Introduction
Diverse anthropic activities such as deforestation, mining, indiscriminate burning, crops, and livestock, among others, cause alterations in the soil’s physical, chemical and biological composition, modifying the landscape and its biodiversity (Casas, 2012). Of these, mining is one of the harshest activities due to the impact on the land surface. It causes deforestation, erosion, and deposits of mining waste, where heavy metals accumulate, producing toxicity in the soil, water sources, and nutrients and biodiversity loss (Gill, 2010).
Soil is one of the environments with the most considerable effect on ecosystem stability, as it is a complex system that supports thousands of organisms and their biological interactions. One of the most important is the relationship between plants and arbuscular mycorrhizal fungi (AMF), which are associated with 72 % of plants (Brundrett & Tedersoo, 2018). In this association, they act as plant establishment promoters that benefit the plant nutritionally and increase its tolerance to pathogens (Ramírez & Rodríguez, 2012). Moreover, they counteract soil compaction thanks to the secretion of glomalin, which acts as a binder of minerals and organic matter, i.e., a feature related to soil stability and structure (Genre et al., 2008; Grümberg et al., 2010).
For this reason, it is necessary to conduct studies that evaluate changes in the microbiota’s current state, vegetation cover, and physicochemical characteristics of soils degraded by mining activities. They allow comparing plant and microbiological communities according to their richness for aspects of their ecology, structural components, and responses at the plantenvironment level that allow plant and microbiological communities to be compared according to their richness for aspects of their ecology, structural components and responses at the plantenvironment level, and to apply these comparisons to the identification of organisms that can be used in programs or plans to restore degraded areas (Marrugo-Negrete et al., 2016).
The evaluation of the plant community and AMF has focused mainly on the study of native forests used as reference areas (Quiroga et al., 2019; Rodríguez-Rodríguez et al., 2021) or the effect of anthropic activities such as agroecosystems agroforestry, and livestock (HernándezAcosta et al., 2021; Restrepo et al., 2019; Sandoval-Pineda et al., 2020; Segura-Madrigal et al., 2020). Worldwide, studies report the effects of gold mining on biological communities, showing that it effectively decreases the diversity of vegetation and AMF (Takarina et al., 2021; Wei et al., 2015). However, few studies in Colombia have analyzed the responses of communities altered by mining, such as the work by Ramirez and Rangel-Ch (2019) that show changes in plant succession processes after gold, platinum, and silver open-pit mining extraction activities in the Choco rainforest. Medina et al. (2009) reported decreases in the abundance and diversity of AMF in areas altered by alluvial mining in the lower Antioqueño River basin.
Therefore, phytoremediation and mycoremediation arise from previous characterization processes in degraded areas and become sustainable alternatives to reduce this impact (Hakeem et al., 2015). The use of plants and fungi with the capacity to detoxify and stabilize contaminated environments, together with the accumulating effect of pioneer plants found in the areafurther enhanced with the association with AMF, is a strategy used worldwide (Audet & Charest, 2007; Hildebrandt et al., 2007; Schneider, Labory, et al., 2013).
Due to the importance of these alternatives in the recovery or stabilization of areas degraded by mining activities, this study aimed to evaluate the effect of mining on the physicochemical composition of affected soils and the abundance and diversity of vegetation and AMF in the municipality of Santa Isabel, province of Tolima (Colombia).
Materials and methods
Study area
The study was conducted on areas around gold mining locations in Santa Isabel, Tolima, Colombia, where gold has been extracted since 1990 through underground exploration and waste is deposited in terraces. The gold deposit exploration process involves the removal of natural vegetation and soil. The removed soil is fragmented and extracted with xanthates and dithiophosphates to release metallic elements, causing acid rain in the mine. Then, leaching with sodium cyanide occurs, recovering gold by adding zinc dust.
