INTRODUCTION
Invasions of exotic species have become a big threat to marine systems worldwide (Mooney and Cleland, 2001) Muñoz. The lionfish (Pterois volitans) is one of the invading species with more negative impacts, given that it modifies the structural and functional organization of the ecosystems it invades, competing with mesopredator native species for food resources and space (Rojas-Vélez et al., 2019), and causing a decrease in the biodiversity of marine ecosystems due to the predation of native species (Albins and Hixon, 2008; Muñoz et al., 2011; Acero P. et al., 2019), especially in coral reefs (Arias-González et al., 2011; Acero P. et al., 2019). The lionfish’s success is due to its fast growth, high reproduction and feed rates, and the absence of natural predators (Côté et al., 2013; Tornabene and Baldwin, 2017; Acero P. et al., 2019; Bustos-Montes et al., 2020).
Lionfish have effective hunting strategies (Green et al., 2011; Albins, 2015; Tornabene and Baldwin, 2017), which generates a negative effect on recruitment and, therefore, on the population density and structure of the reef native fish community (Albins and Hixon, 2008; Albins, 2015). It has been found through stable isotopes (δ15N and δ13C) that it is a generalist predator feeding on hundreds of species (Acero P. et al., 2019; Eddy et al., 2020). Moreover, at different life stages and depths, it adapts to changes in the prey community (Muñoz et al., 2011; Pimiento et al., 2013; Eddy et al., 2020).
Lionfish have widely spread throughout the western Atlantic, the Caribbean, Gulf of Mexico (Schofield, 2010), even reaching the Brazilian province (Ferreira et al., 2015; Luiz et al., 2021). It was first observed in Florida in 1985, and, since 2000, it started an unprecedented expansion in speed and magnitude in the American Atlantic (Whitfield et al., 2002; Schofield, 2009, 2010). In Colombia, it was observed in the Providence island in 2008 (Schofield, 2009), and a year later in the continental Caribbean (González et al., 2009). The high richness of prey consumed by this species in the Colombian Caribbean evinces that it has a potentially negative effect, thus constituting a local extinction threat for some species of commercial and ecological importance (Acero P. et al., 2019).
Lionfish have been studied in shallow coral reefs in the Caribbean, but there is little knowledge about their biology and ecology in mesophotic reef ecosystems (MRE). Andradi-Brown (2019) suggests that the invasion of MRE can be even more harmful, significantly reducing fish abundance and diversity, for it reaches a higher size which requires more resources. Studies on this species at different depth levels have evidenced a vertical distribution in the Western Caribbean between 0 and 304 m (Andradi-Brown et al., 2017; Andradi-Brown, 2019).
In Colombia, the first record in a mesophotic environment was in 2014 in the underwater mountain Bajo Frijol located in the Corales de Profundidad Natural National Park (CPNNP) (Sánchez et al., 2016). The maximum depth of observation was 140 m, and all the specimens were larger than 10 cm in size (Henao-Castro et al., 2016). Given the marked expansion of lionfish and their potential negative effects on the reef ecosystems, it is necessary to broaden the knowledge of their biology and ecology in a deep environment. This work aims at describing the distribution, size, diet, and reproduction of lionfish in mesophotic environments of CPNNP, as input for adopting management actions in the protected area that help mitigate their effect.
STUDY AREA
The Corales de Profundidad Natural National Park is a protected, fully submerged area of 142,192.15 ha and located in the Colombian Caribbean (9° 43’ 16.6”-10° 07’ 30.3” N and 76° 0’ 16.2”-17’ 41.1” W), 32 km away from the closest point of the continent (Figure 1). Its depth ranges from 32 to 1240 m. As a feature, three general oceanographic conditions are dominant over it: the surface Caribbean current down to an approximate depth of 160 m; the Darién countercurrent with varying intensities according to depth; and the Caribbean deep current, a slow eastwards movement over the abyssal slope and bottom (Marrugo and Martínez, 2016; Morales-Giraldo et al., 2017).
The area is subjected to the trade winds regime and the Intertropical Convergence Zone, which contributes to three climatic seasons: (a) dry (December-April), with the displacement of water masses towards the southwest; (b) rainy (September-November), with trade winds weakening and predominant winds from the southeast or southwest; and (c) transition (May-August), with high temperature and low-intensity rainfall (Pujos et al., 1986; Andrade-Amaya, 2001). In the Park’s northeastern sector is Bajo Frijol or Frijolito, an underwater mountain with an elevation of 116.0 m, 8.2 km long, and 1.4 km wide (Morales-Giraldo et al., 2017). It is characterized by an only mesophotic environment, with 13 species of Scleractinia, high biomass of reef fish, shark presence, and species of commercial interest belonging to the Lutjanidae and Serranidae families (Marrugo and Martínez, 2016; Sánchez et al., 2016).
