INTRODUCTION
Ecuador is a country with a vast shrimp production (López, 2016), which represents 90 % of its aquaculture sector and is one of the main economic supports of the country (Gonzabay et al., 2021). The whiteleg shrimp Litopenaeus vannamei is the main crustacean cultivated around the world and is of great importance for food security. The development of this crustacean during cultivation depends on factors such as water and soil quality, physicochemical variables, and feeding (Gonzabay et al., 2021). The latter is one of the most relevant economic aspects, exceeding 50 % of the total production costs (Castro and Ordinola, 2021).
In the soil of shrimp ponds, nitrogen and phosphorous contributions - which mainly enter through unconsumed feed and feces - is degraded in the sediment and incorporated to the water column, affecting the entire ecological system, as this forms anaerobic substrates (Zhu et al., 2016; Juárez et al., 2021). Given its negative impact on the environment, ways to transform aquaculture into a sustainable activity must be sought. To this effect, the processes involved should be improved by means of technology, in order to reduce environmental and production costs, especially with regard to feed (Guevara et al., 2022). One of these strategies has been the implementation of automatic feeders, which allows reducing the loss of feed concentrating in the sediment, as well as improving the feed conversion rate and reducing costs (FAO, 2020).
However, the use of automatic feeders can have negative impacts on soil quality (Beltrán, 2017), as there is a risk that the balanced feed is not completely consumed at the feeding points, which entails specific areas of organic matter accumulation, thereby deteriorating soil quality (Rojas et al., 2017; Gilbert, 2021). Therefore, the objective of this study was to evaluate the impact of automatic feeding on the soil quality of a Litopenaeus vannamei culture over one cultivation cycle.
MATERIALS AND METHODS
The study area corresponded to a shrimp farm located in km 18 of the road to the coast (Vía a la Costa) in the Chongón sector (607400.00 m E and 9756200.00 m S), near the city of Guayaquil, Guayas province (Figure 1).
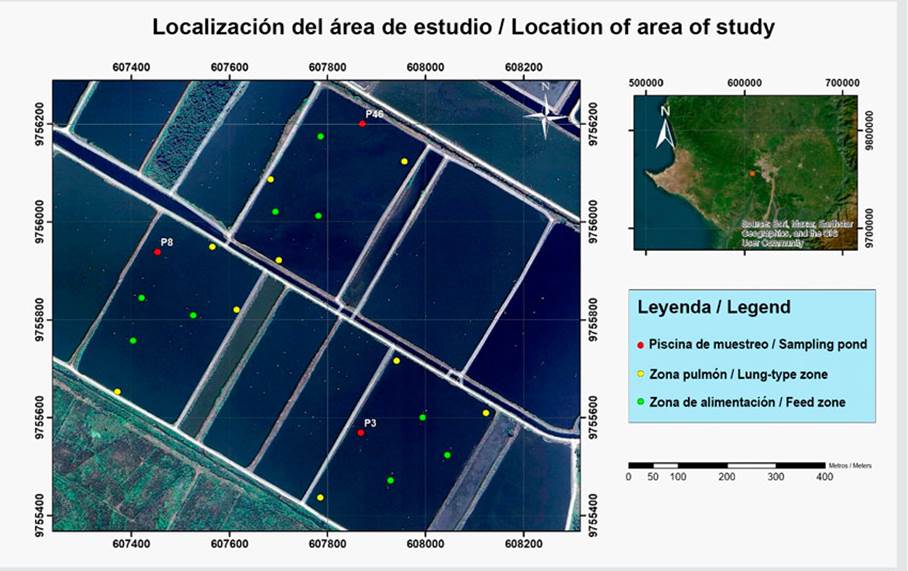
Figure 1 Map of the location of the study area. Red dots show the sampling ponds. Yellow and green dots indicate the samples taken in the lung-type and feeding zones, respectively.
The shrimp farm spans a total of 550 ha. The ponds used during testing were P3 (18.7 ha), P8 (17 ha), and P46 (17.5 ha). The cultivation period went between March and June 2023, which corresponded to a rainy period. The P3, P8, and P46 ponds had a stocking density of 155000 animals/ha; a final harvest of 95000, 93496, and 101000 animals/ha; and a survival rate of 62, 60, and 65 %, respectively. Automatic feeding was of the sonic type, using Eruvaka feeders with a hopper capacity of 125 kg and a dispersion radius of 13 ± 2 m. The feeding frequency was multi-ratio to the satiety of the individuals.