Three areas were evaluated at 1,945 meters above sea level. The first area comprises mining waste deposit terraces (SI-M) at 04°43′ N and 75°04′ W. The second is an intermediate area with mining waste and initial natural plant successions (SI-SV) located at 04°43′ N and 75°04′ W. The third is an adjacent area to the mining waste zone with native vegetation and no mining deposits (SI-N), classified as an Andean humid-montane low forest at 04°43′ N and 75°05′ W. It has an average temperature of 16 ° C, with an average annual rainfall of 1,900 mm (Segura-Madrigal et al., 2020).
The vegetation associated with the study area was evaluated in the SI-SV and SI-N areas but not in the SI-M area, as it did not have any vegetation. The characterization of physicochemical factors and AMF evaluation were only conducted for the SI-M and SI-N areas.
Plant characterization
Plants were characterized using the rapid area plot (RAP) methodology, proposed by Isa-Jaum (2004), with rectangular transects of 4 x 50 m for SI-N. In SI-SV, it was modified to 4 x 14m due to the low vegetation of the area. The vegetation was assessed by census and measuring the species present, selecting those with a diameter at breast height (dbh) ≥ 2.5 cm on the left side and all the plant species on the right side. The plant material collected was first pressed on newspaper and then preserved with 70 % alcohol, registering ephemeral characteristics (Ariza et al., 2009). Samples were determined by comparison with JSTOR images of type specimens (Global Plants, 2017), virtual herbaria in COL (Universidad Nacional de Colombia [UNAL], 2016), KEW (The Herbarium Catalog, Royal Botanical Gardens, 2017) and MO (Tropicos, 2017) and by confrontation with material deposited in the Universidad del Tolima TOLI Herbarium.
Sampling and soil analysis
The soil of each area was collected at four sample points, separated by 10 m in the form of an “x,” using a Pressler borer at a depth between 0 – 20 cm. Each point was composed of five subsamples homogenized and collected in plastic bags until analysis (Instituto Geográfico Agustín Codazzí [IGAC], 2014).
Physicochemical parameters
Physicochemical analyses (i.e., pH, organic matter, phosphorous, sulfur, Al+H, exchangeable bases, cation exchange capacity (CEC), minor elements, and electrical conductivity (EC)) of the areas under study (SI-M and SI-N) were carried out in the Agroanalysis Soil Laboratory. The total content of heavy metals (arsenic, cadmium, chromium, cobalt, lead, zinc, copper, vanadium, mercury, nickel, and selenium) was established by x-ray fluorescence in the ALPHA 1 SAS Laboratory. Furthermore, available heavy metals (arsenic, cadmium, chromium, mercury, and lead) were measured in AGROSAVIA analytical chemistry laboratory.
AMF spore isolation, quantification, and multiplication
The quantitative analysis of the AMF was made from 10 g of soil in each study area and point. Spores were obtained from 10 g of soil using the wet sieving method and sucrose gradient centrifugation. Evaluations were conducted from soil obtained directly during the collection and using trap crops. The latter consisted of making four replicates per sampling point from 150 g of soil, homogenizing it in soil and sterile sand 3:1 ratio. Then, it was deposited in pots where 20 seeds of Brachiaria decumbens were planted. After one month, only three plants were selected and maintained per pot. Plants were irrigated at field capacity and fertilized once a month with a solution of humic extracts with a low phosphorus content. After three months, plants were subjected to water stress and cutting off their leaves, and subsequently, after fifteen days, soil spores were extracted using the methodology published by Gerdemann and Nicolson (1963) with two centrifugations at 4,000 and 3,000 rpm for 5 and 10 minutes respectively (Sieverding, 1983; Usuga et al., 2008).
Morphologic identification of AMF
Spores were grouped according to their morphology. Each morphotype was fixed in polyvinyl alcohol-lactic acid-glycerol (PVLG) and PVLG + Melzer’s reagent, taking photographic records in a compound microscope (Primo Star with a 0.5x adapter and an Axiocam coupled camera Erc 5s with a 5-megapixel Zen program). Finally, a comparison was made with the morphological descriptions reported by Blaszkowsky (2003), Schüßler (2013), Oehl et al. (2011), and International Culture Collection of (Vesicular) Arbuscular Mycorrhizal Fungi (INVAM) (University of Kansas, 2017) to complete their identification.