MATERIALS AND METHODS
Field sampling
Twelve stations were sampled between 35 and 160 m (Figure 1). Lionfish were caught in CPNNP’s monitoring campaigns and extraction days covering the three MRC strata (Kahng et al., 2010; Rocha et al., 2018): upper mesophotic area (UMZ: 30-60 m), middle mesophotic area (MMZ: 61-90 m), and lower mesophotic area (LMZ: ˃ 90 m). The specimens were caught with Hawaiian-type harpoons during closed-circuit rebreather dives (rebreather); dorsal, anal, and pelvic spines were removed with scissors; and they were kept in a fish trap. While in the vessel, they were stored in thermal bags in a cool box with ice. The characteristics of the bottom were recorded, and lionfish were counted, which were shot with a remote-operated or a drifting camera.
Laboratory procedure
Fish were codified, and the total length (Tl) and total weight (Tw) were recorded. A longitudinal cut was made in the belly area in the posterior-anterior direction, and the digestive tract was extracted and then weighed on an analytical scale (+ 0.0001 g). The content was poured into a strainer, and the obtained material was washed with seawater to eliminate the gastric acids and stored in labeled plastic jars. Preys were separated by taxonomic groups for their identification, preserving them in 70 % ethanol. When possible, they were measured (Tl) and individually weighted. Deteriorated or torn preys were classified under general items as crustaceans, fish, vertebrae, bones, and scale remains. The highly digested material was categorized as non-identifiable organic material.
For the isotopic analysis of each specimen, ≈ 1 cm3 of muscle was extracted and stored in a vial then put in an oven at 60° C for 48 h to desiccate the samples. To extract the lipids, each sample was placed in 25 mL of petroleum ether, stirred with a sonicator for 15 min, and finally centrifuged three times at 2200 rpm at a low temperature. Later, they were washed three times with deionized water and placed back in the sonicator for 10 min to eliminate urea (Kim and Koch, 2012). Each sample was macerated in a glass mortar to homogenize the tissue. A subsample (0.6-1.0 mg) was obtained, weighted on an analytical scale (+ 0.0001 g), and stored in a tin capsule (8 × 5 mm). The carbon and hydrogen isotopes were determined on a Carlo Erba NA 1500 NC elemental analyzer with a ConFlo III interface connected to a Delta Plus XP (EA-IRMS; ThermoQuest) mass spectrometer in the Andalusian Earth Sciences Institute in Granada, Spain.
Both gonads and liver were extracted and weighted, and the eviscerated fish were weighted. A fraction of the tissue from the middle part of the two gonads was fixed in 4 % formalin and later subjected to dehydration, impregnation, penetration, cutting, and staining techniques in successive Harris hematoxylin-eosin solutions (Martoja and Martoja-Pierson, 1970). Sex was macroscopically determined, and females were assigned to their development stage (Brown-Peterson et al., 2011). Considering that the species is an asynchronous partial spawner (Morris et al., 2011; Fogg et al., 2017; Eddy et al., 2019), the assigned reproductive stage was defined by the most advanced maturity stage observed in oocytes. Males were categorized as mature and immature.
Data analysis
Measures of central tendency were calculated (arithmetic mean) and dispersion (standard error, coefficient of variation, maximum and minimum values). The data fitting to the normal distribution was assessed through the Shapiro-Wilk test, and the variance homogeneity through the Leven test (Zar, 2010). The size and weight differences between sexes were assessed through the Mann-Whitney test (not normal data but with variance homogeneity), and the carbon isotopic signals (δ13C) and nitrogen (δ 15N) through the t-Student test, given that the normality and homogeneity assumptions on the variances were met (Zar, 2010; McDonald, 2014).
The food composition of this species was described using numerical -% N-, gravimetric -% P-, and frequency indices -% F- (Hyslop, 1980). To confirm the importance of each food component, the relative importance index -RII- (Pinkas et al., 1971) was used:
To determine whether lionfish are specialist or generalist predators, the Levin standardized index was applied (Krebs, 1999):
This index fluctuates between 0 and 1. Values lower than 0.6 indicate that the species tend tends to be specialist, and higher than 0.6 imply a generalist species (Labropoulou and Eleftheriou, 1997).