Sediment samples were obtained during stocking and harvesting in three ponds over a cultivation cycle in two zones. The first zone was located near an automatic feeder (feeding zone), and the second one was outside the automatic feeders’ dispersion radius (lung-type zone). 1 kg of sediment were directly taken and stored in airtight polyethylene bags. Sample extraction was carried out at points near automatic feeders (n = 3) and in the lung-type zone (n = 3). In the sediment samples, the following parameters were analyzed: pH (Skoog et al.,1992), total nitrogen (Kjeldahlm, 1883), organic matter (Davies, 1974), and organic carbon according to the NMX-AA-021 method (1985).
The results were analyzed using the Statistica (V.7) software. A one-way analysis of variance (Anova) was performed to identify significant differences in pre- and post-cultivation sampling for the feeding and lung-type zones regarding the following variables: organic matter, organic carbon, pH, total nitrogen, and C/N. An a posteriori Tukey test was carried out in order to identify differences between groups. Variance homogeneity was validated via the Levene test, considering a statistical significance of p < 0.05.
RESULTS
The organic carbon percentage showed no statistical differences between feeding and lung-type areas both before and after L. vannamei cultivation (Figure 2; p ≥ 0.05). This percentage increased by 0.3 % in the lung-type zone after cultivation ended, but, in the feeding area, there was a 0.04 % decrease, albeit showing no significant differences (Figure 2; p ≥ 0.05). As for the organic matter percentage, there were no differences between the lung-type and feeding zones in both cultivation periods (Figure 3; p ≥ 0.05). In the lung-type zone, this percentage increased from 3.1 to 3.2, and, in the feeding area, it decreased from 3.4 to 3.1, albeit with no significant differences (p ≥ 0.05; Figure 3). Regarding the pH, no significant differences were observed before and after cultivation in the lung-type and feeding zones (p ≥ 0.05; Figure 4). However, when cultivation ended, the pH at the lung-type point increased by 0.41, as well as by 0.20 in the feeding area, albeit with no significant differences (p ≥ 0.05).
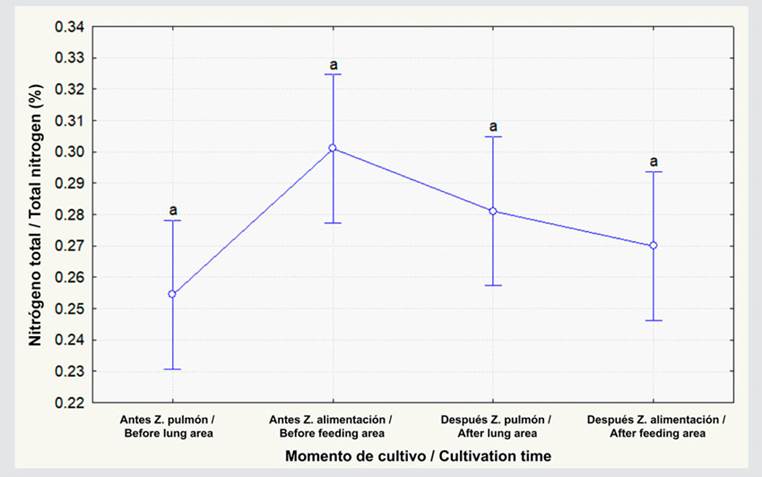
Figure 2 Organic carbon percentage before and after L. vannamei cultivation in lung-type and feeding zones. The data represent the average ± standard error (n = 3). Different letters indicate significant differences (Anova, Tukey test, p < 0.05).
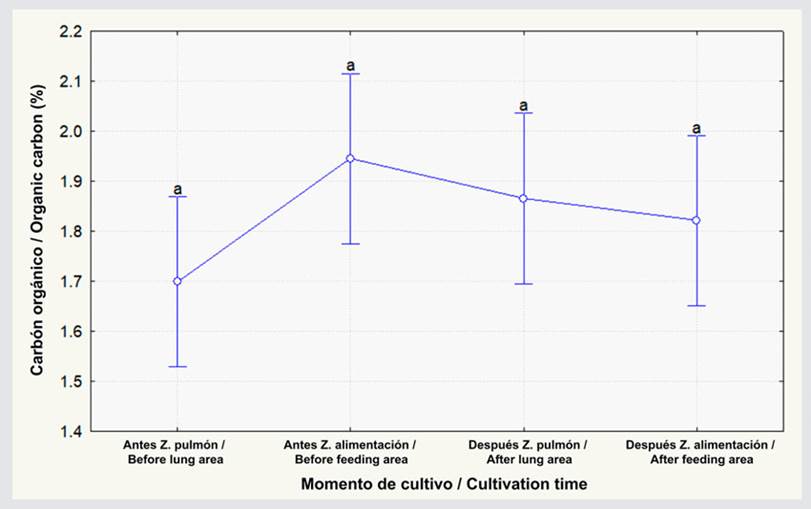
Figure 3 Organic matter percentage before and after L. vannamei cultivation for the lung-type and feeding areas. The data represent the average ± standard error (n = 3). Different letters indicate significant differences (Anova, Tukey test, p < 0.05).