Diversity indices and statistical analysis
AMF and vegetation diversity and abundance were evaluated using adequate numbers proposed by Hill (1973) and the Shannon-Wiener Diversity Index and Simpson's Dominance Index.
Physicochemical soil properties and AMF abundance were analyzed using the Shapiro-Wilk normality test (data of total spore abundance were log (x + 1) transformed prior to analysis to meet requirements for normality and homogeneity of variance). These results were then used to perform an analysis of variance (ANOVA) using the Tukey or Kruskal Wallis comparison methods, depending on whether the data were parametric or non-parametric. The multivariate analysis of principal components and the canonical correlation test between physicochemical soil variables and the diversity of AMF species were used. Tests were carried out using the statistical program CANOCO 4.5 for Windows and Past.
Results and discussion
Floristic abundance and richness
The study registered 605 individuals belonging to 29 families, 46 genera, and 54 species (Table 1). The most abundant plant families in the study areas were Poaceae with 231 individuals (four species), i.e., 38 % of the total percentage of families, Asteraceae with 95 (eight species), Melastomataceae with 38 (eight species), and Solanaceae with 15 (four species). These comprised 24 % of the total percentage of families found in the study, and the remaining 38 % corresponds to the remaining 24 families found. Moreover, diversity (Shannon) and dominance (Simpson) indices show that the floristic composition of the zones analyzed was homogeneous. Nevertheless, the conserved area showed a higher species diversity than the intermediate area.
Table 1 Diversity indices and Hill numbers SIM mining deposit areas SISV area with natural plant succession SIN area without mining intervention TC trap crops
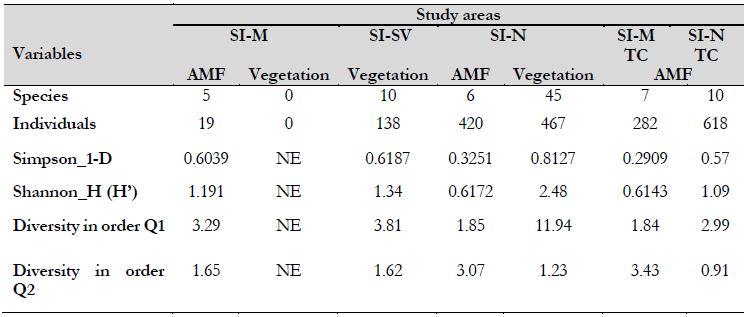
Note.NE: Not evaluated
Source: Elaborated by the authors
The SI-SV area shows a diversity equal to a theoretical community with 3.8 genera. On the other hand, a diversity equal to a community with 11.9 effective genera was found in the conserved area. We can conclude that the conserved area is 8.12 times more diverse when expressing this equivalence.
Our study recognizes the typical floristic richness of the Colombian Andean region in zones surrounding degraded areas due to gold mining activities. Fifty-four plant species were reported together for the SI-N and the SI-SV areas. The abundance and diversity of the floristic composition highlight the Melastomataceae, Asteraceae, and Solanaceae families as the most abundant in the SI-N zone, agreeing with the species diversity and dominance registered for the premontane Andean range (Alvear et al., 2010). The genus Miconia (Melastomataceae) was the most abundant and diverse in these families. It is, however, congruent since this genus is considered the largest in the number of woody plants with flowers, with around 1,050 species described and concentrated throughout the Andes (Goldenberg et al., 2013; Roa-García & Torres-Gonzáles, 2021).