The values of the stable isotopes (δ) were calculated through the formula
with R sample being the proportion between the isotopes 15N/14N for δ15N and the proportion of the isotopes 13C/12C for δ13C (Park and Epstein, 1961). Using the SIBER (Stable Isotope Bayesian Ellipses in R) function, the isotopic amplitude of lionfish by sex was determined through standardized ellipses (Jackson et al., 2011). The estimation of isotopic overlap was estimated using the nicheROVER function in R (Lysy et al., 2015), which is based on the Bayesian method that calculates the overlap probability using multidimensional information as niche indicators. This function was calculated with 104 iterations and 95 % data probability in each group (i.e., males vs. females, and females vs. males) found in the isotopic space.
For each sex the weight-size ratio was determined by adjusting data to the model:
where Pt is the total weight, Lt total length, a is the intercept, and b is the growth coefficient (Csirke, 1989). A significant difference of the growth coefficient from 3 was assessed with a t-Student test (Pauly, 1984), considering the growth as isometric if b is not significantly different from 3, and allometric otherwise.
As indicators of the physical conditions of the fish, the gonadosomatic (IGS) and hepatosomatic (IHS) indices and Fulton’s condition factor (CF) were calculated, which were expressed as percentages (Lloret et al., 2014):
where Pt is the total weight (g), Lt the total length (cm), Pg the wet weight of the gonad (g), Pe the eviscerated weight (g), and Ph the liver wet weight (g). These indices were analyzed by sex and gonadal maturity stage.
RESULTS
Abundance and characteristics of bottoms
Two hundred thirty-seven (237) lionfish specimens were observed between 35 and 160 m depth, 228 were found in the UMZ, mainly with the closed-circuit dive method. In MMZ and LMZ, four and five individuals were seen, respectively. Six were observed with ROV, two with a drift camera, and one was initially caught in a fish trap.
The depth strata showed bottoms with different types of reliefs, generally with aggregations of juvenile fish. In the UMZ, large barrel sponges were observed, hard bottoms covered by algae, and rocky knolls with coral presence (BF1-BF6 and SC4). In the MMZ, big rocks with associated fauna were found, (SC1 and SC11). The LMZ was characterized by soft bottoms with dispersed fauna, (SC4, SC7, and SC8), with medium or small rocks (Figure 2; Table 1).
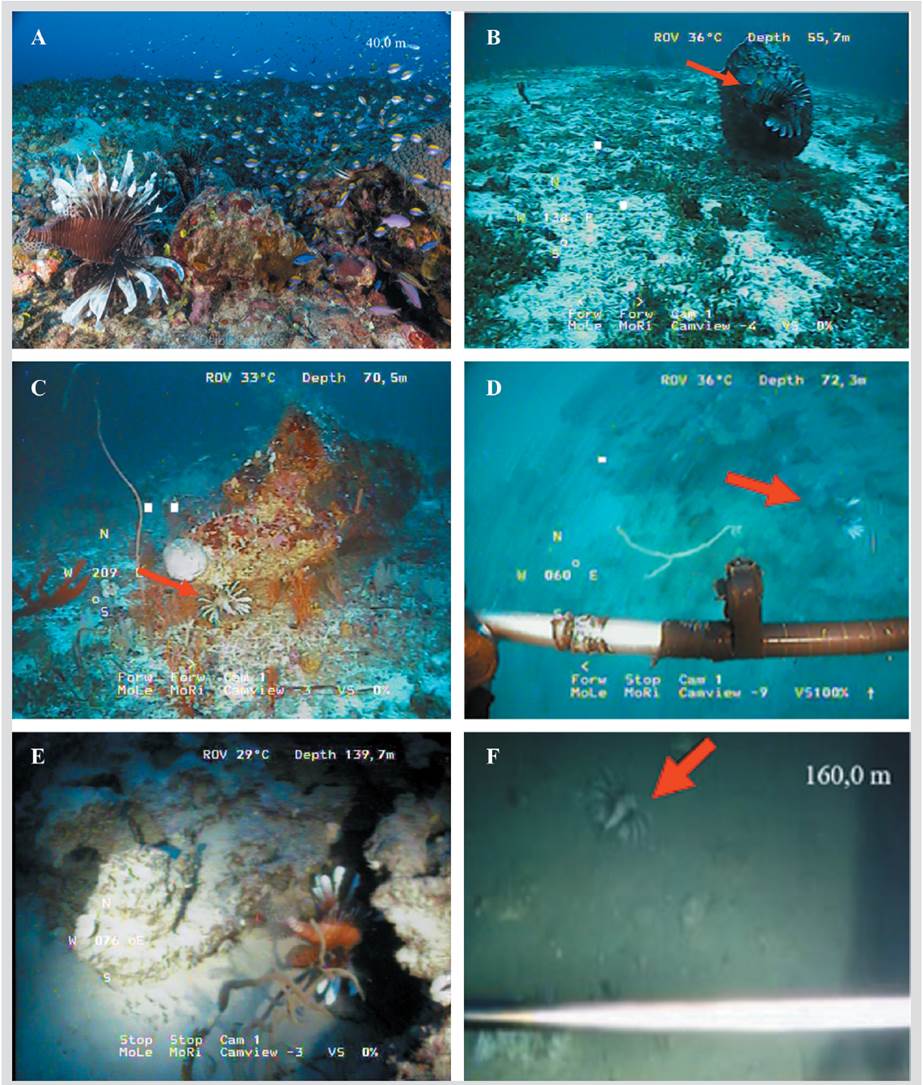
Figure 2 Sites where lionfish were observed in the CPNNP. UMZ (a and b), MMZ (c and d), and LMZ (e and f). Photo a by Deibis Seguro; photos b, c, d, and e by Cedeño-Posso et al. (2015); photo f by Cedeño-Posso et al. (2017).
