INTRODUCTION
Used automobile engine lubricants contain chemical compounds that are potentially toxic to the environment, including polycyclic aromatic hydrocarbons, chlorinated compounds, and heavy metals. Such xenobiotics are produced by incomplete combustion in the engine, wear and tear, and contamination by substances from the fuels (Olonisakin et al., 2005; Cvengros et al., 2017). Generally, after traveling 5 000 to 8 000 km, engine lubricants are removed from the sump and subsequently stored. However, improper disposal can result in the dispersion of organic and inorganic xenobiotics in marine-coastal ecosystems, which can be easily absorbed and incorporated into the biota. Alterations in biochemical and cellular responses caused by water-soluble fractions of used automobile engine lubricants (Wsfual) have been previously evaluated in various model organisms: annelids (Zapata-Vívenes et al., 2018), bivalves (Zapata-Vívenes et al., 2022), and fish (Freitas et al., 2020).
Wsfual toxicity often increases due to the formation of reactive oxygen species (ROS) or other free radicals, which occur during the redox metabolism of their xenobiotics at the cellular level (Zheng et al., 2020). The overproduction of ROS can disrupt cellular antioxidant efficiency, which constitutes a prelude to oxidative changes such as lipid peroxidation, alterations in the structural properties of biological membranes, the disruption of their fluidity, and the reduction of the capacity to maintain ionic balance (He et al., 2017; Basria et al., 2019). Changes in biological membranes may compromise the stability and functionality of lysosomes, which can lead to degradative processes and cell lysis (Trivedi et al., 2020). Lysosomes play a fundamental role in the metabolism and storage of xenobiotics, and the destabilization of their membranes has been used as a marker of cellular stress (Aliko et al., 2015; Parisi et al., 2021).
Biomarkers that determine stress by pollution encompass a range of molecular and cellular responses, which can be sensitively modulated by the presence of contaminants (Zannella et al., 2017). By using biomarkers in representative organisms of the environment, particularly estimated in sessile and filter-feeding species such as bivalve mollusks, it has become feasible to monitor the health status of marine-coastal ecosystems (Krishnakumar et al., 2018). Particularly, immunological biomarkers have been frequently used to estimate the effects of environments impacted by xenobiotic mixtures, and, in bivalves, they offer interesting aspects for application in ecotoxicological studies (Balbi et al., 2021). The immune capacity of bivalves is mediated by hemocytes and bacteriolytic substances that constitute effective first-line defense mechanisms against invasive microorganisms (Zannella et al. 2017) and have been used as warning signals to identify areas impacted by pollution (Renault et al. 2015).
Pinctada imbricata (Röding 1758) is a pearl oyster with relative abundance and broad distribution in the Atlantic Ocean. It has been found to form dense beds in the Caribbean Sea, particularly in Colombia and Venezuela, and generally develops in shallow waters in reef areas and Thalassia testudinum seagrass beds (Vásquez et al., 2015). This species holds dual economic importance, as its meat is utilized as a protein source and its shell is valued for nacre production. The potential use of P. imbricata in mariculture has been recognized (Lodeiros et al., 2016; Romero-Ferreira et al., 2017), and it has been proposed as a biological model in ecotoxicological tests (Nusetti et al., 2004; Villegas et al., 2015). In this vein, this research evaluated the short-term effects of sublethal concentrations of Wsfual on the immune-innate responses (cellular and humoral) and the stability of lysosomal membranes in hemocytes of the bivalve P. imbricata. Concurrently, in the digestive gland, the levels of malondialdehyde and lysozyme activity were determined as markers of oxidative damage and humoral defense, respectively. The concentrations selected for the exposure bioassay represent possible contamination scenarios caused by Wsfual spills in marine-coastal ecosystems.
MATERIALS AND METHODS
Organisms. Adult specimens of the pearl oyster P. imbricata, without sex distinction and an anteroposterior length of 50-60 mm, were manually collected by free diving in reef areas located in the southern region of the Cariaco Gulf (10°26’56’’ N, 64°02’00’’ W), Venezuela, during the coastal upwelling season. The oysters were transported in isothermal containers to the laboratory and then maintained in 40 L capacity aquariums with filtered seawater (10 µm) at a temperature of 25 ± 1º C, a salinity of 36, and a pH of 7.8 ± 0.2. They were oxygenated (6.2 mg/L) for one week as an acclimatization period. These physicochemical variables were similar to those found in their environment at the time of collection. The oysters were fed daily ad libitum with an isomix of the cultured microalgae (Chaetoceros araya and Tetraselmis chuii).