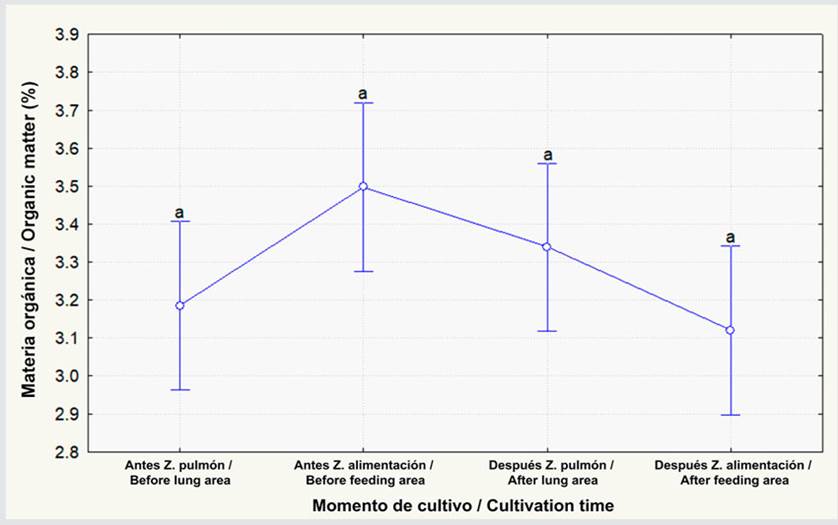
Figure 4 pH levels in the three ponds before and after L. vannamei cultivation for the lung-type and feeding zones. The data represent the average ± standard error (n = 9). Different letters indicate significant differences (Anova, Tukey test, p < 0.05).
The total nitrogen percentage also showed no differences between the lung-type and feeding areas (Figure 5; p ≥ 0.05). Figure 6 indicates that there were no significant differences regarding the C/N ratio between lung-type and feeding areas before and after L. vannamei cultivation (p ≥ 0.05). The concentration levels in the lung-type area were similar (i.e., 6.6 and 6.7), whereas, in the feeding zone, they increased from 6.4 to 6.9, albeit with no significant differences (p ≥ 0.05).
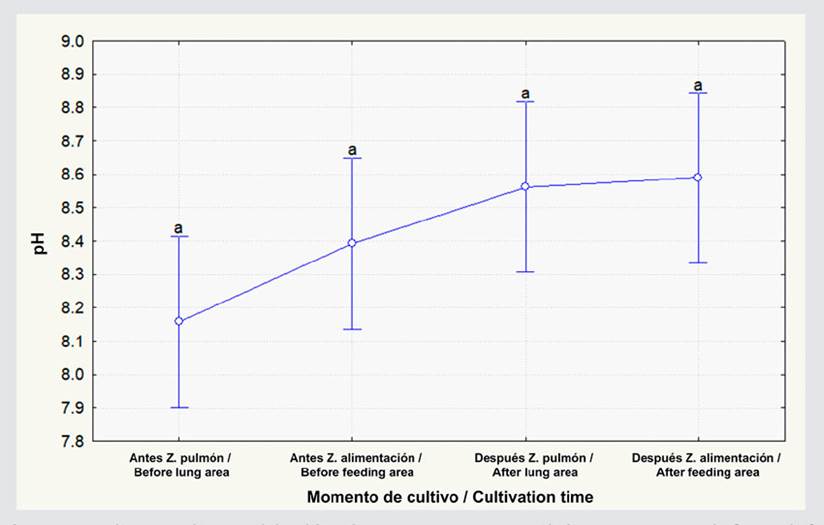
Figure 5 Total nitrogen percentage before and after L. vannamei cultivation at the lung-type and feeding points. The data represent the average ± standard error (n = 3). Different letters indicate significant differences (Anova, Tukey test, p < 0.05).
DISCUSSION
This research could determine that the organic matter percentage fluctuated between 3.12 and 3.49 %. These values are different from those obtained by Jewel et al. (2021), who observed an organic carbon concentration of 2.39 ± 0.06 in whiteleg shrimp cultivation. This difference can be attributed to the daily water exchanges (2.5 m) that were carried out in the aforementioned study, in contrast to this study’s 2 m per day, which reduced the amount of organic matter that could be sedimented. On the other hand, Islam et al. (2016) found values that fluctuated between 4.81 and 6.59 %. These high values might be due to the fact that the cultivation period lasted 12 months, which is higher than that of this study (three months), thus affecting the organic matter’s sedimentation capacity.