Table 1 Characteristics of the sampled stations and lionfish abundance. UMZ: upper mesophotic zone. MMZ: medium mesophotic zone. LMZ: lower mesophotic zone. BF: Bajo Frijol. ZP: fishing area. SC: CPNNP sector.
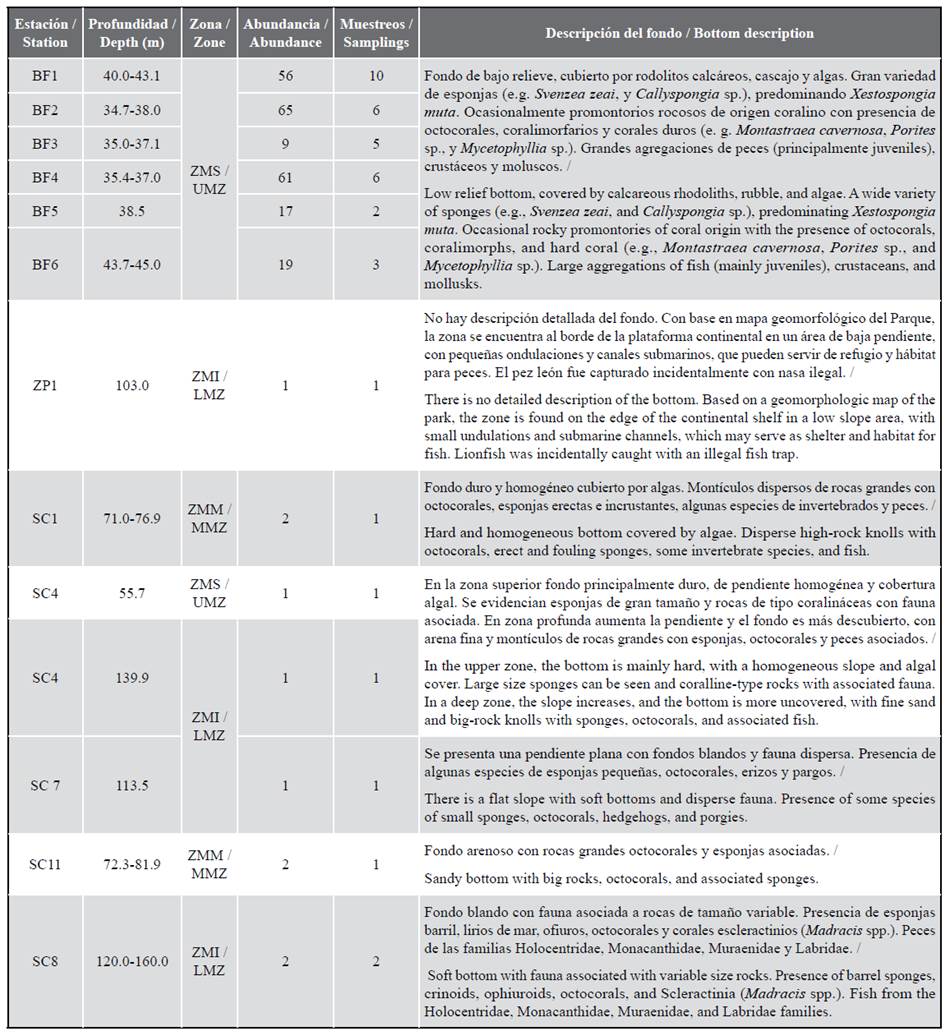
In SC8 a lionfish was observed at 160 m, associated with a soft bottom with rocks, being the best specimen in CPNNP recorded at higher depth. In that station, the presence of Madracis sp. coral was recorded along with a wide variety of echinoderms, corals, and octocorals (Figure 2f).