Water-soluble fraction of lubricants. The source of contaminants consisted of a mix of used vehicle engine lubricants obtained from a local service station (gas station) and filtered seawater (45 µm), at a 1:9 v/v ratio. The mix was kept under constant agitation for a period of 24 hours at room temperature, with one hour of settling time. To eliminate the remnants of the non-soluble fraction, the mixture was separated by siphoning and subsequently filtered under a vacuum system through a Whatman #1 paper. The collected Wsfual was used as a stock solution (100 % v/v) to perform dilutions (Zapata-Vívenes et al., 2020).
Exposure bioassays. 20 organisms per aquarium (in triplicate) were exposed to ascending concentrations of 1, 10, and 20 % v/v of Wsfual for various periods: 3, 5, and 7 days. The sublethal concentrations were selected based on a previously estimated median lethal concentration (LC50= 83.10 %; 96 h). A group of organisms, which served as a control, were placed alone in seawater and without contact with contaminants. The quality and physicochemical parameters of the seawater were maintained similarly to the acclimation period. Three quarters of the seawater were changed daily, in order to maintain the available concentrations of the contaminant mix and remove pseudo-feces and excretion products from the organisms. To measure the cytological and immunological parameters after each exposure period, hemolymph was extracted from each oyster. Additionally, the digestive gland was dissected to quantify lysozyme activity and the levels of peroxidized lipids and total proteins.
Hemolymph extraction. Using a 1 mL capacity hypodermic syringe preloaded with 0.2 mL of seawater-EDTA at 0.01 mmol/L, a direct puncture was made into the venous sinus located near the phasic portion of the adductor muscle, aided by a mechanical separation of the valves. 0.5-1 mL of hemolymph was extracted from each specimen. The hemolymph was poured into microcentrifuge tubes placed on ice (4 °C). The sample was washed and resuspended in 1 mL of filtered seawater-EDTA 0.01 mmol/L at pH 7.8. Finally, it was preserved at 8 °C for subsequent analysis.
Cell viability and total hemocyte count. A mixture of 20 µL of the final hemocyte suspension was combined with an equal volume of 0.4 % trypan blue solution (Sigma) (Strober, 2015). Cell viability and the total number of hemocytes (TNH) per mL were determined via light microscopy in a Neubauer chamber at a magnification of 400X. The THC per mL was determined using the average of four quadrants (X) of the chamber and was calculated using the following formula: THC/mL = (X/4) × dilution factor × 10,000 (Zapata-Vívenes et al., 2022).
Phagocytosis. A hemolymph sample adjusted to a concentration of 2 x 106 cells/mL was used in the presence of heat-inactivated Sacharomyces cereviceaea yeast (3 mg/mL) as an antigen source. In a microcentrifuge tube, 100 µL of the hemocyte suspension were added, along with an equal volume of yeast, and centrifuged at 1 000 x g for 5 min. The samples were incubated for a period of 24 h at 8 °C. To the cell precipitate, 100 µL of 0,4% trypan blue were added and resuspended with a Pasteur pipette. Finally, 10 µL were placed in a Neubauer chamber for counting under a light microscope at a magnification of 400X. A total of 100-200 viable cells were counted for each sample, and hemocytes containing at least one visible yeast in their cytoplasm were considered to be phagocytes (Nusetti et al. 2004). This process was repeated at least three times.
Lysosomal membrane stability. Lysosomal membrane stability was parameterized by counting damaged cells (Lowe et al., 1992). A total of 40 µL of neutral red stock solution (4 mg/mL dissolved in dimethyl sulfoxide) were added to an equal volume of hemolymph. Mixing and incubation were performed in a moist and dark chamber for 15 min. The percentage of hemocytes with lysosomal membrane destabilization (LMD; %) was estimated for 240 min (Méthé et al., 2017).
Lysozyme. The protocol used to assess lysozyme activity in the digestive gland of P. imbricata was based on the lysis of the cell wall of Micrococcus lysodeikticus, using agarose lyso-plates. The diameters of the lysis inhibition halos were compared to the standard of hen egg-white lysozymes (HEL) through linear regression analysis (Goven and Kennedy, 1996).