The most predominant species found in our study was the oak tree Quercus humboldtii Bonpl. [Fagaceae] (Lozano & Torres, 1974) because it represents 6 % of the total percentage of species found in the SI-N area with 34 individuals. It can be related to its wide distribution (from 1,000 to 3,600 meters above sea level); however, it is more restricted to the high and humid parts of the Andes (Alvear et al., 2010; Corporación Autónoma Regional del Tolima [CORTOLIMA], 2009; Nieto & Joker, 2000; Segura-Madrigal et al., 2020). It is possible that when observing species with high associative and dominant tendencies as Q. humboldtii, the floristic composition of the zones analyzed was homogeneous since this has been previously reported In forests with similar altitude and precipitation conditions (Alvear et al., 2010; CORTOLIMA, 2009; SeguraMadrigal et al., 2020).
Besides characterizing the vegetation typical of the area, the floristic composition of an area with mining sludge and native soil (SI-SV) is recognized, noting a primary vegetation type very common in recovery areas, with the presence of the families Asteraceae and Poaceae (Ramirez & Rangel-Ch., 2019). These have a high capacity to settle in open places with degrees of anthropic intervention, such as mining sites and livestock pasture areas, among others. Thanks to their anemochory dispersion strategy, they travel long distances and colonize uncovered or bare soils (Arias & Barrera, 2007; Ranjeet et al., 2010). Within these families, the predominant genera were Baccharis (Asteraceae), Paspalum, and Panicum (Poaceae).
The use of pioneer plants with detoxification capacity and stabilization of contaminated environments, enhanced with the association of AMF, is a strategy used globally (Audet & Charest, 2007; Hildebrandt et al., 2007; Schneider, Labory, et al., 2013). In this study, Baccharis stands out as a potential species in the recovery of degraded areas. It is pioneer and abundant, a condition that favors the appearance of propagules of other native plant species (Sarmiento, 2008), establishes in previous stages, and indicates an advance in the plant succession process because it strengthens the first millimeters of the soil profile, which is critical in erosion control (Acuerdo municipal No. 006, 2012; Rangel et al., 2011; Sarmiento, 2008). Besides, Baccharis sp. can grow in soils contaminated with heavy metals, together with its potential in the phytoextraction of lead (Pb) and zinc (Zn) (from 2,120 to 3,060 mg/kg of Pb and from 1,090 to 1,490 mg/kg of Zn) making it an important genus in management plans for degraded areas due to mining activity (Afonso et al., 2021; Bech et al., 2012).
Physicochemical soil properties
The soil in the SI-M and SI-N areas was classified as a sandy loam. The parameters that showed significant differences (p < 0.05) were bulk density, Na, Ca, Mg, Al+H, Zn, Cu, Fe, and Mn. Regarding the heavy metals As, Cd, Cr, Co, Cu, Ni, Pb, Vn, and Zn, the similarity analysis showed that most showed significant differences between the study areas, except for Ni and Cr (Table 2). The principal component analysis (PCA) showed that 69.5 % of the data was explained by PC1 and 24.3 % by PC2, totaling 93.4 % of the total variability from the physicochemical attributes. The variables that mainly contributed to the segregation of components were electrical conductivity (mS/cm), pH, percentage of organic matter, and Fe, S, P, and Mn content. Regarding the PCA performed on heavy metals, PC1 represented 83.9 % of the total variability and only 10.3 % for PC2. Hence, PC1 and PC2 contributed 94.2 % of the variability, whereas all heavy metals contributed directly to the total variance.
The canonical correlation analysis indicated that AMF is related to the SI-N area, and the heavy metals are associated with the SI-M area. Furthermore, the number of spores found had a positive relationship with organic matter and the minor elements Fe, Mn, Cu, and S. The opposite case was found for heavy metals that have a negative correlation with the number of spores, especially zinc, lead, vanadium, and chromium; moreover, the latter also showed high values in the SI-N area (Figure 1).