Morphologic characteristics and size-weight ratio
Seventy-five individuals of P. volitans were captured with a length (¯x ± EE) of 30.5 ± 3.5 cm and a weight of 376.5 ± 43.5 g. The size of the smallest fish was 19.0 cm, the largest was 42.0 cm, and the weight was between 61 and 790 g. Males were significantly larger (Mann-Whitney’s test, U11;39= 396.0; P < 0.001) and heavier than females. The estimated ratios between length and weight were isometric for females (t test, t1 = 1.16; P = 0.25), males (t test, t1 = 0.89; P = 0.57), and combined sexes (t test, t1 = 0.56; P = 0.37) (Figure 3). However, the comparison of the obtained models indicates that growth is different between sexes (Ancova, F3;71= 235.4; P < 0.001).
Food habits
Out of 75 analyzed stomachs, 66 (88 %) showed partially digested food or prey remains and 9 (12 %) were empty. 16 food components were identified, with crustaceans being the category with the higher number of components (S = 10) in the diet. The fish and NIOM remains showed the highest % P and % F (Table 2). According to the results obtained for % N and % P, the most important teleostean families were Acanthuridae and Monacanthidae. Likewise, those two had the highest frequency in the fish group (Table 2). Among the crustaceans, the families with the highest % N and % P were Penaeidae and Palicidae, and Palaemonidae and the suborder Dendrobranchiata showed the highest % F. According to % RII , the Acanthuridae family was the most important, followed by Penaeidae and Monacanthidae. The trophic niche breadth of lionfish in this environment is low (Bi = 0.22).
Carbon stable isotope (δ13C) and nitrogen (δ15N) analyses were conducted on 19 females (30.5 ± 4.4 cm) and 11 males (35.6 ± 3.6 cm). In general, the mean of δ13C was -17.08 ± 0.36‰ (from -17.96 to -16.74‰) and 8.68 ± 0.46‰ for δ15N (from 7.38 to 9.66‰). Males had a carbon enrichment of (-17.06 ± 0.27‰ of δ13C) like the females (-17.15 ± 0.50‰ de δ13C), without significant differences (t-Student, t28 = 1.2 × 10-3; P = 0.17). Likewise, males showed similar nitrogen enrichment (8.85 ± 0.35‰ of δ15N) as females (8.60 ± 0.54‰ of δ15N), with no significant differences (t-Student, t28 = 1.4; P = 0.17).
The ellipse area (SEAc) calculated through a Bayesian model was 0.49‰ in males, and 0.52‰ in females (Figure 4) consuming preys with a narrow interval of δ13C (≈ 1.4‰) and δ15N (≈ 2.3‰). There is a trophic niche overlap between sexes (males vs. females: 74.9 % and females vs. males: 76.8 %) indicating that despite the differences in size, there is no significant differential use of resources or consumption of different species as main preys.
Table 2 Percentage regarding trophic indices of the prey of Pterois volitans. N: number of individuals. % N: percentage by number. % W: percentage by weight. % FO: frequency of occurrence. % RII: index of relative importance. UOM: Unidentified organic matter.
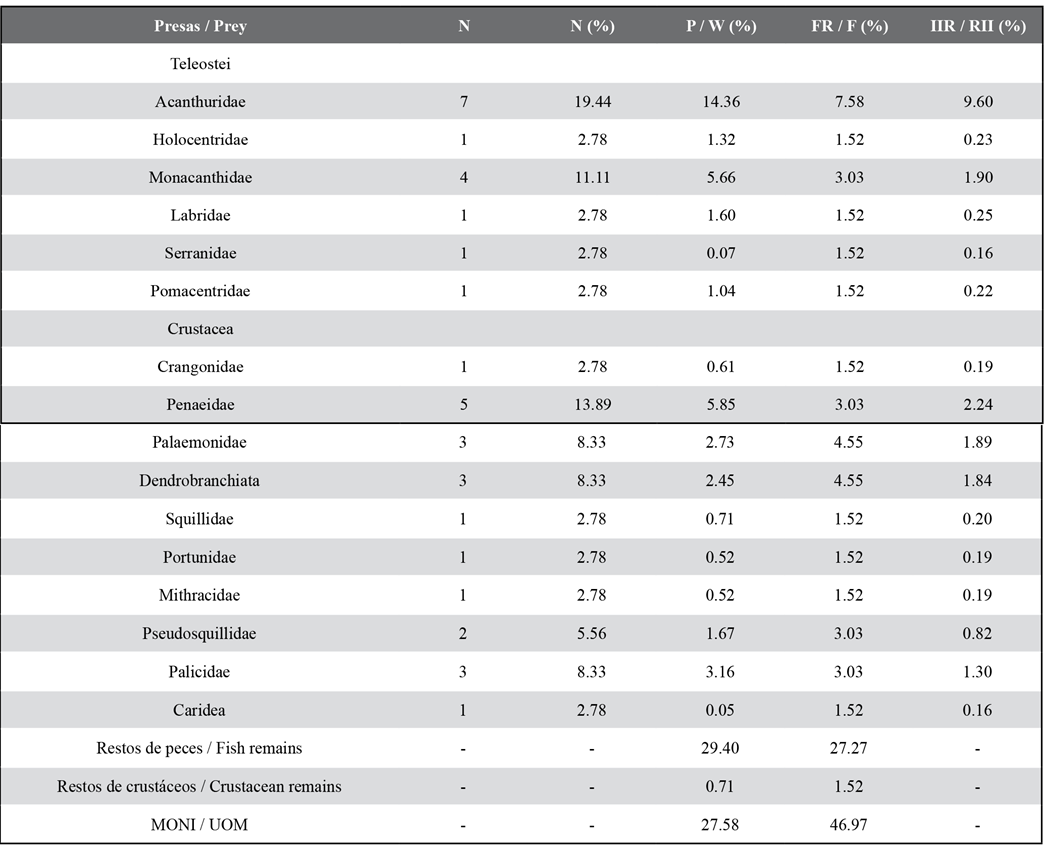
Reproduction