Lipid peroxidation and total proteins. The malondialdehyde (MDA) level in the digestive gland was determined spectrophotometrically at 535 nm through a thiobarbituric acid reactive substances assay, using 1,1,3,3-tetraethoxypropane as a standard (Ohkawa et al. 1979). The values were normalized to the total protein content. Protein quantification was carried out following the methodology proposed by Lowry et al. (1951).
Statistical analysis. The effects on immunological and biochemical parameters were numerically analyzed via a two-way ANOVA (concentrations and days of exposure) with a Dunnet post hoc test (Sokal and Rohlf, 2012). Prior to analysis, the data were tested for normal distribution and variance homogeneity. All data were analyzed using the Statgraphics Plus version 5.1 software.
RESULTS
Cell count and viability. An increase in the TNH) was observed in oysters exposed to 1 % Wsfual over three days of exposure (F = 4.32; p < 0.001), in contrast to control oysters for this period (Figure 1). During days 5 and 7, the oysters exposed to Wsfual showed a tendency towards a decrease in the TNH at all exposure concentrations (F = 15.80; p < 0.001), evidencing the greatest effect at the 20 % concentration.
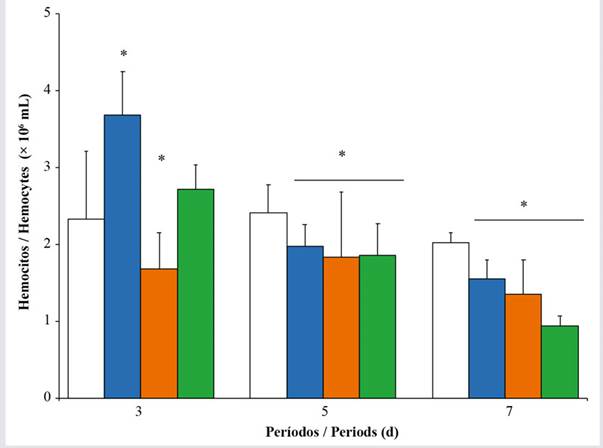
Figure 1 Total hemocyte count of Pinctada imbricata exposed to concentrations i.e., 0 % (), 1 % (), 10 % (), and 20 % () of a water-soluble fraction of used automobile engine lubricants (Wsfual) over different periods. The bars and trend lines represent the means and standard deviation, respectively. (*) indicates a difference of p < 0.05 with respect to the control (0 %).
The viability of hemocytes in oysters exposed to Wsfual and those unexposed varied significantly, both at different concentrations and during the exposure periods (F = 25.86, p < 0.001 and F = 4.28, p < 0.001, respectively) (Figure 2). During day 3 of exposure, there was no variation in the oysters’ hemocyte viability when contrasted with the controls. However, after the fifth day of exposure, a clear reduction in this parameter was observed at all exposure concentrations. After seven days, the oysters exposed to the highest concentration of Wsfual showed a reduction in the percentage of viable cells (~ 66 %).
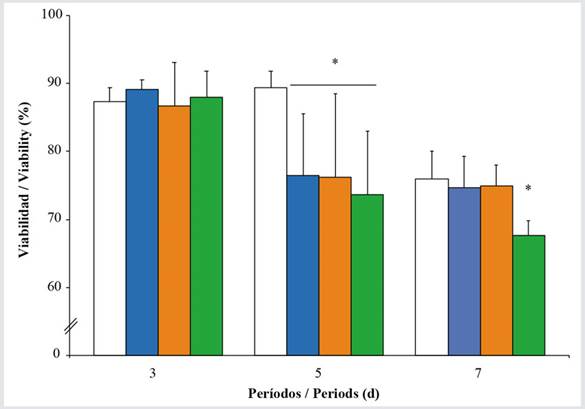
Figure 2 Hemocyte viability in Pinctada imbricata exposed to various concentrations i.e., 0 % (), 1 % (), 10 % (), and 20 % () of a water-soluble fraction of used automobile engine lubricants (Wsfual) over different periods. The bars and trend lines represent the means and standard deviation, respectively. (*) indicates a difference of p < 0.05 with respect to the control (0 %).