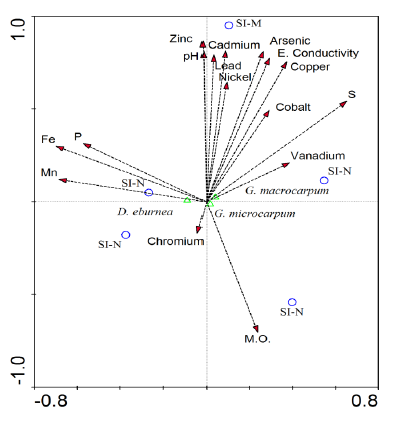
Figure 1 Canonical correspondence analysis of the physicochemical variables associated with the study areas (SI-N, SI-M) and AMF abundance
Table 2 Physicochemical characteristics of the area with mining deposits (SI-M) and the area without mining intervention (SI-N)
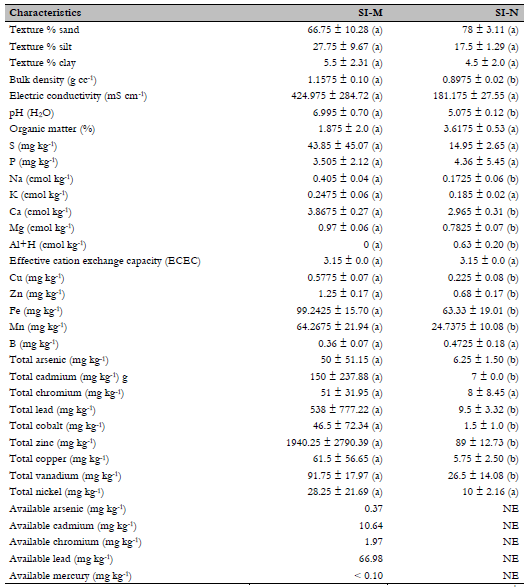
Note.(a) and (b): Average n = 4. Parameters with the same letters show no significant differences. Data are means ± the standard deviation with a confidence interval of 95 %. NE: Not evaluated. Total heavy metal values were measured using X-ray fluorescence, and available heavy metal values using inductively coupled plasma emission spectrophotometry.
Source: Elaborated by the authors.
Heavy metals and vegetation were influential factors in the diversity and abundance of AMF. An example is an SI-M area, with an abrupt decrease in plant species and high total concentrations of As, Ni, Zn, Cd, V, and Cr. It reduces fungi diversity and abundance due to the inability to develop their obligatory symbiotic association, and decreases spore viability (Schneider, Labory, et al., 2013). Two of the heavy metals with the most negative influence on richness indices in mycorrhizal communities are Cr, which is only related to the genus Gigaspora in tree samples found in soils contaminated by this metal (Khan, 2001), and V, which reduces mycorrhizal colonization by up to 73 % on average. However, despite its toxicity, mycorrhizal communities can settle in soils contaminated by this metal, which does not affect symbiosis (RodríguezRodríguez et al., 2021; Vidal, 2012).
The increase of spores in the SI-N area may be related to high organic matter (OM) values since it has a positive correlation with genera such as Acaulospora, Entrophospora, and Scutellospora (Perez & Vertel, 2010; Serralde & Ramírez, 2004; Willis et al., 2016). This relationship can be associated with the positive influence that OM has on CEC, soil structure, fertility, water retention capacity, porosity, diversity, and microbial activity. In turn, these microorganisms counteract soil compaction and increase OM levels, as reflected in the SI-N area, in which low bulk density levels and a high abundance of microorganisms are found (Ramírez et al., 2019; RodríguezRodríguez et al., 2021; Rojas, 2013; Salamanca & Sadeghian, 2005).
The values obtained from the minor elements Fe, Mn, Cu, and S also show a possible effect on the composition of the AMF communities in Andean soils. Influence is reported especially for Glomus species and those belonging to Diversisporales order in dry seasons, increasing abundance (Ramírez et al., 2019); in this study, the species D. eburnea showed the highest correlation to Fe and Mn.
AMF richness and abundance
The AMF count from direct soil samples showed that the spores in the SI-N area are 22 times more abundant (420/19) than those in the SI-M area. Furthermore, it was 2.1 times (618/282) more abundant when the count was obtained from trap crops. These increased the AMF richness for the SI-M (282 spores and seven species) and the SI-N areas (618 spores and ten species). Thus, from soil samples and trap crops, eight different morphospecies were registered in SI-M and ten in SI-N, corresponding to five families, seven genera, and 11 species (Table 3).