Based on the visual inspection of gonads, 50 out of the 75 fish collected were females and 25 males. Histological preparations of 50 individuals were analyzed, finding that all of them were mature. 78 % were females (19-33 cm, 61-440 g), and 22 % were males (29-42 cm, 338-760 g). In females the regression stage was predominant (n = 15), followed by active spawning (n = 10), spawning (n = 9) and late development (n = 5). Developing fish were classified as late because oocytes in primary growth, cortical alveolus, and vitellogenesis (primary and secondary) were observed. In the spawning stage, tertiary vitellogenated oocytes were evident, as well as post-ovulatory follicles; cases where the migration or rupture of the germinal vesicle was observed were assigned as active spawning (Figure 5). The gonads in regression were characterized by the presence not only of post-ovulatory follicles, but also of atresia. Due to its oocyte asynchronous development, several stages of the oogenesis in the same ovary in active spawning (Figure 5) can be observed. In all cases, the assignations, made macroscopically by visual inspection of the gonads, matched the microscopic ones.
Fish body condition and gonadal cycle
The condition factor (CF) and the gonadosomatic (GSI) and hepatosomatic (HSI) indices are shown in Figure 6. In females, the CF increased with the gonadal development (as the GSI increased) and reached a peak in the active spawning stage, to then decrease in regression. The CF of mature males was comparatively low. Advanced maturity stages (spawning and active spawning) were seen in females between 25 and 33 cm Tl, although an individual of 19 cm Tl was recorded in the regression stage.
DISCUSSION
The presence of lionfish in the three ecological zones of the CPNNP’s MRE (35-160 m depth) coincides with the findings in other sectors of the Caribbean Sea (Andradi-Brown, 2019) and indicates colonization success in mesophotic environments in the Colombian Caribbean. The greater number of sightings in the UMZ is consistent with records in the Western Caribbean (Andradi-Brown, 2019), although the greater technology required and the reduced dive time at greater depths must also be considered.
Therefore, in the park’s MMZ and LMZ, there is a higher difficulty to control the lionfish population through extractive activities, which adds to a lower impact on fishing (Goodbody-Gringley et al., 2019), since hand-line fishing (most used art in mesophotic and deep zones) has a low catch rate for this species. Fish trap, the method that provides greater catch frequency (Merlano-Cera and García-Urueña, 2018), is a forbidden fishing practice inside the CPNNP.
Hence, in the protected area, the implementation of specific catching methods for mesophotic and coral ecosystems is required, which are exclusively directed toward this species (Harris et al., 2020). The increase in the maximum sighting depth in CPNNP to 160 m confirms the fish’s capabilities for invasion in mesophotic environments, with the implications that this might have on their functionality (Barbour et al., 2011; Brightman-Claydon et al., 2012; Agudo y Klein-Salas, 2014), considering that these ecosystems have been deemed of high importance for conservation and protection due to their high ichthyic richness (Pinheiro et al., 2016).
Lionfish abundance in the mesophotic zones is also associated with the habitat and the availability of food resources, as it is evident in other Caribbean zones (Agudo and Klein-Salas, 2014; Nuttall et al., 2014). The CPNNP, being away from the coast (˃32 km) and having a wide depth interval (34-1240 m), offers adequate conditions for the proliferation of lionfish in mesophotic environments. Most sightings occurred in sites with large sponges, coral and octocoral groups, and big rocks; theory states that lionfish density in MRE is apparently affected by habitat complexity, which generates an adequate niche for this species (Andradi-Brown, 2019; Hunt et al., 2019).