Phagocytosis and lysozyme. The number of phagocytes (NP) showed a significant increase during the first period of exposure, especially at concentrations of 10 and 20 %. However, during the seventh day, there was a decrease in this response, with the most pronounced effect taking place on oysters exposed to higher concentrations of Wsfual (Figure 3). Lysozyme activity in the digestive gland showed highly significant differences between concentrations (F = 4.83; p < 0.001) among the organisms subjected to Wsfual, but it did not show a similar trend over the days of exposure (F = 0.60; p > 0.05). A noticeable decrease in lysozyme activity was observed at the highest concentration (20 %) during the first three days of exposure (Figure 4). Conversely, an increase in the activity of this bacteriolytic enzyme was observed over the seven days of exposure in oysters exposed to the 10% concentration of Wsfual (F = 14.22; p < 0.001).
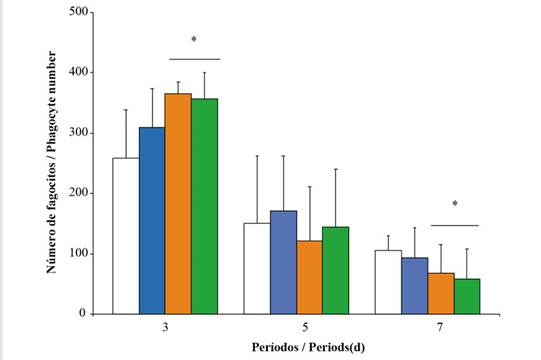
Figure 3 Number of phagocytes in the hemolymph of Pinctada imbricata exposed to various concentrations i.e., 0 % (), 1 % (), 10 % (), and 20 % () of a water-soluble fraction of used automobile engine lubricants (Wsfual) over different periods. The bars and trend lines represent the means and the standard deviation, respectively. (*) indicates a difference of p < 0.05 with respect to the control (0 %).
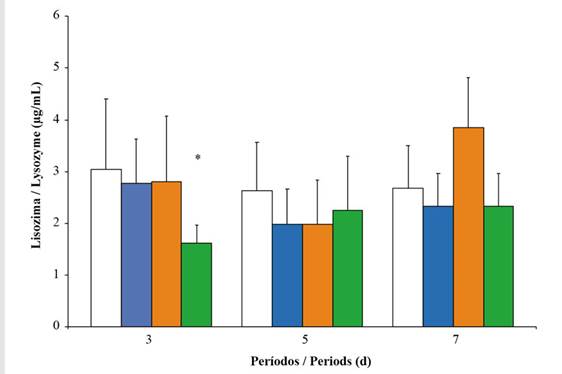
Figure 4 Lysozyme activity (µg/mL) in the digestive gland of Pinctada imbricata exposed to concentrations i.e., 0 % (), 1 % (), 10 % (), and 20 % () of a water-soluble fraction of used automobile engine lubricants (Wsfual) over different periods. The bars and trend lines represent the means and the standard deviation, respectively. (*) indicates a difference of p < 0.05 with respect to the control (0 %).
Lysosomal stability. Oysters exposed to increasing concentrations of Wsfual exhibited a population of hemocytes with a reduced capacity for retaining neutral red over 240 min. This parameter showed highly significant differences between the oysters exposed to the experimental concentrations (F = 22.54; p < 0.001) and across the three periods of exposure to Wsfual (F = 17.17; p < 0.001), with an observed increase in lysosomal damage concomitantly associated with exposure concentrations. The highest percentage of damaged cells was evidenced in oysters exposed to 20 % for seven days (Figure 5).
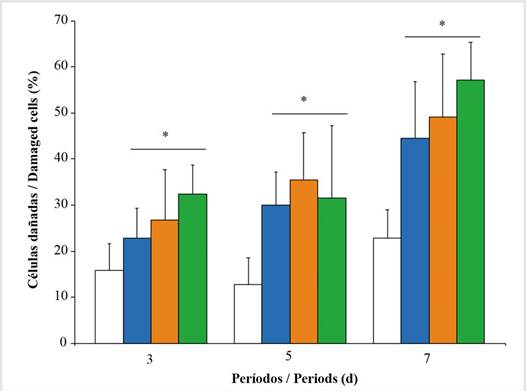
Figure 5 Percentage of cells with membrane damage in Pinctada imbricata (during 240 minutes of incubation) exposed to i.e., 0 % (), 1 % (), 10 % (), and 20 % () of a water-soluble fraction of used automobile engine lubricants (Wsfual) over different periods. The bars and trend lines represent the means and the standard deviation, respectively. (*) indicates a difference of p<0.05 with respect to the control (0 %).