Glomeraceae showed the highest number of morphospecies with four, followed by Diversisporaceae with three. Acaulosporaceae and Archaeosporaceae had one morphospecie. Diversity indices demonstrate that the SI-N area is the richest in AMF species, with ten species and a higher dominance than the SI-M area, which exhibited three species with similar abundance (Table 1). Nonetheless, the PCA performed on the species matrix pointed out that the first axis or component explains 98.5 % of the total variance. Further, the species with the highest dispersion are Glomus macrocarpum, Glomus microcarpum, and Diversispora spurca.
Table 3 Diversity and abundance of AMF SI-M (mining deposit areas SI-SV area with natural plant succession SI-N area without mining intervention)
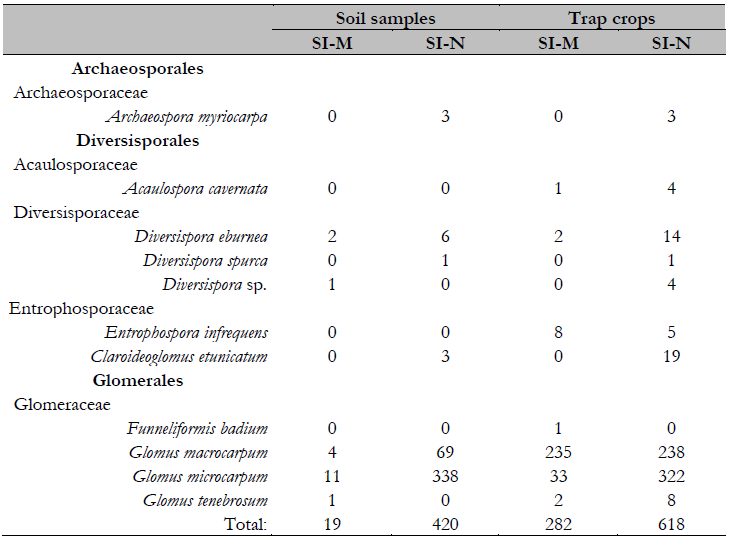
Source: Elaborated by the authors
Additionally, the species with the highest frequency of occurrence in soil samples from both areas was G. microcarpum (SI-M: 52.4 %, SI-N: 65.9 %). In trap crops, this same species was the most frequent for SI-N (47.4 %); however, G. macrocarpum was the one with the highest frequency of occurrence in the SI-M area (76.5 %), and G. microcarpum in the SI-N area (47.4 %).
Regarding AMF abundance and diversity, a significant increase was observed in trap crops compared with direct counting of soil samples, and as expected, a higher number of spores and species in the native area (SI-N) compared to the impacted area (SI-M). This evidence shows the mining waste’s apparent effect on the AMF spores.
Glomus was the most representative due to its abundance in Andean areas degraded by gold mining and wide distribution and tolerance to adverse conditions, ratifying its adaptability to this environment. This finding agrees with reports of its high prevalence in soils contaminated with arsenic and other heavy metals (Pulungan & Nasution, 2021; Rodríguez-Rodríguez et al., 2021; Schneider, Stürmer, et al., 2013), high drought levels (León, 2015), acid pH (Serralde & Ramírez, 2004), low OM content, among others (Cavalcanti et al., 2014; Rodríguez-Rodríguez et al., 2021; Schneider, Stürmer, et al., 2013; Wei et al., 2015).
Different authors also point out that species of Glomeraceae are generalists due to their high sporulation capacity and their ability to quickly adapt to degraded soils (Cavalcanti et al., 2014; Guo et al., 2020; Pulungan & Nasution, 2021; Ramos et al., 2014), besides being found in several regions of Colombia, including Tolima (Aranguen, 2015; Monroy et al., 2013; Peña et al., 2006). Glomus tenebrosum stands out for its presence in trap crops in both areas, finding only one spore directly in the soil. It is possibly due to its sensitivity to adverse mine tailing conditions since this species has been reported mainly in trap crops in mining degraded areas (Selvam & Mahadevan, 2002). In Colombia, Salamanca and Silva (1998) found this species directly in the soil of the municipality of San José del Guaviare but without any degree of alteration.