In addition, Pterois shares the habitat with many fish species and invertebrates of ecological and economic importance, and it looks for food in places with fish aggregations, mainly of small sizes (Schmahl et al., 2008). This coincides with the observations in the park’s UMZ and other mesophotic and deep zones (Nuttall et al., 2014; Whitfield et al., 2014), thus supporting the idea that Pterois impacts the park’s native fish populations, either by consumption of juvenile fish and small species or by competing with native mesopredator species for preys or shelter (Goodbody-Gringley et al., 2019; Hunt et al., 2019).
The caught specimens had an average size larger than that recorded in shallow reefs of the Colombian Caribbean (Abril-Torres, 2012; Muñoz-Escobar and Gil-Agudelo, 2012; Gómez-Pardo, 2014; Pabón and Acero P., 2016; Romero-Arteaga, 2017; Merlano-Cera and García-Urueña, 2018). They also are larger than those described in most studies carried out in the Western Atlantic (see review in Acero P. et al., 2019), except for what was found by Eddy et al. (2016) in Bermuda (33.5 ± 0.2 cm) and Muñoz et al. (2011) in North Carolina, USA (30.9 ± 0.5 cm). This agrees with the trend described in the invaded area that lionfish tend to be larger at greater depth (Andradi-Brown, 2019; Bustos-Montes et al., 2020), which relates to the following: (a) captures are mainly made in shallow sectors, which decreases the average size and weight over time (Frazer et al., 2012; Henly, 2017); and (b) there could be ontogenetic migrations of shallow ecosystems to deep ones (Barbour et al., 2010; Biggs and Olden, 2011; Brightman-Claydon et al., 2012).
The obtained length-weight ratios do not coincide with the allometric growth recorded earlier for this species (Barbour et al., 2011; Darling et al., 2011; de León et al., 2013; Fogg et al., 2013; Edwards et al., 2014; Sandel et al., 2015; Aguilar-Perera and Quijano-Puerto, 2016; Sabido-Itzá et al., 2016; Villaseñor-Derbez and Fitzgerald, 2019; Chasqui et al., 2020). However, it was confirmed that males reach larger sizes (Edwards et al., 2014). Fogg et al. (2019) state that handling lionfish populations requires making estimations of the local reproductive cycle and growth rates because, in the case of the size-weight ratio, there are significant differences in biomass estimations calculated from the fish lengths when using coefficients different from those of each region in particular. The above highlights the importance of the findings made, pioneering in mesophotic environments in Colombia for handling and control of this invading species in the local ecosystems. In this way, the effectiveness of the associated programs and the impact on the ecosystems could be assessed, and future estimations of this species’ biomass could be made.
Food habits
The high proportion of prey in an advanced state of digestion complicated their identification. This was influenced by the time elapsed between capture and processing of specimens due to logistic restrictions associated with the sampling in a mesophotic environment away from the coast. Therefore, it is necessary to keep analyzing lionfish specimens to know their diet more broadly and accurately in the park. In Bermuda, a big number of stomachs with very digested prey were found, inferring that possibly lionfish prey richness could be much broader than the registered one (Eddy et al., 2016). Besides, the lower richness of preys in the CPNNP may be a consequence of the lower richness of species in mesophotic environments than in shallow ones, as was recorded in Puerto Rico (Bejarano et al., 2014), Curaçao (Pinheiro et al., 2016), and a global review of publications between 1968 and 2010 (Pyle et al., 2019). In addition, lower diversity and biomass have been found in the deeper strata in Bermuda (Stefanoudis et al., 2019a).
The food items found in the stomach analyses have been previously found in the USA, Bahamas, Bermuda, Gulf of Mexico, and the Caribbean, where lionfish’s diet is mainly made up of teleostean and crustaceans (Morris et al., 2009; Muñoz et al., 2011; Dahl and Patterson, 2014; Sandel et al., 2015; Eddy et al., 2016; Acero P. et al., 2019). The most important fish families, Acanthuridae and Monacanthidae, have been also recorded as preys in other studies in the Caribbean, but with lower numerical importance (Muñoz et al., 2011; Pantoja-Echevarría et al., 2017; Acero P. et al., 2019), possibly due to the fact that they were found less frequently in the stomachs. These two families have species that are observed in the CPNNP with occasional to common abundances (Chasqui-Velasco and González-Corredor, 2019). The importance of the Penaeidae family in the lionfish’s diet coincides with findings in other studies (Pantoja-Echevarría et al., 2017; Acero P. et al., 2019). It was found in Cuba that large-size individuals mainly consumed penaeid shrimp (Pantoja-Echevarría et al., 2017).