Lipid peroxidation. The levels of MDA in the digestive gland of oysters exposed during the three periods showed similar contents (F = 1.90; p > 0.05). However, highly significant differences were observed between the experimental concentrations (F = 6.17; p < 0.05). A slight increase in the MDA concentrations was evidenced in oysters exposed to the highest concentration during three and five days of exposure (Figure 6).
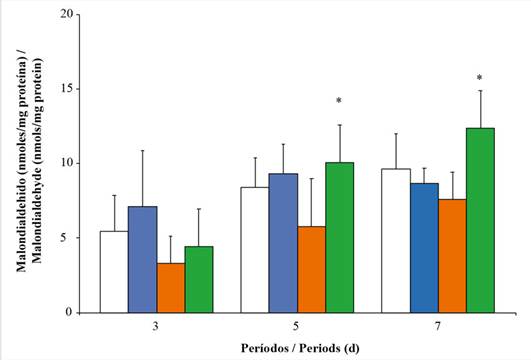
Figure 6 Levels of malondialdehyde (MDA) in the digestive gland of Pinctada imbricata exposed to i.e., 0 % (), 1 % (), 10 % (), and 20 % () of a water-soluble fraction of used automobile engine lubricants (Wsfual) over different periods. The bars and trend lines represent the means and the standard deviation, respectively. (*) indicates a difference of p < 0.05 with respect to the control (0 %).
DISCUSSION
This study shows evidence of alterations in the hematological and innate immunological responses of the oyster P. imbricata, particularly in defenses mediated by hemocytes and the activation of humoral protection mechanisms in the presence of sublethal concentrations of Wsfual. These indications are based on the increase in TNH and NP in oysters contaminated with Wsfual during the earliest period of exposure. It appears that the increase in the hemocyte population in exposed oysters is part of the unspecific responses to chemical stress. There is evidence to suggest that immune functions in bivalves can be modulated in the presence of xenobiotic mixtures, which is closely related to exposure concentrations (Burgos-Aceves and Faggio, 2017; Zapata-Vívenes et al., 2022). In contrast, at low concentrations of Wsfual, there was a correspondence between TNH, PV, and the increase in NP, which could be linked to possible hematopoietic stimulation of oysters by the direct action of xenobiotics, similar to what often unspecifically occurs facing the invasion of opportunistic microorganisms (Allam and Raftos, 2015; Bachère et al., 2015). Similarly, an increase in cell damage caused by oxyradicals on hemocyte viability and immune defenses, such as the production of superoxide anions, TNH, and phagocytic activity, has been observed in the mussel Mytilus edulis collected in areas impacted by oil spills (Auguste et al., 2020; Balbi et al., 2021), as well as in Tegillarca granosa, which is associated with the intake of microplastics (Sun et al., 2020). It is considered that the production of hemocytes and the increase in phagocytic activity are energetically costly processes, and that, in the presence of complex mixtures of contaminants, they can be affected by the diversion of energy towards physiological processes of molecular defense, through redox metabolism, against the newly incorporated xenobiotics (López-Landavery et al., 2022).
In the five- and seven-day periods, TNH and PF showed a significant decrease, thus allowing to estimate the time and minimum concentration necessary for the Wsfual to cause a detrimental effect on cellular responses. The suppressive effects resulting from exposure to contaminants have been a notable response in bivalves and other organisms from different phyla, especially when defense mechanisms against xenobiotics are overwhelmed due to accumulation, metabolism, the overproduction of free radicals, or the formation of substances with higher levels of toxicity in comparison with the original molecule (Balbi et al., 2021; López-Landavery et al., 2022). The reduction in cellular-type responses during prolonged exposure is a physiological signal of their limited performance and sensitivity in chemically stressed P. imbricata oysters. Some predominant functions of hemocytes, such as phagocytosis, digestion, metabolite transport, nutrient storage, wound healing and shell repair, mineralization and excretion, chemotaxis, pathogen recognition, and antimicrobial synthesis might be altered by the presence of toxic mixtures (Song et al., 2010). Immunological responses can vary depending on the type of contaminant. For example, in impacted environments, it has been recorded that some responses such as phenol oxidase activity are inhibited in clams, whereas lysozyme and esterase activities, along with TNH, are increased in individuals collected from highly polluted sites (Mansour et al., 2020), which leads to an increased risk of microbial infection due to the suppression of the phagocytic activity of hemocytes.