As for the organic carbon percentage, the values were between 1.69 and 1.94 %, differing from those obtained by Mustafa et al. (2018) , who recorded percentages between 3.19 and 5.22. The high organic carbon levels reported in the aforementioned research may be associated with the duration of the cultivation period (one year), which increased the sedimentation capacity. On the other hand, Anna and Dinesh (2021) obtained low organic carbon values (1.28 %) without the use of automatic feeders. Nevertheless, the results of this study show similar values, likely indicating that, over three months of cultivation and with a stocking density of 15 animals/m2, no significant organic matter accumulation is observed.
The results of the pH analysis agree with the values obtained in the study conducted by Vinothkumar et al. (2018) , who reported an increase in this variable over the two cultivation stages, with values corresponding to 7.8 and 8.3 before and after harvesting. In this project, the pH fluctuated between 8.15 and 8.59, whereas Islam et al. (2016) obtained values between 6.22 and 8.22 throughout the cultivation period. This fluctuation is explained by the fact that the ponds were in a floodable area due to the effect of the tides. In this work, the ponds were not located in floodable areas; the water level was controlled via daily water exchanges of 2 m. This could have influenced the fact that the pH variations were not wide, since there was constant renewal.
The total nitrogen percentage observed was lower than that found by Vinothkumar et al. (2018) in the pre-cultivation phase, these authors obtained 0.63 %, more than two times the values obtained in this research. However, after cultivation, they recorded a total nitrogen percentage of 0.35 %, a value close to that of this project (0.30 %); this can be attributed to the fact that, in the study by Vinothkumar et al. (2018), nitrogen-rich to promote algae growth before cultivation started, this caused an increase in the initial nitrogen percentage. During the time that elapsed until harvest, nitrogen had to be assimilated by microorganisms, resulting in values between 0.05 and 0.4 %; according to Mustafa et al. (2018) , the aforementioned concentrations can be found in the sediment after completing and starting an L. vannamei cultivation cycle in a pond.
On the other hand, a study conducted by Poersch et al. (2020) obtained total nitrogen concentrations lower than 0.07 % in ponds; these concentrations could be influenced by the amount of organic carbon and the sediment’s high degree of porosity. In addition, said authors determined that constant artificial aeration, coupled with the predominantly sandy contents of the sediment, could have enhanced the decomposition of organic matter, preventing the accumulation of carbon in the sediment. In this research, the amount of organic carbon fluctuated between 1.69 and 1.94, which, together with a less porous soil structure, could explain the total nitrogen content’s fluctuation between 0.25 and 0.30, given that, according to Pelletier et al. (2011) , a greater porosity enhances the nitrogen volatilization process.
As for studies on the C/N ratio in pond sediments, usable information for use in comparisons with this study is scarce; most of the works that have conducted C/N analyses are related to biofloc systems. Some authors like Xu et al. (2022) have performed experiments with different concentrations of C/N, obtaining a better understanding of assimilation of nitrogenated compounds (NO3-N, TN, TAN) when increasing C/N ratios from 8:1 to 16:1; in addition, other authors such as Xu et al. (2016) and Panigrahi et al. (2018) experimented with the effect of different C/N ratios to reduce the concentrations of nitrates (NO3-N), nitrites (NO2-N), total nitrogen (TN), and total ammonia nitrogen (TAN), reporting better reduction rates when increasing the concentrations in the ratio, with the most favorable being the 20:1 C/N ratio.
The relationships presented in this study agree with Boyd (1995) , who found ratios between 6:1 and 12:1 in pond souls, albeit lower than those reported by Boyd et al. (2001). These authors suggest that ratios higher than 10:1 in sediments are adequate for preventing an accelerated decomposition of organic matter and the occurrence of parasitic nematodes, even though they provide a low nitrogenated compounds reduction rate when compared against a biofloc system (Xu et al., 2016, 2022; Panigrahi et al., 2018). Based on that observed in previous research, it is inferred that the C/N ratio of 6:1 found in this study, according to Boyd (1995), demonstrates that the experimental ponds are within the optimal rage for an adequate mineralization of organic matter.
CONCLUSIONS
Automatic feeding does not cause changes in soil quality before and after the cultivation of L. vannamei in both feeding and lung-type areas. No significant differences were observed in the organic carbon percentage, organic matter, pH, and total nitrogen, leading the carbon-nitrogen ratio to show no variations related to automatic feeders, reporting adequate levels for the mineralization of organic matter