The calculated amplitude of the trophic niche was against those found in other studies in the Caribbean, where lionfish have been considered a generalist opportunist species able to consume abundant prey, reaching more than 200 species (Acero P. et al., 2019). This ability to adapt to different environments has allowed the population success of the P. volitans, decimating populations of Haemulidae, Labridae, and Pomacentridae native species in some places (Albins and Hixon, 2008; Morris and Akins, 2009; Green et al., 2012).
In the CPNNP, the carbon and nitrogen isotopic ratios in lionfish were lower than those recorded in studies carried out at higher latitudes, both in shallow (Muñoz et al., 2011; Layman and Allgeier, 2012; Arredondo, 2016; Curtis et al., 2017) and deep (Eddy et al., 2020) environments, and also in shallow coral reefs in the Colombian Caribbean (Acero P. et al., 2019). The only study with similar concentrations in both isotopes was carried out in the Bahamas (O’Farrell et al., 2014). The lowest concentrations of isotopes in the Colombian mesophotic environment coincides with recent findings in Bermuda (Eddy et al., 2020). The isotopic differences between shallow and deep zones may reflect changes in the structure of the reef community for different taxa, and their low connectivity (Stefanoudis et al., 2019a, 2019b), and therefore, the prey availability for lionfish may change (Eddy et al., 2020). It is important to highlight that macroalgae and seagrass are not common in deep waters, so the δ13C values in lionfish prey may decrease with depth as the carbon sources become more planktonic and pelagic (Eddy et al., 2020).
The low intervals of δ13C (1.22‰) and δ15N (2.28‰) coincide with the amplitude of the Levin’ niche in that, in CPNNP, Pterois tends to behave like a specialist, which has been observed in some regions of the Great Caribbean such as Cuba and Bahamas, where it was evident in large-size individuals (Green et al., 2012; Rojas-Vélez et al., 2019). In this context, in the CPNNP, there could be food specialization because, in tens of fish species, it has been evident that generalists can show trophic segregation at the level of subgroups of individuals in small niches (Bolnick et al., 2003). If this is so, it should be considered that the specialized groups of a generalist species may affect preys in a similar way to the specialists regarding the decrease and even their local extinction (Araújo et al., 2011). However, the results of this study may be influenced, to a greater or lesser extent, by the low sample size, so further analysis would be required to fully describe this predator’s diet.
Reproduction
The histologically observed oocyte development in P. volitans females in the CPNNP coincides with the description made in the Gulf of Mexico and Cuba (Fogg et al., 2017; Cruz-López et al., 2020). The fact that caught females were sexually mature despite having been collected at different times of the year is a sign of the reproductive potential of the species and its capability to keep reproductively active for a long period or even during the whole year, as has been described in other regions (Gardner et al., 2015; Fogg et al., 2017).
This predominance of mature females in deep environments coincides with findings in Bermuda (Eddy et al., 2019) and suggests that mesophotic environments could be important places for the lifecycle of this species, especially regarding reproduction. The CF pattern of females during gametogenesis suggests an energy transfer (expressed in weight) for the development of ovaries. The GSI increases during oogenesis, reflecting the increase in the relative weight of gonads, while the fat reserves accumulated in the liver move to the ovaries and the HSI decreases quickly (Costa, 2013; Domínguez-Petit et al., 2017). However, in this study, the GSI and HSI behaviors are similar between stages, which goes to show that, in Pterois, the energy mobilization for the oogenesis is fast.
This coincides with the differences in size found between the two sexes because females allocate a greater proportion of the obtained energy to the formation of gonad products (Gadgil et al., 1970) than to the somatic growth. Lionfish mature between the first and the second year of life (Fogg et al., 2017; Bustos-Montes et al., 2020) and has a high mortality rate (Bustos-Montes et al., 2020), so its energetic strategy must be efficient enough to maintain high and constant reproductive rates that compensate the expense reducing their growth with maturing (Fogg et al., 2019), as it has been confirmed in other teleosteans (Reznick, 1983; Roff, 1983). In the case of males, the fact that they reach higher sizes increase their success possibilities in the intersexual competition and their mating opportunities (Shine, 1989; Fogg and Faletti, 2018). The results of this first study on the biology and ecology of lionfish in the CPNNP’s mesophotic environment highlight the need for continuing to make monitoring efforts and observations in the whole depth interval of the park and the implementation of new strategies for controlling and handling lionfish.