Lysozyme activity in the digestive gland was modulated at different exposure concentrations. Initially, a slight decrease in activity was recorded during the first three days of exposure at 20 %. On the contrary, during the seven days of exposure, the oysters maintained at 10 % Wsfual showed an increase in the activity of this enzyme. The action of lysozyme demonstrates a reciprocal effect in relation to immunological defenses mediated by hemocytes, and the increase in lysozyme activity in the digestive gland of P. imbricata exposed in the short term to Wsfual indicates that this response is part of the humoral homeostasis mechanism, which increases biochemical defenses against a chemical stress situation. This protection mechanism has been described in other marine invertebrates, particularly when some immune-cellular parameters are suppressed in invertebrates (Zapata-Vivenes et al., 2022). Lysozyme is an antimicrobial enzyme that acts on the bonds of peptidoglycans in the walls of certain bacteria and fungi, as an innate component that protects the organism from environmental bacteria and controls the natural symbiotic flora (Wei et al., 2017; Liao et al., 2020). Other studies, using P. imbricata as a model, basically showed that the phagocytic capacity of hemocytes and the microbial lytic activity of lysozyme were not adversely altered during the process of sublethal contamination by aqueous fractions of a heavy oil formulation (Nusetti et al., 2004). Likely, the stress conditions manifested in the organism during acute exposure to Wsfual did not have major implications in the capacity of immunological responses associated with the phagocytic potential of hemocytes and the bacteriolytic activity of lysozyme, even though the organism developed a state of oxidative stress that, in certain cases, corresponds with immunotoxic effects.
The oysters exposed to Wsfual exhibited populations of hemocytes with elevated percentages of lysosomal destabilization, showing a parallel relationship between the doses used and the response obtained. The highest percentage of damaged cells was observed in oysters exposed to the highest concentration over seven days of exposure - this damage could influence the reduction of cell viability. Similarly, Hwang et al. (2014) recorded 50 % destabilized cells in the presence of total polycyclic aromatic hydrocarbons in the oyster Crassostrea virginica, directly affecting immunological functions. The dysfunction of lysosomal processes has been linked to many aspects of pathologies associated with chemical toxicity and degenerative processes (Martínez-Gómez et al., 2008). It is known that, in bivalves, lysosomes are the organelles with the greatest capacity for accumulation and homeostasis of xenobiotics, including heavy metals (Zha et al., 2019) and hydrocarbons (Xie et al., 2017). The modulation of the immune system by contaminant agents has been described in a variety of aquatic invertebrates, including bivalve mollusks. Generally, chronic exposure to organic substances (aromatic hydrocarbons, pesticides, polychlorinated biphenyls, among others) has negative effects on the phagocytic activity of hemocytes (Fournier et al., 2000; Jian et al., 2017), this trend of immunological dysfunction is not a constant under acute conditions.
The levels of MDA in oysters exposed to Wsfual during the last period demonstrate oxidative damage to lipids caused by the metabolism of xenobiotics. Similarly, Nusetti et al. (2004) recorded that exposure to aqueous fractions of Fuel Oil No. 6 can cause an increase in the activities of antioxidant enzymes and xenobiotic-metabolizing enzymes in the digestive gland of P. imbricata, as a result of the exacerbated production of free radicals. Lipid peroxidation can disturb the structural and functional properties of the membranes of cytoplasmic organelles. In this context, alterations in biological membranes can compromise the functionality of lysosomal components, entailing degradative processes that can lead to cellular autolysis (Domouhtsidou et al., 2004).
CONCLUSIONS
The chemical components present in Wsfual alter the normal functioning of the immune defense system of the oyster P. imbricata, which is based on modulatory effects on innate immunity and the disruption of the stability of lysosomes in their hemocytes. Furthermore, exposure to Wsfual leads to oxidative damage in the digestive gland, potentially disturbing its immunological-humoral functions. The molecular and immune-cellular responses evaluated prove to be ideal biomarkers for assessing alterations caused by contaminants, which can be implemented as routine analyses in environmental monitoring and aquaculture systems, using P. imbricata as a model organism.