg) was verified. Nonetheless, with the increasing inclusion of RTTF there was a linear reduction in the feeding cost (24.61-23.21 dollars) for the entire period (30-58 d), as can be seen in table 3. In this regard, Aragadvay et al. (2016) found that the inclusion of taro meal for human consumption in the diet of post-weaning pigs up to 30% did not affect the BW (23.44-22.87 kg), with a slight detriment in the FC (1.39-1.48 kg/kg). Variation in ADG and FC for the full period (30-58 d) with the inclusion of RTTF in the diet could be explained largely by substituting ingredients of high nutritional value – such as corn and soybean meal (Satessa et al., 2020). These raw materials are irreplaceable in the diet of pigs due to their high energy and protein content of high biological value and digestibility (Florou-Paneri et al. 2014; Liu et al., 2019).
The linear reduction found for the production cost can be related to the use of a product of rejection that does not compete with conventional raw materials which are frequently used for human consumption. This element was demonstrated by Bauza et al. (2005) and Caicedo and Flores (2020) who used alternative ingredients in pigs after weaning and managed to reduce the production cost without negative effects on productive indicators or the animals’ health.
The optimal use of starch from RTTF is due to its structural form, amylose has a linear structure, whilst amylopectin is ramified (Vargas & Hernández, 2013). This favors the entry of water into intermolecular spaces, improving the solubility of the polymers (Araujo et al., 2004). In summary, when the starch granules hydrate, they cause increase in its size and its structure changes from a semicrystalline to an amorphous one – this process is known as gelatinization (Torres et al., 2013). This change of structure favors the use of starch due to the action of amylases generated in the salivary and pancreatic glands of pigs (Lapis et al., 2017). On the other hand, the combination of small granules and low content of highly soluble dietary fiber makes taro tubers an excellent source of carbohydrates (Vargas & Hernández, 2013).