Entrophospora infrequens and Acaulospora cavernata were only found in the trap crops of the two areas evaluated. Entrophospora infrequens, G. macrocarpum, and G. microcarpum have been observed directly in degraded areas due to limestone mining (Texeira-Rios et al., 2013). In Colombia, it has been found in Llanos Orientales (eastern plains), both in citrus crops and in two varieties of corn (Monroy et al., 2013; Serralde & Ramírez, 2004). A. cavernata has been recorded worldwide in Thuja occidentalis agricultural and forest parcels (Bárcenas-Ortega et al., 2011; Ramos et al., 2014; Souza, 2015). However, it had not been associated with areas degraded by mining activities.
Our study is a starting point in consolidating management and recovery plans for mining areas. It allows knowing, for the first time, the vegetation and the AMF community associated with this type of area, which will be essential in subsequent phytoremediation projects. Considering the importance of AMF for the balance of host plants, the possibility of selecting species found in an affected area that can be used in management programs to recover degraded zones or similar areas is opened. Natural ecosystems, such as SI-N, are sources of various populations of microorganisms, including AMF. They can improve the quality of restoration by reintroducing key organisms from the microbiome of native plants enhancing plant diversity, accelerating succession, and increasing plant establishment that is frequently lost in recovery (Koziol, 2018).
For this reason, it is vital to know the native microbiota of plants in natural ecosystems or that altered by anthropic activities through diversity studies, which have brought benefits such as the discovery of species remarkably adapted to various environmental conditions (Aguilera et al., 2014; Estrada et al., 2011; Teixeira-Rios et al., 2013).
The preceding is added to the fact that mining in the last 15 years has doubled in Colombia, thus increasing the degraded land (Ospina-Correa et al., 2021). Therefore, mining waste management is required in affected areas. For example, the province of Cesar employs biochar and AMF to recover soil affected by carbon mining, improving soil properties and stimulating growth of seedlings (Quiroz-Mojica et al., 2021).
Conclusions
This study found that the SI-M area is contaminated with heavy metals such as As, Cd, Co, Pb, Zn, Cu, and Vn. Further, the physicochemical parameters mainly altered are pH, Na, Ca, Mg, Al+H, Zn, Fe, and Mn compared to the SI-N area. The floristic composition of the zones analyzed was homogeneous. The highest diversity of species was found in the SI-N area, noting a reduction of the vegetal cover due to mining tailings in the SI-SV area. Bulk density, alkaline pH, and a decrease in OM conditions, together with the concentration of the heavy metals Cr and Vn, considerably affected the abundance of AMF in the SI-M area. Moreover, our study highlights plant species of Baccharis (Asteraceae), Agrostis (Poaceae), and Miconia (Melastomataceae), together with the AMF Glomus microcarpum andG. macrocarpum for showing potential in phytoremediation processes.
Contribution of the authors
Herik Johan Guzmán Lasso: Elaboration of a project for access to economic resources, design of methodologies, registration of information in the field, construction of databases, analysis of information and preparation of the manuscript; Yessica Lorena Perdomo Useche: Elaboration of a project for access to economic resources, design of methodologies, registration of information in the field, construction of databases, analysis of information and preparation of the manuscript; Hilda Rocio Mosquera Mosquera: Taxonomic identification of specimens, design of methodologies, analysis of information and preparation of the manuscript; Urley Adrian Perez Moncada: Taxonomic identification of specimens, analysis of information and preparation of the manuscript; Maryeimy Varón López: Elaboration of a project for access to economic resources, design of methodologies, registration of information in the field, activities supervisión, analysis of information and preparation of the manuscript.